Abstract
Purpose
This study was designed to measure time-dependent changes in muscle excursion and collagen content after tenotomy, and to analyze the correlation between muscle excursion and collagen content in a rabbit model.
Materials and Methods
Twenty-four rabbits underwent tenotomy of the second extensor digitorum longus (EDL) muscles on the right legs and were randomly assigned to three groups based on the period of time after tenotomy (2, 4, and 6 weeks). The second EDL muscles on left legs were used as controls. At each time after tenotomy, passive muscle excursion and collagen content, determined by hydroxyproline content, were measured bilaterally, and the ratio of each value to the normal one was used.
Results
The mean ratio of muscle excursion after tenotomy to the value of the control decreased in a time-dependent fashion: 92.5% at 2 weeks, 78.6% at 4 weeks, and 55.1% at 6 weeks. The mean ratio of hydroxyproline content in muscle to the value of the control increased in a time-dependent fashion: 119.5% at 2 weeks, 157.3% at 4 weeks, and 166.6% at 6 weeks. There was a significant negative correlation between the ratio of hydroxyproline content in muscle after tenotomy to the control values and the ratio of muscle excursion after tenotomy to the control values (r=-0.602, p=0.002).
Tendon rupture is one of the more common injuries seen in clinical practice as a result of trauma or degenerative disease.1 After tendon rupture, the connecting muscle contracts, and muscle contracture occurs with delay in repair. Loss of normal resting tension inside the muscle decreases muscle mass, contractile function, and the number of capillaries and sarcomeres.2,3 Increases are seen in necrosis of muscle fibers and the amount of connective tissue in muscle.2 These muscle changes seem to affect the clinical outcome of tendon repair. An understanding of pathologic muscle changes after tendon rupture will influence both the timing and technique of surgical treatment (i.e., direct repair, grafting, or tendon transfer).
According to an animal study using rabbit extensor digitorum longus (EDL) tendons, maximal muscle tension decreased over time after tenotomy.4 In another study using rabbit soleus muscles, muscle excursion continued to decrease significantly over time after tenotomy and was mainly observed during the first 4 weeks.5 The recovery of muscle excursion was independently influenced by the timing of tendon repair after tenotomy and the muscle excursion at the time of the repair.5
Muscle fibers, the basic structural elements of skeletal muscle, are organized histologically by the surrounding connective tissues (endomysium, perimysium, and epimysium), which bind the contractile units together to provide integrated motion.6 In a study using rat muscles, collagen networks in the endomysium and perimysium increased significantly after tenotomy.2 Increases in the collagen content within muscles after tenotomy or tendon rupture seem to be related to the inability of muscles to recover following a delay in tendon repair.
In this study, we hypothesized that the collagen content measured at each time point after tenotomy would correlate with the passive excursion of the muscle. The purpose of this study was to measure the time-dependent changes in muscle excursion and the collagen content after tenotomy, and to determine how these measures correlate with each other in a rabbit model.
All experimental methods were approved by the Institutional Animal Care and Use Committee. Twenty-four male New Zealand white rabbits were used for the measurements of passive muscle excursion and collagen content at each time point after tenotomy (control, and 2, 4, and 6 weeks after tenotomy).
The EDL muscle of the second toe was chosen as the experimental muscle because it has a long and large tendon that can be easily manipulated surgically. This muscle is easy to define because all muscle fibers of the muscle of the second toe originate from the medial surface of the tibia.7 The EDL is also an intrasynovial tendon like the human finger flexor and extensor tendons that are commonly injured.
Twenty-four animals were randomly assigned to one of three groups that were defined based on the time after tenotomy (2 weeks, 4 weeks, or 6 weeks). The operations in each animal were done on the right legs, and left legs were used as the controls.
Anesthesia was induced with an intramuscular injection of 15 mg/kg of Zoletil 50 (Zoletil 50 mg/mL, Tiletamine 125 mg, and Zolazepam 125 mg; Virbac, Carros, France) and 5 mg/kg of Rompun (xylazine hydrochloride 23.32 mg/mL; Bayer Korea, Seoul, South Korea) and was maintained with enflurane, which provided approximately 30 min of adequate sedation.
All operative procedures were performed by one hand surgeon (YRC). The right hind limbs were shaved, and animals were positioned supine on the operating table with the knee in approximately 90° of flexion and the hip in flexion and external rotation. Under aseptic conditions, a 1.5 cm longitudinal skin incision was made over the medial side of the distal tibia, and the tendon of the second toe extensor digitorum muscle was exposed. The tendon was transected. The wound was closed, and all animals were returned to their cages. Muscle excursion at 2, 4, and 6 weeks after tenotomy was measured. The left hind limbs were used as controls. On each rabbit, the proximal tendon stump of the muscle was completely released from the adhesions with the surrounding tissues before the measurement of muscle excursion. Muscle excursion was measured by the distance that the tenotomized proximal tendon end moved from the retracted position to the point of maximal pull of the tendon with a hemostat using a digital caliper. The pulling force applied by the operating surgeon was maximally set at the point where no apparent plastic deformation occurred by stretching of the tendon.
Animals were sacrificed with pentobarbital at three different time points: 2, 4, and 6 weeks after tenotomy. The second toe extensor digitorum muscles were harvested from bilateral lower limbs (the muscle after tenotomy and the control muscle) after measuring the muscle excursion. Hydroxyproline, a major component of collagen, was quantified as a measure of the collagen content using the Hydroxyproline Colorimetric Assay Kit (BioVision, San Francisco, CA, USA). The standard curve was initially prepared. The hydroxyproline standard was diluted to 0.1 mg/mL by adding 10 µL of the 1 mg/mL standard to 90 µL of dH2O. Increasing volumes (0, 2, 4, 6, 8, and 10 µL) were added to a series of wells, and the overall volumes were adjusted to 50 µL/well with dH2O to generate 0, 0.2, 0.4, 0.6, 0.8, and 1.0 µg/well of the hydroxyproline standard. Muscle samples were homogenized in dH2O, using 100 µL H2O for every 10 mg of tissue. To a 100 µL sample of homogenate, 100 µL of concentrated HCl (-12 N) was added in a pressure-tight, Teflon-capped vial and hydrolyzed at 120℃ for 3 hr. A total of 10 µL of each hydrolyzed sample was transferred to a 96-well plate and evaporated to dryness under a vacuum. A total of 100 µL of the chloramine T reagent was added to each sample and standard, and incubated at room temperature for 5 min. Then, 100 µL of the p-dimethylaminobenzaldehyde reagent was added to each well and incubated for 90 min at 60℃. Finally, absorbance at 560 nm was measured in a VersaMax ELISA Microplate Reader (Molecular Devices, Sunnyvale, CA, USA).
The SAS version 9.2 (SAS Institute Inc., Cary, NC, USA) was used for all of the statistical analyses. The ratio of muscle excursion over hydroxyproline content was used on the control values to counter the individual differences. The muscle excursion ratio and hydroxyproline content ratio at each time point were compared with the linear mixed model. Post-hoc analysis was also performed to compare the two muscles' excursions and hydroxyproline content ratios at three combinations of time points: 2 weeks after tenotomy versus 4 weeks after tenotomy; 2 weeks after tenotomy versus 6 weeks after tenotomy; and, 4 weeks after tenotomy versus 6 weeks after tenotomy. Correlations between the muscle excursion ratio and the hydroxyproline ratio at each time point after tenotomy were also analyzed. For all analyses, the level of significance was p<0.05.
Mean passive muscle excursions after tenotomy were 3.7±0.8 mm at 2 weeks, 3.2±0.8 mm at 4 weeks, and 2.2±0.3 mm at 6 weeks. Whereas mean passive muscle excursions of controls were 4.0±0.9 mm, 4.2±1.5 mm, and 4.2±1.0 mm, respectively, the mean ratios of muscle excursion after tenotomy to the value of the controls were 92.5±11.9% at 2 weeks, 78.6±15.4% at 4 weeks, and 55.1±7.4% at 6 weeks. The decrease of the mean ratio of muscle excursion after tenotomy was significant between the groups at time points after tenotomy (p<0.001). Post-hoc analysis of the muscle excursion ratios after tenotomy showed a significant decrease in the muscle excursion ratio between 4 and 6 weeks after tenotomy (p<0.001) (Table 1, Fig. 1).
Hydroxyproline content in the muscle was measured in order to quantify the collagen content. Hydroxyproline content in the muscle after tenotomy was 0.50±0.08 ng/uL at 2 weeks, 1.66±0.45 ng/uL at 4 weeks, and 1.04±0.47 ng/uL at 6 weeks. Whereas hydroxyproline content in the muscle of controls was 0.42±0.06 ng/uL, 1.09±0.33 ng/uL, and 0.64±0.32 ng/uL, respectively, the mean ratios of hydroxyproline content in muscle after tenotomy to the value of the controls were 119.5±9.7% at 2 weeks, 157.3±47.7% at 4 weeks, and 166.6±25.2% at 6 weeks. The decrease in the mean ratio of hydroxyproline content in the muscle after tenotomy was significant between the groups at three time points after tenotomy (p<0.001). Post-hoc analysis of the hydroxyproline content ratios in muscle after tenotomy showed a significant increase of collagen content ratio between 2 and 4 weeks after tenotomy (p=0.039) and between 2 and 6 weeks after tenotomy (p<0.001) (Table 2, Fig. 2).
There was a significant negative correlation between the ratio of hydroxyproline content in the muscle after tenotomy to the control value and the ratio of the muscle excursion after tenotomy to the control values (r=-0.602, p=0.002). These findings suggest that the decrease in muscle excursion correlates with the increase in collagen content in the muscle over time following tenotomy.
In this study, the collagen content was measured at each time point after tenotomy and was found to correlate with the passive excursion of the muscle: the results showed that muscle excursion decreases significantly and the collagen content in muscle increases significantly as time passes after tenotomy. These two measurements at each time point after tenotomy correlated with each other.
There are numerous examples of tendon injuries in clinical situations, such as extensor or flexor tendon lacerations in the hand, rotator cuff injury, and rupture of the Achilles tendon. Once normal skeletal muscle loses its resting tension after tendon rupture, the muscle experiences a decrease in mass, contractile function, and number of capillaries and sarcomeres.2,3 The muscle also demonstrates increases in muscle fiber necrosis and connective tissue content.2 These pathologic changes induce muscle contracture and are clinically evident by decreased passive muscle excursion. According to a study using rabbit soleus muscles, the recovery of muscle excursion after tendon repair was independently influenced by the timing of tendon repair after tenotomy and the muscle excursion at the time of repair.5 Józsa, et al.8 demonstrated that a delay in tendon repair greater than 6 weeks after tenotomy resulted in persistent muscle atrophy and intramuscular fibrosis and the inability to completely regenerate. In our study using rabbit second toe EDL muscles, the mean ratio of muscle excursion after tenotomy decreased significantly in a time-dependent manner after tenotomy, especially between 4 and 6 weeks after tenotomy (p<0.001). Our results differed from those of Jeon, et al.5 who reported a decrease in soleus muscle excursion after tenotomy that occurred mostly during the first 4 weeks. Although both the second toe EDL muscle and the soleus muscle have unipennate muscle fiber architecture, the soleus muscle has a higher pennation angle than the EDL muscle and is fused for much of its length with the gastrocnemius muscle.9 However, it is clear that the passive muscle excursion significantly decreases in a time-dependent fashion after tenotomy or tendon rupture.
Skeletal muscle fibers are organized by the surrounding connective tissues in a three-level organization: the endomysium surrounds individual fibers; the perimysium binds muscle fibers into fascicles and; the epimysium (fascia) surrounds the whole muscle.2 Because tenotomy is known to cause a marked increase in the amount of intramuscular connective tissue (especially in the endomysial and perimysial collagen networks in a rat tenotomy model),2 time-dependent changes in the collagen content after tenotomy were measured. In this study, significant time-dependent increases in the collagen content after tenotomy were demonstrated, especially between 2 and 4 weeks after tenotomy (p=0.039). Increase in the collagen content probably occurred in the endomysium and the epimysium. This result seems to explain why the release of adhesions around the epimysium (fascia) does not affect the restoration of muscle excursion at the time of delayed tendon repair in clinical situations.
Post-hoc analysis showed that collagen content significantly increased between 2 and 4 weeks after tenotomy (p=0.039), while muscle excursion significantly decreased between 4 and 6 weeks after tenotomy (p<0.001). It might mean that the increase of collagen content in turn induces the decrease of muscle excursion after tenotomy.
In the correlation analysis between decreased ratio of passive muscle excursion and increased ratio of collagen content in a time-dependent fashion to the values of the controls, there was a significant negative correlation (r=-0.602, p=0.002), indicating that the time-dependent decrease in muscle excursion significantly correlates with the increase in collagen content in muscle after tenotomy. According to Jeon, et al.,5 the amount of recovery of muscle excursion after tendon repair is independent of the timing of repair and the muscle excursion observed during repair. Because the decrease in muscle excursion significantly correlated with the increase in collagen content in muscle in our study, the recovery of muscle excursion after tendon repair might depend on the collagen content in the muscle at the time of tendon repair.
This study has some limitations. First, the goal was to identify time-dependent changes in passive muscle excursion and collagen content in muscle. However, these changes were compared in three different groups of adult rabbits because it is impossible to measure these changes in the same individual. The ratios of these measures were compared to the normal values of the contralateral sides, and the linear mixed model was used to analyze the time-dependent changes in the ratios of the measurements. Second, we did not measure the muscle mass when harvesting the tenotomized muscle. Muscle atrophy following tenotomy could affect the ratio of collagen content; however, the previous morphometric study using a rabbit soleus muscle post-tenotomy showed marked increase of connective tissue in both the endomysial and the perimysial layers. Furthermore, our study has been focused on the relationship between muscle excursion and collagen content following tenotomy. Additionally, the number of sarcomeres in the muscles was not measured. According to a previous study, muscles decrease in sarcomere number after tenotomy in order to increase sarcomere length and restore contractile function.10 The correlations of recovery of muscle excursion after tendon repair and the collagen content at the time of tendon repair were not measured. This information warrants further study.
In conclusion, the passive muscle excursion after tenotomy decreased, while the collagen content in muscle after tenotomy increased in a time-dependent fashion. The decrease in the passive muscle excursion after tenotomy significantly correlated with the increase in the collagen content in muscle after tenotomy. Because muscle recovery depends on the muscle excursion at the time of tendon repair,5 suppression of collagen network formation in the muscle could be a therapeutic target to increase the muscle excursion in cases of delayed tendon repair.
Figures and Tables
Fig. 1
Time-dependent change in the ratio of passive muscle excursion to normal value (%) following tenotomy of the second extensor digitorum longus muscle.
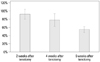
Fig. 2
Time-dependent changes in the ratios of hydroxyproline (collagen) content to the normal value (%) following tenotomy of the second extensor digitorum longus muscle.
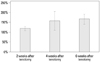
ACKNOWLEDGEMENTS
This research was supported by Basic Science Research Program through the National Research Foundation of Korea (NRF) funded by the Ministry of Science, ICT & Future Planning (grant number: 2011-0014399).
References
1. Jamali AA, Afshar P, Abrams RA, Lieber RL. Skeletal muscle response to tenotomy. Muscle Nerve. 2000; 23:851–862.


2. Józsa L, Kannus P, Thöring J, Reffy A, Järvinen M, Kvist M. The effect of tenotomy and immobilisation on intramuscular connective tissue. A morphometric and microscopic study in rat calf muscles. J Bone Joint Surg Br. 1990; 72:293–297.
3. Baker JH, Hall-Craggs EC. Changes in sarcomere length following tenotomy in the rat. Muscle Nerve. 1980; 3:413–416.


4. Tsai AM, Jamali AA, Abrams RA, Lieber RL. Skeletal muscle recovery following tenotomy and simulated seven day delayed tendon repair. Trans Orthop Res Soc. 1998; 23:663.
5. Jeon SH, Chung MS, Baek GH, Lee YH, Gong HS. The effect of muscle excursion on muscle recovery after tendon repair in a neglected tendon injury: a study in rabbit soleus muscles. J Orthop Res. 2011; 29:74–78.


6. Lieber RL, Fridén J. Functional and clinical significance of skeletal muscle architecture. Muscle Nerve. 2000; 23:1647–1666.


7. Takahashi M, Ward SR, Marchuk LL, Frank CB, Lieber RL. Asynchronous muscle and tendon adaptation after surgical tensioning procedures. J Bone Joint Surg Am. 2010; 92:664–674.


8. Józsa L, Bálint BJ, Vándor E, Réffy A, Demel Z. Recapillarization of tenotomized skeletal muscles after delayed tendon suture I Experimental study. Res Exp Med (Berl). 1985; 185:163–168.

