Abstract
Purpose
Recent findings of increased cathelicidin protein and its proteolytic fragments in rosacea suggest a pathogenic role for cathelicidin in this disease. The relationship between cathelicidin and protease-activated receptor 2 (PAR-2) is therefore of interest, as PAR-2, expressed principally in keratinocytes, regulates pro-inflammatory cytokine expression in the skin. The purpose of this study was to determine the relationship between expression of PAR-2 and cathelicidin in rosacea and to test the effect of direct PAR-2 activation on cathelicidin expression in keratinocytes.
Materials and Methods
Samples from 40 patients with clinicopathologic diagnosis of rosacea and facial skin tissue samples from 20 patients with no specific findings or milium without inflammation were retrieved. Intensities of immunohistochemical staining for PAR-2 and cathelicidin were compared between normal and rosacea-affected skin tissues. Additionally, correlations between PAR-2 and cathelicidin staining intensities within rosacea patients were analyzed. In cultured keratinocytes, changes in PAR-2, cathelicidin, and vascular endothelial growth factor (VEGF) mRNA and protein were analyzed after treatment with PAR-2 activating peptide (AP).
Results
Cathelicidin expression was significantly higher in rosacea skin tissues than in normal tissues (p<0.001), while PAR-2 expression was not significantly higher in rosacea tissues than in normal skin tissues. A positive correlation between PAR-2 and cathelicidin within rosacea samples was observed (R=0.330, p=0.037). After treatment of PAR-2 AP, both mRNA and protein levels for PAR-2, cathelicidin, and VEGF significantly increased in cultured keratinocytes, compared with PAR-2 control peptide treatment.
Rosacea is a common inflammatory skin disease, most prominent in the central area of the face, namely the cheeks, nose, chin, and forehead, which are densely populated with sebaceous glands.1 Fair-skinned individuals suffer disproportionately from this disease with its chronic relapsing course. The disease is characterized by primary and secondary features: primary features include flushing, persistent erythema, telangiectasia, as well as papules and pustules, while secondary features consist of burning or stinging, plaques, dry appearance, edema or phyma.2 The chronic inflammatory and vascular manifestations of rosacea point to a complex, multifactorial pathophysiology. Interestingly, recent findings suggest that an altered regulation of the innate immune response may be involved.3
Cathelicidins comprise a family of antimicrobial peptides (AMPs) expressed in leukocytes and in various epithelial tissues, where they participate in innate immune responses.4,5 Some cathelicidin peptides, including LL-37 expressed in humans, express both vasoactive and proinflammatory activities.6,7 Significantly higher levels of cathelicidin peptides may be detected in rosacea-affected skin, compared to healthy skin.8 Increased expression of the serine protease kallikrein 5 in the skin of patients with rosacea may promote proteolytic conversion of cathelicidin precursor into the active cathelicidin peptide LL-37.8,9 Abnormally increased expression of LL-37 may induce vascular endothelial growth factor (VEGF) in epidermal keratinocytes, which corresponds to the enhanced inflammation and vascular response seen in rosacea.9,10
Protease-activated receptor 2 (PAR-2), a PAR-family G-protein-coupled 7-transmembrane domain receptor, is activated by serine proteases such as kallikrein, and PAR-2 mediates inflammation in various tissues.11,12,13,14 PAR-2 signaling may also promote expression of the epithelial antimicrobial peptides human β-defensin-2 (hBD-2) and LL-37 as components of innate immunity.15,16 Although expression of the serine protease that activates PAR-2 is increased in rosacea, the regulation of PAR-2 and its role in rosacea remain unclear.
We hypothesized that PAR-2 expression increases in the skin of patients with rosacea and that direct activation of PAR-2 would increase production of cathelicidin LL-37, which may participate in rosacea. To elucidate the role of PAR-2 in the pathogenesis of rosacea, we tested for correlations between cathelicidin and PAR-2 expression in skin samples from patients with rosacea, as well as the induction of cathelicidin expression in cultured keratinocytes upon treatment with PAR-2 activating peptide (AP).
The Institutional Review Board of Severance Hospital approved this study (No. 4-2012-0191). Data for the 40 patients diagnosed with rosacea at the Department of Dermatology, Yonsei University College of Medicine from 2005 to 2010 were reviewed. The diagnosis of rosacea was based on clinical photos and medical charts together with pathological findings. For comparison, 20 samples of facial skin diagnosed as no specific findings or milium without inflammation were selected for review.
Cells of the immortalized human keratinocyte cell line HaCaT were maintained in Dulbecco's Modified Eagle's medium (Welgene, Daegu, Korea) supplemented with 10% fetal bovine serum and 1% penicillin-streptomycin at 37℃ in a humidified CO2 incubator (95% air, 5% CO2). PAR-2 AP, SLIGKV-NH2 (Ser-Leu-Ile-Gly-Lys-Val) (Peptron, Daejeon, Korea) was used to activate PAR-2. Reverse PAR-2 control peptide (CP) (Peptron) was used to determine the specificity of effect of PAR-2 activators. PAR-2 AP (100 µM) was added to HaCaT cells after replacing the culture medium with serum-free medium. In this time-dependent stimulation experiment, HaCaT cells were all plated at the same time, and PAR-2 was then added at the appropriate times thereafter. All HaCaT cells were simultaneously harvested 24 hours after plating.
Hematoxylin-eosin stained slides and paraffin-embedded tissues from each case were retrieved from the surgical files of the Department of Pathology. Each tissue was serially sliced into four sections 3 to 4 µm thick for immunohistochemical analysis. Immunohistochemistry was performed using the polymer detection method (NovoLink Max Polymer Detection System, Leica Biosystems, Newcastle Ltd., Newcastle, UK).
Briefly, sections previously attached to silane-coated slides were dried at 60℃ for 1 hr. The sections were deparaffinized in xylene and rehydrated in graded alcohol and distilled water. For antigen retrieval, the sections were put into 10 mM citrate buffer (pH 6.0) and boiled in a microwave oven. After cooling at room temperature, sections were treated with a peroxidase block kit for 15 min, washed with Tris-buffered saline (TBS) for 5 min, and incubated with primary antibodies at room temperature for 1 hr.
The antibodies and dilutions used were as follows: PAR-2 (rabbit polyclonal anti-human, Santa Cruz Biotechnology, Santa Cruz, CA, USA, 1:200) and cathelicidin (rabbit polyclonal anti-human, Abcam, Cambridge, MA, USA, 1:200). Slides were washed with TBS three times, and then reacted with Post Primary (included in the kit) at room temperature for 30 min. After washing with TBS three times, slides were reacted with polymer for 30 min. Color was developed using the NovaRED substrate kit (VECTOR Laboratory, Burlingame, CA, USA). The reaction was stopped with distilled water while being observed under a microscope. Harris hematoxylin was used as a counterstain.
Slides were examined at 400× magnification. Two investigators independently reviewed slides without knowledge of the clinical data. The intensity of keratinocytes showing immunoreactivity to each marker in more than three high powered fields was assessed semi-quantitatively using the following grading system: 0=no staining, 1=weak staining, 2=moderate staining, and 3=strong staining.
Total RNA was extracted using the RNeasy mini-kit (Qiagen, Valencia, CA, USA) according to the manufacturer's instructions. One microgram of total RNA was used as the first strand for cDNA synthesis with Primescript™ (TaKaRa Bio Inc., Otsu, Japan). For all quantitative real-time RT-PCR analyses, amplification was performed in a Step One Real-Time PCR System (Applied Biosystems, Foster City, CA, USA) and visualized with a Power SYBR GREEN MASTER MIX (Applied Biosystems). The cycling conditions were as follows: 2 min at 50℃, 10 min at 95℃, and 40 cycles of 95℃ for 15 seconds, and 60℃ for 1 min. For each specific mRNA analysis, gene expression was normalized to glyceraldehyde 3-phosphate dehydrogenase (GAPDH) mRNA in the same sample. The primer sequences used were as follows: PAR-2 forward, 5'-GGCCCTGTGGGTCTTTCTTTT-3', and reverse, 5'-CAAGGGGAACCAGATGACAGA-3'; forward 5'-GGCTGGTGAAGCGGTGTAT-3', and reverse, 5'-TGGGTACAAGATTCCGCAAAAA-3' (cathelicidin); forward, 5'-CGCAGCTACTGCCATCCAAT-3', reverse, 5'-GTGAGGTTTGATCCGCATAATCT-3' (VEGF); forward, 5'-GCTCCTCCTGTTCGACAGTCA-3' and reverse, 5'-ACCTTCCATGGTGTCTGA-3' (GAPDH).
Relative quantification was performed using the ΔΔCt (threshold cycle) method, where ΔCt was (target Ct-GAPDH Ct), and ΔΔCt was (ΔCt sample-ΔCt untreated control). The quantity of the sample relative to control mRNA was 2-ΔΔCt. The mRNA expression was indicated with a Ct value and was corrected for GAPDH expression.
Whole cell extracts were prepared in RIPA buffer (TBS, 0.5% deoxycholate, 0.1% SDS, 1% Triton X-100) containing protease inhibitor cocktail (Sigma, St. Louis, MO, USA). Proteins isolated from HaCaT cells after treatment with PAR-2 AP were separated by SDS-PAGE on 15% gels, and then transferred to nitrocellulose membranes using standard procedures. The membranes were blocked with 5% v/v skim milk in a TBS-T buffer (TBS with 0.1% w/v Tween-20) and reacted with primary antibodies overnight at 4℃. Primary antibodies against PAR-2 (Santa Cruz Biotechnology), cathelicidin (Abcam), and VEGF (Santa Cruz Biotechnology, Santa Cruz, CA, USA) were used at a 1:1000 dilution. The membranes were then washed three times for 15 min each with the TBS-T buffer and incubated for 1 hr with 1% skim milk in TBS-T buffer containing horseradish peroxidase-conjugated secondary antibodies (1:2000). The proteins were visualized using an enhanced chemiluminescence system (Thermo Scientific, Bremen, Germany). GAPDH (Santa Cruz Biotechnology) was used as a control for protein loading.
Statistical analyses were performed using Statistical Package for the Social Sciences (SPSS Inc., Chicago, IL, USA) for Windows. Staining intensities of PAR-2 and cathelicidin were compared between normal and rosacea-affected skin using the Student's t-test. The relationship between PAR-2 and cathelicidin staining intensities was tested by Pearson correlation analysis. Differences in PAR-2, cathelicidin and VEGF expression between treatment with PAR-2 AP and PAR-2 CP were analyzed with the Student's t-test. Significance was analyzed at the level of 0.05.
Among the 40 patients with rosacea enrolled in this study, 10 were male and 30 were female. Their ages ranged from 21 to 76 years (mean, 47.2±13.8 years). The physician classified each patient by rosacea subtype and graded the severity of their disease. Twelve patients (30%) were diagnosed with erythematotelangiectatic rosacea (ETR) and 28 patients (70%) were diagnosed with papulopustular rosacea (PPR). No patients presented with the phymatous subtype. Of the 40 patients, six patients (15%) were graded as mild, 24 (60%) were graded as moderate, and 10 (25%) were graded as severe, based on the severity of erythema (Table 1).17
Histopathologically, vascular dilation with perivascular and perifollicular lymphohistiocytic inflammation of varying degrees was noted in the upper dermis of lesional skin from patients with rosacea. Mean staining intensities of PAR-2 and cathelicidin in rosacea were 1.7±0.6 and 1.8±0.7, respectively. Mean staining intensities of PAR-2 and cathelicidin in normal skin were 1.3±1.0 and 0.5±0.3, respectively. Cathelicidin expression in rosacea skin tissues was significantly higher than that in normal tissues (p<0.001). Rosacea tissues showed detectably greater PAR-2 expression than normal skin tissues, although the difference did not reach statistical significance. Additionally, differences in staining intensities between PAR-2 and cathelicidin according to clinical severity were not significant (Table 2). However, a significant correlation between clinical severity and grade of inflammation was observed (p=0.018). As well, differences in expressions of PAR-2 and cathelicidin between subtypes of rosacea, such as ETR and PPR, were also not significant.
The level of cathelicidin expression according to PAR-2 expression was examined. As PAR-2 expression increased, a trend toward an increase in cathelicidin expression was shown (beta=0.330, p=0.037) (Fig. 1). In Figs. 2 and 3, two patients with rosacea who showed high expression of PAR-2 and cathelicidin in affected skin tissues are presented together with their immunohistochemical findings.
After 24-hr incubation of keratinocytes with PAR-2 AP, the levels of PAR-2, cathelicidin, and VEGF mRNA were significantly higher than those treated with PAR-2 CP (p<0.05) (Fig. 4). In Western blotting, increased levels of PAR-2, cathelicidin, and VEGF proteins were observed after a 24-hr treatment with PAR-2 AP (Fig. 5).
The complex pathophysiology of rosacea suggests a multifactorial etiology with vascular and immunological components underlying a chronic inflammatory response.18 Prominent vasodilation in the affected skin may explain the persistent erythema.19 Microbial agents, notably bacteria associated with Demodex folliculorum infestation, are suspected to activate immune responses in patients predisposed to rosacea.20,21 Altered adaptive and innate immune responsiveness and enhanced cellular reactivity are hypothesized. Abnormal innate immunity is suggested by detection of an AMP and its processing serine protease at higher levels in rosacea lesions than in normal skin.8 In the present study, we tested the involvement of PAR-2 in the expression of AMPs that may contribute to rosacea.
Two important and well-studied types of AMPs in human skin are defensins and cathelicidin LL-37.22,23 hBD-2, the first AMP characterized in human skin, is induced in skin inflammation and infection, and is most effective against gram-negative bacteria.24 Human cathelicidin LL-37, active against both gram-positive and gram-negative bacteria, may be detected directly in human skin only at sites of inflammation.25 Additional functions of LL-37 include chemotactic activation and modulation of cytokines and inflammatory mediators such as cyclooxygenase-2, pro-interleukin (IL) 1b, and IL-8 in keratinocytes.26 Aberrant LL-37 expression at inflammatory sites modifies dendritic cell differentiation, thereby linking innate and adaptive immune responses. The LL-37-activated dendritic cells may potentiate expression of co-stimulatory molecules and a Th1 response in vitro.27 In cultured endothelial cells, LL-37 induces angiogenesis and triggers the proliferation of vessel-like structures.6 Effects induced in vitro by LL-37 correspond to changes seen in rosacea, and altered cathelicidin expression is observed in rosacea-affected skin.8 Although the regulation of cathelicidin LL-37 production is incompletely understood, proteolytic processing of a precursor protein is essential for its activation as a pro-inflammatory molecule.28 The abundance of LL-37 in the lesions of rosacea may result through abnormal production or activity of an epidermal serine protease or of kallikrein, which process the cathelicidin precursor.8,9 Cathelicidin LL-37 is subject to regulation by various factors, including cytokines, bacterial metabolites such as butyrate, hypoxia, and exposure to the sunlight through up-regulation of human cathelicidin antimicrobial protein (hCAP18) expression by vitamin D3.29,30,31,32
The PARs comprise a subfamily of G protein-coupled, 7-transmembrane domain receptors.12,33 PAR-1, -3, and -4 are activated by thrombin, whereas PAR-2 is activated by trypsin-like serine proteases such as kallikrein. Epidermal cells produce several kallikreins, including kallikrein 5 and kallikrein 14, which activate PAR-2.14,34 Detection of PAR-2 and kallikrein 14 in rosacea lesions has been widely reported.35 From this we hypothesized that direct activation of PAR-2 in rosacea lesions may increase cathelicidin expression.
Initially, we found significantly higher cathelicidin expression in rosacea skin tissues than in normal skin, although PAR-2 expression did not differ significantly between normal skin and rosacea. Cathelicidin expression also showed a significant positive correlation with PAR-2 expression on immunohistochemical staining. These findings may plausibly reflect an interaction between PAR-2 and cathelicidin in the pathogenesis of rosacea. Additionally, both cathelicidin and PAR-2 receptor mRNA and protein increased in keratinocytes treated with PAR-2 AP in vitro. These results suggested that not only PAR-2 expression but also cathelicidin could be regulated by direct activation of PAR-2. That is, PAR-2 may serve to trigger an inflammatory response through induction of cathelicidin in keratinocytes. However, as a difference in staining intensities of PAR-2 and cathelicidin according to inflammation and clinical severity was not observed in our study, in the future, further studies regarding relationship between cathelicidin expression and inflammation induction are needed.
PAR-2 AP induces intercellular adhesion molecule-1 expression in human keratinocytes by activating nuclear factor-kappa B.36 Activation of PAR-2 in keratinocytes may also increase production of IL-6, granulocyte-macrophage colony-stimulating factor and IL-8/CXCL 8, promoting granulocyte and T-cell recruitment.37,38 In addition, a reduction of ear swelling and inflammatory infiltrates in PAR-2 (-/-) mice used in a model of contact hypersensitivity indicates a pro-inflammatory role for PAR-2 in allergic dermatitis.39 Just as PAR-2, which is constitutively expressed in epithelial cells, mediates inflammation in diverse tissues, so may PAR-2 present a potentially effective target for the treatment of inflammatory disease.
Interestingly, keratinocytes treated with PAR-2 AP in vitro increased expression of PAR-2 itself. To our knowledge, PAR-2 AP-induced PAR-2 expression has not been previously reported. While PAR-2 activation may stimulate various signaling cascades, including the activation of inflammatory cytokines, conversely, inflammatory cytokines or growth factors, such as IL-1β, TNF-α, TGF-β, and platelet-derived growth factor may stimulate PAR-2 expression.40,41 We therefore considered the possibility that PAR-2 activation by exogenous PAR-2 AP proceeds through a specific cascade leading to PAR-2 expression.
Contrary to expectation, PAR-2 expression did not differ significantly between normal and rosacea-affected skin. Unlike cathelicidin, PAR-2 is constitutively expressed in normal keratinocytes, and judging from immunohistochemical staining results, does not seem to be affected by increased serine protease activity, which is highly expressed in rosacea patients. However, despite the lack of statistical significance, PAR-2 expression in rosacea-affected skin was higher than that in normal skin.
Our findings are limited in that we could not directly compare cathelicidin and PAR-2 expression between lesions and non-lesions within rosacea patients, as this would involve an invasive procedure with cosmetic risks. Similarly, the activity of serine protease was not evaluated because frozen skin tissues are needed for such assay.
Based on our results, we postulated that increased expression of PAR-2 and serine proteases induced by exogenous irritants and aggravating factors may lead to production of cathelicidin itself through PAR-2 signaling and to excessive LL-37 production through processing by serine proteases and that both pathways contribute to the pathology of rosacea. In conclusion, PAR-2 may contribute to the pathogenesis of rosacea through regulatory action of innate immune response. Molecular antagonists of PAR-2 present a plausible therapeutic intervention for rosacea.
ACKNOWLEDGEMENTS
This study was supported by the "ILJIN" Faculty Research Assistance Program of Yonsei University College of Medicine in 2012 (6-2012-0095).
References
1. Korting HC, Schöllmann C. Current topical and systemic approaches to treatment of rosacea. J Eur Acad Dermatol Venereol. 2009; 23:876–882. PMID: 19508315.


2. Wilkin J, Dahl M, Detmar M, Drake L, Feinstein A, Odom R, et al. Standard classification of rosacea: Report of the National Rosacea Society Expert Committee on the Classification and Staging of Rosacea. J Am Acad Dermatol. 2002; 46:584–587. PMID: 11907512.


3. Yamasaki K, Gallo RL. Rosacea as a disease of cathelicidins and skin innate immunity. J Investig Dermatol Symp Proc. 2011; 15:12–15.


4. Nizet V, Ohtake T, Lauth X, Trowbridge J, Rudisill J, Dorschner RA, et al. Innate antimicrobial peptide protects the skin from invasive bacterial infection. Nature. 2001; 414:454–457. PMID: 11719807.


5. Zanetti M. The role of cathelicidins in the innate host defenses of mammals. Curr Issues Mol Biol. 2005; 7:179–196. PMID: 16053249.
6. Koczulla R, von Degenfeld G, Kupatt C, Krötz F, Zahler S, Gloe T, et al. An angiogenic role for the human peptide antibiotic LL-37/hCAP-18. J Clin Invest. 2003; 111:1665–1672. PMID: 12782669.


7. Braff MH, Hawkins MA, Di Nardo A, Lopez-Garcia B, Howell MD, Wong C, et al. Structure-function relationships among human cathelicidin peptides: dissociation of antimicrobial properties from host immunostimulatory activities. J Immunol. 2005; 174:4271–4278. PMID: 15778390.


8. Yamasaki K, Di Nardo A, Bardan A, Murakami M, Ohtake T, Coda A, et al. Increased serine protease activity and cathelicidin promotes skin inflammation in rosacea. Nat Med. 2007; 13:975–980. PMID: 17676051.


9. Yamasaki K, Schauber J, Coda A, Lin H, Dorschner RA, Schechter NM, et al. Kallikrein-mediated proteolysis regulates the antimicrobial effects of cathelicidins in skin. FASEB J. 2006; 20:2068–2080. PMID: 17012259.


10. Detmar M, Brown LF, Claffey KP, Yeo KT, Kocher O, Jackman RW, et al. Overexpression of vascular permeability factor/vascular endothelial growth factor and its receptors in psoriasis. J Exp Med. 1994; 180:1141–1146. PMID: 8064230.


11. Hansen KK, Oikonomopoulou K, Li Y, Hollenberg MD. Proteinases, proteinase-activated receptors (PARs) and the pathophysiology of cancer and diseases of the cardiovascular, musculoskeletal, nervous and gastrointestinal systems. Naunyn Schmiedebergs Arch Pharmacol. 2008; 377:377–392. PMID: 17952408.


12. Coughlin SR. Protease-activated receptors start a family. Proc Natl Acad Sci U S A. 1994; 91:9200–9202. PMID: 7937741.


13. Rattenholl A, Steinhoff M. Proteinase-activated receptor-2 in the skin: receptor expression, activation and function during health and disease. Drug News Perspect. 2008; 21:369–381. PMID: 19259550.


14. Stefansson K, Brattsand M, Roosterman D, Kempkes C, Bocheva G, Steinhoff M, et al. Activation of proteinase-activated receptor-2 by human kallikrein-related peptidases. J Invest Dermatol. 2008; 128:18–25. PMID: 17625593.


15. Chung WO, Hansen SR, Rao D, Dale BA. Protease-activated receptor signaling increases epithelial antimicrobial peptide expression. J Immunol. 2004; 173:5165–5170. PMID: 15470061.


16. Lee SE, Kim JM, Jeong SK, Jeon JE, Yoon HJ, Jeong MK, et al. Protease-activated receptor-2 mediates the expression of inflammatory cytokines, antimicrobial peptides, and matrix metalloproteinases in keratinocytes in response to Propionibacterium acnes. Arch Dermatol Res. 2010; 302:745–756. PMID: 20697725.


17. Elewski BE, Fleischer AB Jr, Pariser DM. A comparison of 15% azelaic acid gel and 0.75% metronidazole gel in the topical treatment of papulopustular rosacea: results of a randomized trial. Arch Dermatol. 2003; 139:1444–1450. PMID: 14623704.


18. Crawford GH, Pelle MT, James WD. Rosacea: I. Etiology, pathogenesis, and subtype classification. J Am Acad Dermatol. 2004; 51:327–341. PMID: 15337973.


19. Neumann E, Frithz A. Capillaropathy and capillaroneogenesis in the pathogenesis of rosacea. Int J Dermatol. 1998; 37:263–266. PMID: 9585896.
20. Lacey N, Delaney S, Kavanagh K, Powell FC. Mite-related bacterial antigens stimulate inflammatory cells in rosacea. Br J Dermatol. 2007; 157:474–481. PMID: 17596156.


21. McAleer MA, Fitzpatrick P, Powell FC. Papulopustular rosacea: prevalence and relationship to photodamage. J Am Acad Dermatol. 2010; 63:33–39. PMID: 20462665.


22. Selsted ME, Ouellette AJ. Mammalian defensins in the antimicrobial immune response. Nat Immunol. 2005; 6:551–557. PMID: 15908936.


23. Zaiou M, Gallo RL. Cathelicidins, essential gene-encoded mammalian antibiotics. J Mol Med (Berl). 2002; 80:549–561. PMID: 12226737.


24. Schröder JM, Harder J. Human beta-defensin-2. Int J Biochem Cell Biol. 1999; 31:645–651. PMID: 10404637.


25. Murakami M, Ohtake T, Dorschner RA, Schittek B, Garbe C, Gallo RL. Cathelicidin anti-microbial peptide expression in sweat, an innate defense system for the skin. J Invest Dermatol. 2002; 119:1090–1095. PMID: 12445197.


26. Wu WK, Wong CC, Li ZJ, Zhang L, Ren SX, Cho CH. Cathelicidins in inflammation and tissue repair: potential therapeutic applications for gastrointestinal disorders. Acta Pharmacol Sin. 2010; 31:1118–1122. PMID: 20676121.


27. Davidson DJ, Currie AJ, Reid GS, Bowdish DM, MacDonald KL, Ma RC, et al. The cationic antimicrobial peptide LL-37 modulates dendritic cell differentiation and dendritic cell-induced T cell polarization. J Immunol. 2004; 172:1146–1156. PMID: 14707090.


28. Zaiou M, Nizet V, Gallo RL. Antimicrobial and protease inhibitory functions of the human cathelicidin (hCAP18/LL-37) prosequence. J Invest Dermatol. 2003; 120:810–816. PMID: 12713586.


29. Steinmann J, Halldórsson S, Agerberth B, Gudmundsson GH. Phenylbutyrate induces antimicrobial peptide expression. Antimicrob Agents Chemother. 2009; 53:5127–5133. PMID: 19770273.


30. Gombart AF, O'Kelly J, Saito T, Koeffler HP. Regulation of the CAMP gene by 1,25(OH)2D3 in various tissues. J Steroid Biochem Mol Biol. 2007; 103:552–557. PMID: 17368184.


31. Yim S, Dhawan P, Ragunath C, Christakos S, Diamond G. Induction of cathelicidin in normal and CF bronchial epithelial cells by 1,25-dihydroxyvitamin D(3). J Cyst Fibros. 2007; 6:403–410. PMID: 17467345.


32. White JH. Vitamin D as an inducer of cathelicidin antimicrobial peptide expression: past, present and future. J Steroid Biochem Mol Biol. 2010; 121:234–238. PMID: 20302931.


33. Kawabata A. PAR-2: structure, function and relevance to human diseases of the gastric mucosa. Expert Rev Mol Med. 2002; 4:1–17. PMID: 14585156.


34. Sondell B, Thornell LE, Stigbrand T, Egelrud T. Immunolocalization of stratum corneum chymotryptic enzyme in human skin and oral epithelium with monoclonal antibodies: evidence of a proteinase specifically expressed in keratinizing squamous epithelia. J Histochem Cytochem. 1994; 42:459–465. PMID: 7510318.


35. Hachem JP, Man MQ, Crumrine D, Uchida Y, Brown BE, Rogiers V, et al. Sustained serine proteases activity by prolonged increase in pH leads to degradation of lipid processing enzymes and profound alterations of barrier function and stratum corneum integrity. J Invest Dermatol. 2005; 125:510–520. PMID: 16117792.


36. Buddenkotte J, Stroh C, Engels IH, Moormann C, Shpacovitch VM, Seeliger S, et al. Agonists of proteinase-activated receptor-2 stimulate upregulation of intercellular cell adhesion molecule-1 in primary human keratinocytes via activation of NF-kappa B. J Invest Dermatol. 2005; 124:38–45. PMID: 15654951.


37. Wakita H, Furukawa F, Takigawa M. Thrombin and trypsin induce granulocyte-macrophage colony-stimulating factor and interleukin-6 gene expression in cultured normal human keratinocytes. Proc Assoc Am Physicians. 1997; 109:190–207. PMID: 9069588.
38. Hou L, Kapas S, Cruchley AT, Macey MG, Harriott P, Chinni C, et al. Immunolocalization of protease-activated receptor-2 in skin: receptor activation stimulates interleukin-8 secretion by keratinocytes in vitro. Immunology. 1998; 94:356–362. PMID: 9767417.
39. Kawagoe J, Takizawa T, Matsumoto J, Tamiya M, Meek SE, Smith AJ, et al. Effect of protease-activated receptor-2 deficiency on allergic dermatitis in the mouse ear. Jpn J Pharmacol. 2002; 88:77–84. PMID: 11859856.


40. Gruber BL, Marchese MJ, Santiago-Schwarz F, Martin CA, Zhang J, Kew RR. Protease-activated receptor-2 (PAR-2) expression in human fibroblasts is regulated by growth factors and extracellular matrix. J Invest Dermatol. 2004; 123:832–839. PMID: 15482468.


41. Xiang Y, Masuko-Hongo K, Sekine T, Nakamura H, Yudoh K, Nishioka K, et al. Expression of proteinase-activated receptors (PAR)-2 in articular chondrocytes is modulated by IL-1beta, TNF-alpha and TGF-beta. Osteoarthritis Cartilage. 2006; 14:1163–1173. PMID: 16757188.
Fig. 1
Significant correlation was observed between PAR-2 and cathelicidin expression in rosacea (R=0.330, p=0.037). PAR-2, protease-activated receptor-2.

Fig. 2
Clinical picture of a 38-year-old male patient with papulopustular rosacea (A) and immunohistochemical findings of PAR-2 (B) and cathelicidin (C) (H&E, ×400). Staining intensity of PAR-2, 2.5; cathelicidin, 2.5. Cathelicidin staining on normal skin tissue (staining intensity, 0) (D). PAR-2, protease-activated receptor-2.

Fig. 3
Clinical picture of a 69-year-old female patient with papulopustular rosacea (A) and immunohistochemical findings of PAR-2 (B) and cathelicidin (C) (H&E, ×400). Staining intensity of PAR-2, 2.5; cathelicidin 3. Cathelicidin staining on normal skin tissue (staining intensity, 0) (D). PAR-2, protease-activated receptor-2.
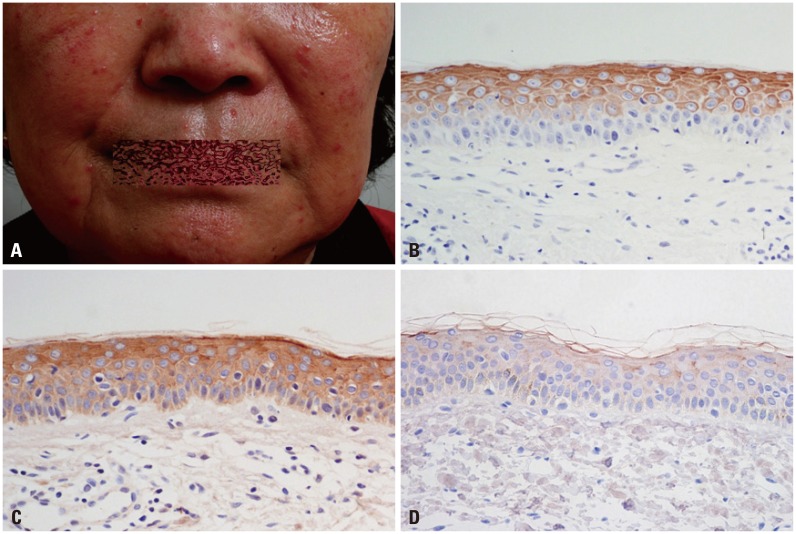
Fig. 4
Effect of PAR-2 AP on the mRNA expression of PAR-2, cathelicidin and VEGF in HaCaT cells. Real time RT-PCR of PAR-2, cathelicidin and VEGF in HaCaT cells after PAR-2 activating peptide and PAR-2 control peptide treatment. Each data point represents the mean (±SEM) result from three independent experiments. *p<0.05. AP, activating peptide; CP, control peptide; VEGF, vascular endothelial growth factor; PAR-2, protease-activated receptor-2; SEM, standard error of the mean.

Fig. 5
Effect of PAR-2 AP on the expression of PAR-2, cathelicidin and VEGF proteins in HaCaT cells. Western blotting against PAR-2, cathelicidin and VEGF in HaCaT cells after PAR-2 activating peptide and PAR-2 control peptide treatment. AP, activating peptide; CP, control peptide; GAPDH, glyceraldehyde phosphate dehydrogenase; PAR-2, protease-activated receptor-2; VEGF, vascular endothelial growth factor.
