Abstract
Purpose
We investigated the correlations between optical quality parameters obtained from the double-pass system and ocular aberrations obtained from the ray-tracing aberrometer in multifocal intraocular lens (IOL) implanted eyes.
Materials and Methods
Twenty eyes from 20 patients were enrolled in this study. Modulation transfer function cutoff frequency, The Strehl ratio, objective scatter index, and objective pseudo-accommodation obtained from the double-pass system were compared with root mean square (RMS) total aberration, RMS higher-order aberration, and spherical aberration obtained from the ray-tracing aberrometer. Additionally, parameters of the double-pass system and ray-tracing aberrometer were compared with manifested refraction values and subjective visual acuity, respectively.
Results
There was no statistically significant correlation between optical quality parameters obtained from the double-pass system and ocular aberrations, except between the Strehl ratio and RMS total aberration (r=-0.566, p=0.018). No significant correlations were found between the parameters of both devices, and manifested refraction values or subjective visual acuity.
Conclusion
Optical quality parameters, especially the Strehl ratio, in multifocal IOL implanted eyes were affected by RMS total aberration. Further studies based on accurate measurements of ocular aberrations and additional optical quality parameters are needed to delineate relationships between optical quality parameters and ocular aberrations in multifocal IOL implanted eyes.
Cataract surgery is both a therapeutic and refractive surgery for restoring normal sight. Multifocal intraocular lenses (IOLs) are designed to overcome the lack of accommodation by providing useful distance and near vision.1,2 Previous studies have confirmed the efficacy of different types of multifocal IOLs.3,4,5 Despite improved visual performance after cataract surgery, some optical difficulties such as decreased contrast sensitivity, glare, and halos have been reported.4,5,6 In these studies, measurements of visual acuity alone were not sufficient to explain the optical condition of multifocal IOL implanted eyes.
Contrast sensitivity, modulation transfer function (MTF), point spread function (PSF), and ocular aberrations have been used to evaluate optical quality after cataract surgery.7,8,9,10,11 Ocular aberrations are well known parameters that could represent optical quality.12 Wavefront analysis has been widely used to isolate the effects of lower order aberrations and higher order aberrations, and to evaluate the contributions of individual aberrations on optical quality.13 With the development of new techniques and devices such as the double-pass system, optical quality can also be measured more precisely.8,14,15,16 The Optical Quality Analysis System (Visiometrics, Terrassa, Spain), a commercially available double-pass device, has been introduced to measure optical quality associated parameters objectively and accurately in clinical practice.17 The double-pass technique is based on recording images from a point source of infrared light after reflection on the retina and a double pass through ocular media.17,18 The size and shape of this light spot are quantified by measuring the PSF.17 Clinically, the double-pass system provides data on retinal image quality [MTF cutoff frequency (MTF cutoff)], Strehl ratio, intraocular scattering [objective scatter index (OSI)], and objective pseudo-accommodation. The value of MTF in the double-pass system is directly computed by Fourier transformation from the acquired double-pass retinal image.17,18 The double-pass system also allows objective measurement of the effect of ocular aberrations on optical quality of the human eye and evaluation of the quality and stability of the tear film in detecting mild symptoms of dry eye.13,19,20
Previous studies reported the impact of ocular aberrations on objective optical quality in monofocal IOL implanted eyes using the double-pass system.13,21 However, there were limited reports evaluating the impact in multifocal IOL implanted eyes using the double-pass system. Although there were some studies evaluating the optical quality parameters (PSF, MTF, and Sterhl ratio) measured with the double-pass system, they did not measured the value of the OSI and objective pseudo-accommodation.8,22
The Hartmann-Shack technique was unreliable for measurements of ocular aberrations in diffractive multifocal IOL implanted eyes.23,24 The diffractive multifocal IOL is composed with a large number of Fresnel edges and a central 2 mm free Fresnel area. When using the Hartmann-Shack aberrometer, measurement error could occur when the reflected light arrives at the detector, which has limited resolution from the high wavefront aberration of a multifocal IOL. In contrast, the ray-tracing aberrometer obtains ocular aberrations using a position sensitive sensor to detect the displacement of a laser beam reflected from the retina. With the ray-tracing aberrometer, the projected light is scattered when passing through the multifocal IOL, but the reflected image is less affected. Because of this difference, the ray-tracing aberrometer could provide a more accurate ocular aberration measurement than the Hartmann-Shack type aberrometer in multifocal IOL implanted eyes.25
Therefore, in the present study we measured and investigated the correlations between optical quality parameters such as MTF cutoff, Strehl ratio, and objective pseudo-accommodation obtained from the double-pass system and ocular aberrations obtained from the ray-tracing aberrometer in multifocal IOL implanted eyes. The effect of ocular scatter on optical quality was evaluated. Optical parameters obtained from these two devices were compared with subjective visual acuity and manifested refraction values.
This study included 20 eyes from 20 patients who underwent cataract surgery with multifocal IOLs (AcrySof IQ ReSTOR SN6AD1, Alcon, Inc., Fort Worth, TX, USA). All patients were treated at Yonsei University College of Medicine, Seoul, Korea. The same surgeon (T-I Kim) performed all the procedures. This study was approved retrospectively by the Institutional Review Board of Severance Hospital and conducted according to the Declaration of Helsinki and Good Clinical Practices. All patients signed documents of informed consent for tests and analysis of the results. The IOLs were selected to achieve emmetropia.
Inclusion criteria for patients were age (40 to 70 years of age) and patients who had undergone operations for senile cataract extraction and multifocal IOL implantation. Exclusion criteria included previous ocular or intraocular surgery, evidence of trauma on biomicroscopic examination, corneal opacity, fundus abnormalities, glaucoma, uveitis, amblyopia, systemic disease, posterior capsule rupture during surgery, IOL decentration >0.5 mm, or corrected distance visual acuity (CDVA) worse than 0.1 logarithm of the minimum angle of resolution (logMAR) (20/25 or better on the Snellen chart), postoperatively. A Scheimpflug imaging system (Pentacam, OCULUS Optikgeräte GmbH, Wetzlar, Germany) was used to assess decentration after cataract surgery.26 Any eye with concurrent disease that might influence optical or neural performance was excluded. When a patient underwent operations in both eyes, one eye was randomly selected to avoid correlation effects in statistical analyses. Randomization sequence was created using EXCEL 2007 (Microsoft, Redmond, WA, USA) using random block sizes of 2 and 4 by an independent staff member.
Postoperative evaluations were performed three months after cataract surgery. All patients were checked for uncorrected distance visual acuity (UCDVA), CDVA, manifested refraction values (sphere, cylinder, and spherical equivalent), objective optical quality, and by slit-lamp biomicroscopy
Objective evaluation of optical quality parameters was performed using the double-pass system. A dim light was maintained to assure at least a 4 mm natural pupil diameter. In our study, based on an unequal pupil configuration of the double-pass system, entrance pupil had a fixed diameter of 2 mm.27 Measurements of optical quality were performed at a 4 mm pupil diameter (the exit pupil) without pharmacologic dilatation.27 The double-pass system automatically compensated for a patient's spherical refractive error; however, cylinder error was not corrected by the machine. We corrected patients' cylindrical error with a trial lens. For each parameter, the double-pass device took 6 measurements and calculated the mean of the measurements.
The first parameter was the MTF cutoff (cycles per degree, cpd). The MTF cutoff is the frequency at which the MTF reaches a value of 0.01. The second parameter was the Strehl ratio, which is the ratio of peak focal intensity in aberration versus an ideal PSF.13 The Strehl ratio provides general information about the eye's optical quality. A value of 1 corresponds to a perfect zero-aberration optical system. The third double-pass parameter was the OSI. The OSI is defined as the ratio between the integrated light in the periphery and in the surroundings of the central peak of the double-pass image.28 In the particular case of the instrument, the central area was selected as a circle of a radius of 1 minute of arc (MOA), and the peripheral zone was selected as a ring set between 12 and 20 MOA.28 Objective pseudo-accommodation was the fourth parameter, which was calculated using the aberration PSF, the focus range at which the PSF of the defocus point is better than 50% of the maximum PSF.
Ocular aberrations were measured using the ray-tracing aberrometer (iTrace, Tracey Technologies, Houston, TX, USA) at a pupil size of 4 mm or more, without pharmacologic dilatation. Data were then recalculated at a 4 mm sized pupil for comparison with the double-pass system measurements.29 The root mean square (RMS) total aberration, RMS higher-order aberration, and spherical aberration were measured. RMS higher-order aberration was analyzed up to the 6th order by expanding the set of Zernike polynomials.
To compare the MTF obtained from these two devices directly and easily, we manually measured spatial frequency when the MTF reached 10% of its maximum value (MTF10).
LogMAR acuity values were used for statistical analysis of visual acuity. We analyzed the correlations between optical quality parameters (MTF cutoff, Strehl ratio, and objective pseudo-accommodation) obtained from the double-pass system and ocular aberrations (RMS total aberration, RMS higher-order aberration, and spherical aberration) obtained from the ray-tracing aberrometer. The effect of ocular scatter on optical quality was investigated using the OSI value obtained from the double-pass system. The correlations between the above mentioned parameters and other visual quality parameters (manifested refraction values, CDVA, and UCDVA) were determined. The correlations were evaluated using Spearman's correlation analysis. The Wilcoxon signed-rank test was used for comparisons of MTF10. Statistical analyses were performed using SAS software (version 9.2, SAS Institute, Inc., Cary, NC, USA). Differences were considered statistically significant for p<0.05.
Among the 20 patients, 10 (50.0%) were male and 10 (50.0%) female. Postoperative data are shown in Table 1. Spearman's correlation analysis showed significant negative correlation between the Strehl ratio and total aberration (r=-0.566, p=0.018) (Table 2). There were no other statistically significant correlations between optical quality parameters (MTF cutoff, Strehl ratio) and ocular aberrations (RMS total aberration, RMS higher-order aberration, and spherical aberration). Statistically, the OSI did not correlate with any optical quality parameters (MTF cutoff, Strehl ratio, and objective pseudo-accommodation) (Table 2). The mean objective pseudo-accommodation was 1.55±0.64 diopters (D), which did not correlate with any ocular aberrations (Table 2).
In terms of manifested refraction values (sphere, cylinder, and spherical equivalent) and subjective visual acuity, we did not find any correlations with MTF cutoff, Strehl ratio, and objective pseudo-accommodation (Table 3). There was no statistically significant correlation between ocular aberrations and manifested refraction values or subjective visual acuity (Table 4).
The MTF10 obtained from the double-pass system was 19.65±10.94, which is significantly smaller than that obtained from the ray-tracing aberrometer, 11.00±7.50 (p=0.005, Wilcoxon signed-rank test).
In the present study, we analyzed the effects of ocular aberrations on objective optical quality parameters in multifocal IOL implanted eyes. We measured objective optical quality and ocular aberrations using the double-pass system and ray-tracing type aberrometer, respectively. Our results showed significant negative correlation between retinal image quality parameter (Strehl ratio) and total aberration.
The effect of ocular aberrations could be summarized as blurring of the retinal image.21 Thus, ocular aberrations cause retinal image degradation, which is related to Strehl ratio. Unlike the MTF which represents contrast information, the Strehl ratio signifies the intensity of the image brightness.30 We confirmed that the Strehl ratio was negatively correlated with total aberration, implying that ocular aberration may affect contrast and intensity of the brightness differently.
In recently published studies evaluating the effect of ocular aberrations on objective optical quality in monofocal IOL implanted eyes, Nochez, et al.13,21 reported inverse correlations between ocular aberrations (2nd order astigmatism, trefoil, and spherical aberration) obtained from the Hartmann-Shack aberrometer and MTF cutoff obtained from the double-pass system. The same group also reported that pseudo-accommodation range was positively correlated with spherical aberration in monofocal IOL implanted eyes.13
However, in the present study, MTF cutoff was not statistically correlated with any ocular aberrations. The difference between our study and the aforementioned study could originate from the unique design of the multifocal IOLs. Before the evaluation of ocular aberration and optical quality, the unique design of multifocal IOLs should be considered. Diffractive multifocal IOLs are designed to allow the visible light near the peak of the visual response curve to be split between the first order (near addition) and the zero order (no power addition). Therefore, the split will be progressively biased toward the first order for shorter wavelengths, and toward the zero order for longer wavelengths.31 With the double-pass system, near infrared wavelength (780 nm) is used, whereas the ray-tracing aberrometer uses a laser with a wavelength of 632 nm. Because the double-pass system uses relatively long wavelength, most light energy will be directed into zero order (non-deviated) after diffraction by the diffractive multifocal IOL. A much smaller percentage of the infrared light will be diffracted in the first order compared with the ray-tracing aberrometer. Therefore, the effect of the diffraction at visible wavelengths in the first order will be significantly underestimated.31 This underestimation could be the reason for poor correlation between ocular aberrations and optical quality parameters.
An alternative explanation is that the methodology for recalculating optical quality parameters and ocular aberrations with a 4 mm pupil could account for the difference between our study and other studies analyzed using a 6 mm pupil.21 Pupil size has been reported to induce significant differences in the higher-order aberration in pseudophakic eyes.32 Thus, the effect of the higher-order aberration measured under relatively small pupils on optical quality could be underestimated.
In this study, we did not find any significant correlations between parameters obtained from the double-pass system and CDVA. Poor correlations between optical quality parameters and CDVA imply that there should be a discrepancy between optical quality and visual acuity in multifocal IOL implanted eyes. It is apparent that visual acuity alone is not sufficient to evaluate postoperative optical quality in the multifocal IOL implanted eye. Thus, measuring parameters such as MTF, PSF, and Strehl ratio should be included when evaluating optical performance after cataract surgery with multifocal IOL implantation.
We also measured objective pseudo-accommodation using the double-pass system. According to one previous study, the accommodation range measured by the double-pass system decreased with increased age.33 The accommodation range obtained from participants aged 46-55 years was 0.73±0.33 D, less than the average accommodation range of multifocal IOL implanted eyes, 1.55±0.64 D (Table 1). This result could demonstrate the near vision improvement of multifocal IOLs. Thus, we confirmed that multifocal IOLs could be used as a treatment option for the correction of presbyopia. In our study, we did not perform the functional analysis of bilateral implantation of multifocal IOLs. According to one study comparing the binocular function between unilateral and bilateral implantation of multifocal IOLs, bilateral implantation of multifocal IOL appeared to contribute to better stereopsis.34 Thus, it will be worthy to measure and compare the optical quality parameters between unilateral and bilateral implantation of multifocal IOLs using the proper test device to evaluate both conditions.
The ray-tracing aberrometer was used in this study because it has the advantage of the larger dynamic range for measurement of accommodation compared with the Hartmann-Shack principle-based aberrometer.35 It is well known that, in a patient's eye with a mild to severe amount of scatter, the Hartmann-Shack wavefront sensors might overestimate image quality.14 According to our results, MTF10 obtained from the ray-tracing aberrometer showed an overestimation in image quality, compared with the double-pass system. The aberrometer estimates optical quality from aberration only, whereas the double-pass system provides estimates of optical quality from both aberration and scattering. Therefore, the aberrometer might fail to provide a correct estimation of the optical quality in multifocal IOL implanted eyes.
The limitation of this study was the relatively small sample size, which can limit the generality of the results. For this reason, statistical significance might have occurred by chance. Therefore, prospective large sample size studies should be conducted to verity our results.
In conclusion, the Strehl ratio, one of the optical quality parameters obtained from the double-pass system, was correlated with total aberration in multifocal IOL implanted eyes. We also reported a larger objective pseudo-accommodation compared to the normal population. Further studies to provide an accurate technique for the measurements of ocular aberrations and other optical qualities in multifocal IOL implanted eyes are mandatory.
Figures and Tables
Table 2
Correlations between Parameters Obtained from the Double-Pass System (MTF Cutoff Frequency, Strehl Ratio, and Objective Pseudo-Accommodation) and Ocular Aberrations Obtained from the Ray-Tracing Aberrometer
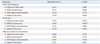
Table 3
Correlations between Parameters Obtained from the Double-Pass System (MTF Cutoff Frequency, Strehl Ratio, and Objective Pseudo-Accommodation) and Manifest Refraction Values or Subjective Visual Acuity
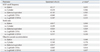
ACKNOWLEDGEMENTS
This work was partially supported by the National Research Foundation of Korea (NRF) grant funded by the Korea government (MEST No. 2013R1A1A2058907) and by the Converging Research Center Program through the Ministry of Science, ICT and Future Planning, Korea (2013K000365).
References
1. Keates RH, Pearce JL, Schneider RT. Clinical results of the multifocal lens. J Cataract Refract Surg. 1987; 13:557–560.


2. Holladay JT, Van Dijk H, Lang A, Portney V, Willis TR, Sun R, et al. Optical performance of multifocal intraocular lenses. J Cataract Refract Surg. 1990; 16:413–422.


3. Steinert RF, Aker BL, Trentacost DJ, Smith PJ, Tarantino N. A prospective comparative study of the AMO ARRAY zonal-progressive multifocal silicone intraocular lens and a monofocal intraocular lens. Ophthalmology. 1999; 106:1243–1255.


4. Montés-Micó R, Alió JL. Distance and near contrast sensitivity function after multifocal intraocular lens implantation. J Cataract Refract Surg. 2003; 29:703–711.


5. Leyland M, Zinicola E. Multifocal versus monofocal intraocular lenses in cataract surgery: a systematic review. Ophthalmology. 2003; 110:1789–1798.


6. Woodward MA, Randleman JB, Stulting RD. Dissatisfaction after multifocal intraocular lens implantation. J Cataract Refract Surg. 2009; 35:992–997.


7. Ortiz D, Alió JL, Bernabéu G, Pongo V. Optical performance of monofocal and multifocal intraocular lenses in the human eye. J Cataract Refract Surg. 2008; 34:755–762.


8. Moreno LJ, Piñero DP, Alió JL, Fimia A, Plaza AB. Double-pass system analysis of the visual outcomes and optical performance of an apodized diffractive multifocal intraocular lens. J Cataract Refract Surg. 2010; 36:2048–2055.


9. Santhiago MR, Wilson SE, Netto MV, Ghanen RC, Monteiro ML, Bechara SJ, et al. Modulation transfer function and optical quality after bilateral implantation of a +3.00 D versus a +4.00 D multifocal intraocular lens. J Cataract Refract Surg. 2012; 38:215–220.


10. Alfonso JF, Fernández-Vega L, Blázquez JI, Montés-Micó R. Visual function comparison of 2 aspheric multifocal intraocular lenses. J Cataract Refract Surg. 2012; 38:242–248.


11. Shin JY, Lee JB, Seo KY, Kim EK, Kim TI. Comparison of preoperative and postoperative ocular biometry in eyes with phakic intraocular lens implantations. Yonsei Med J. 2013; 54:1259–1265.


12. Applegate RA, Sarver EJ, Khemsara V. Are all aberrations equal. J Refract Surg. 2002; 18:S556–S562.


13. Nochez Y, Majzoub S, Pisella PJ. Effect of residual ocular spherical aberration on objective and subjective quality of vision in pseudophakic eyes. J Cataract Refract Surg. 2011; 37:1076–1081.


14. Díaz-Doutón F, Benito A, Pujol J, Arjona M, Güell JL, Artal P. Comparison of the retinal image quality with a Hartmann-Shack wavefront sensor and a double-pass instrument. Invest Ophthalmol Vis Sci. 2006; 47:1710–1716.


15. Vilaseca M, Arjona M, Pujol J, Issolio L, Güell JL. Optical quality of foldable monofocal intraocular lenses before and after injection: comparative evaluation using a double-pass system. J Cataract Refract Surg. 2009; 35:1415–1423.


16. Ondategui JC, Vilaseca M, Arjona M, Montasell A, Cardona G, Güell JL, et al. Optical quality after myopic photorefractive keratectomy and laser in situ keratomileusis: comparison using a double-pass system. J Cataract Refract Surg. 2012; 38:16–27.


17. Güell JL, Pujol J, Arjona M, Diaz-Douton F, Artal P. Optical Quality Analysis System; Instrument for objective clinical evaluation of ocular optical quality. J Cataract Refract Surg. 2004; 30:1598–1599.
18. Santamaría J, Artal P, Bescós J. Determination of the point-spread function of human eyes using a hybrid optical-digital method. J Opt Soc Am A. 1987; 4:1109–1114.


19. Saad A, Saab M, Gatinel D. Repeatability of measurements with a double-pass system. J Cataract Refract Surg. 2010; 36:28–33.


20. Benito A, Pérez GM, Mirabet S, Vilaseca M, Pujol J, Marín JM, et al. Objective optical assessment of tear-film quality dynamics in normal and mildly symptomatic dry eyes. J Cataract Refract Surg. 2011; 37:1481–1487.


21. Nochez Y, Majzoub S, Pisella PJ. Effect of interaction of macroaberrations and scattered light on objective quality of vision in pseudophakic eyes with aspheric monofocal intraocular lenses. J Cataract Refract Surg. 2012; 38:633–640.


22. Alió JL, Piñero DP, Plaza-Puche AB, Amparo F, Jiménez R, Rodríguez-Prats JL, et al. Visual and optical performance with two different diffractive multifocal intraocular lenses compared to a monofocal lens. J Refract Surg. 2011; 27:570–581.


23. Charman WN, Montés-Micó R, Radhakrishnan H. Problems in the measurement of wavefront aberration for eyes implanted with diffractive bifocal and multifocal intraocular lenses. J Refract Surg. 2008; 24:280–286.


24. Gatinel D. Limited accuracy of Hartmann-Shack wavefront sensing in eyes with diffractive multifocal IOLs. J Cataract Refract Surg. 2008; 34:528.


25. Jun I, Choi YJ, Kim EK, Seo KY, Kim TI. Internal spherical aberration by ray tracing-type aberrometry in multifocal pseudophakic eyes. Eye (Lond). 2012; 26:1243–1248.


26. de Castro A, Rosales P, Marcos S. Tilt and decentration of intraocular lenses in vivo from Purkinje and Scheimpflug imaging. Validation study. J Cataract Refract Surg. 2007; 33:418–429.


27. Artal P, Iglesias I, López-Gil N, Green DG. Double-pass measurements of the retinal-image quality with unequal entrance and exit pupil sizes and the reversibility of the eye's optical system. J Opt Soc Am A Opt Image Sci Vis. 1995; 12:2358–2366.


28. Artal P, Benito A, Pérez GM, Alcón E, De Casas A, Pujol J, et al. An objective scatter index based on double-pass retinal images of a point source to classify cataracts. PLoS One. 2011; 6:e16823.


29. Marcos S. Aberrations and visual performance following standard laser vision correction. J Refract Surg. 2001; 17:S596–S601.


30. Tuan KM, Chernyak D, Feldman ST. Predicting patients' night vision complaints with wavefront technology. Am J Ophthalmol. 2006; 141:1–6.


31. Gatinel D. Double pass-technique limitations for evaluation of optical performance after diffractive IOL implantation. J Cataract Refract Surg. 2011; 37:621–622.


32. McKelvie J, McArdle B, McGhee C. The influence of tilt, decentration, and pupil size on the higher-order aberration profile of aspheric intraocular lenses. Ophthalmology. 2011; 118:1724–1731.


33. Aldaba M, Vilaseca M, Arjona M, Pujol J. Age-related changes in accommodation measured with a double-pass system. Ophthalmic Physiol Opt. 2013; 33:508–515.

