Abstract
Purpose
The purpose of this study was to investigate the effects of unilateral pedicle screw fixation on the fusion segment and the superior adjacent segment after one segment lumbar fusion using validated finite element models.
Materials and Methods
Four L3-4 fusion models were simulated according to the extent of decompression and the method of pedicle screws fixation in L3-4 lumbar fusion. These models included hemi-laminectomy with bilateral pedicle screw fixation in the L3-4 segment (BF-HL model), total laminectomy with bilateral pedicle screw fixation (BF-TL model), hemi-laminectomy with unilateral pedicle screw fixation (UF-HL model), and total laminectomy with unilateral pedicle screw fixation (UF-TL model). In each scenario, intradiscal pressures, annulus stress, and range of motion at the L2-3 and L3-4 segments were analyzed under flexion, extension, lateral bending, and torsional moments.
Results
Under four pure moments, the unilateral fixation leads to a reduction in increment of range of motion at the adjacent segment, but larger motions were noted at the fusion segment (L3-4) in the unilateral fixation (UF-HL and UF-TL) models when compared to bilateral fixation. The maximal von Mises stress showed similar patterns to range of motion at both superior adjacent L2-3 segments and fusion segment.
Conclusion
The current study suggests that unilateral pedicle screw fixation seems to be unable to afford sufficient biomechanical stability in case of bilateral total laminectomy. Conversely, in the case of hemi-laminectomy, unilateral fixation could be an alternative option, which also has potential benefit to reduce the stress of the adjacent segment.
Because pedicle screw system has an advantage for initial stability and increase fusion rates, instrumented posterior lumbar fusion surgery is widely used for the treatment of degenerative lumbar disease.1,2 However, even though many previous studies have reported excellent surgical results with instrumented lumbar fusion for degenerative lumbar disease,1,3,4 the robust stiffness of pedicle screws and rods might lead to an increase in the stress concentration at the adjacent segment after fusion, which could be a candidate risk factor for adjacent segment degeneration (ASD).5,6,7,8
Several clinical studies have demonstrated that unilateral pedicle screw fixation for one- or two-level fusion produced similar fusion rates and clinical results to the bilateral pedicle screw fixation system,9,10,11,12,13 though there have been conflicting biomechanical results.14,15 Unilateral instrumentation has advantages over bilateral fixation in terms of reduced operation time, medical expenses, blood loss, and hospital stay duration.10,11 Furthermore, unilateral pedicle screw fixation can lead to a decrease in fusion segment stiffness and stress of facet joint at superior adjacent segment.5,16 From this perspective, unilateral pedicle screw fixation would have a positive effect on stress concentration at the adjacent segment after lumbar fusion surgery, which seems to be a potential benefit of unilateral fixation.
However, it should be acknowledged that the postoperative stability after spine surgery depends on the extent of decompression such as laminectomy and facetectomy.17,18 Accordingly, the extent of decompression should be considered when predicting postoperative stability following supine surgery. Nevertheless, there has been no study to consider simultaneously both the fixation method and the extent of decompression. Therefore, the purpose of the current study was to investigate and compare biomechanical stress at both the fusion segment and the superior adjacent segment with different extents of decompression and fixation types, such as unilateral or bilateral pedicle screw fixation, using validated lumbar finite element (FE) models.
A three-dimensional (3D) nonlinear FE model of the lumbar spine was developed which consisted of three lumbar vertebrae, two intervertebral discs, and the associated spinal ligaments. Geometrical details of the human lumbar spine (L2-4) were obtained from high-resolution computed tomography (CT) images of a 46-year-old male subject who had no spinal deformities. Digital CT data were imported into a software program (Mimics; Materialise Inc., Leuven, Belgium) and used to generate a 3D geometrical surface of the lumbar spine. Exported IGES files from the Mimics software were input into Unigraphics NX 3.0 (Siemens PLM Software, Torrance, CA, USA) to form solid models for each vertebral segment. The solid model was then imported into Hypermesh 8.0 (Altair Engineering, Inc., Troy, MI, USA) to generate FE meshes. The FE method was analyzed with commercially available software (ABAQUS 6.6-1; Hibbitt, Karlsson and Sorenson, Inc., Providence, RI, USA).16,19
3D homogenous and transversely isotropic solid elements were used to model the cortical and cancellous cores and the posterior bony parts of the vertebrae. The anterior longitudinal ligament, posterior longitudinal ligament, intertransverse ligament, ligament flavum, capsular ligament, interspinous ligament, and supraspinous ligament were modeled using tension-only truss elements.16,19
Material properties were selected based on various literature sources (Table 1).20,21,22,23,24 The cortical and cancellous regions of the vertebrae were modeled independently. Differentiating between cortical and trabecular bone in the posterior region was difficult; therefore, the posterior elements were all assigned a single set of material properties.
The annulus fibrosus (AF) was modeled as a composite of a solid matrix with embedded fibers (via the REBAR parameter) in concentric rings surrounding a nucleus pulposus (NP), which was considered to be an incompressible inviscid fluid. Element members with hybrid formulation (C3D8H) combined with a low elastic modulus and large Poisson ratio definitions were applied to simulate the NP. Eight-node brick elements were employed to model the matrix of the ground substance. Each of the four concentric rings of the ground substance contained two evenly spaced layers of annulus fibers oriented at ±30° to the horizontal plane. The reinforcement structure annulus fibers were represented by truss elements with modified tension-only elasticity. In the radial direction, four double cross-linked fiber layers were defined, and those fibers were bounded by the annulus ground substance and both endplates. In addition, these fibers proportionally decreased the elastic strength from the outermost (550 MPa) to the innermost (358 MPa) layer.16,19,25,26
The articulating facet joint surfaces were modeled using surface-to-surface contact elements in combination with the penalty algorithm with a normal contact stiffness of 200 N/mm and a friction coefficient of zero. The thickness of the cartilage layer of the facet joint was assumed to be 0.2 mm. The initial gap between the cartilage layers was assumed to be 0.5 mm. The cartilage was assumed to be isotropic: a linear elastic with a Young's modulus of 35 MPa and a Poisson's ratio of 0.4.27 Spinal ligaments were represented with nonlinear material properties. Naturally-occurring changes in ligament stiffness (initially low stiffness at low strains, followed by increasing stiffness at higher strains) were simulated through a "hypoelastic" material designation (Table 1). 3D truss elements were used to simulate ligaments, which were active only upon tension.16,19
Two kinds of decompression states were simulated. One was hemi-laminectomy at the L3-4 segment, for which the right lamina was totally removed at the isthmic portion. The other was total (bilateral) laminectomy. In order to simulate the total laminectomy, both sides of the lamina were removed at the isthmic portion. In both models, a supraspinous ligament and interspinous ligament between the L3 and L4 spinous processes were removed along with a part of the L3 and L4 spinous processes, and the ligamentum flavum of L3-4 was also removed depending on the extent of the laminectomy. All models had continuity of the proximal posterior ligament complex between the L2 spinous process and the remaining L3 spinous process (Fig. 1).
A posterior pedicle screw fixation was simulated in the L3-4 intertransverse process fusion. All screws had sharp threads to prevent relative motion at the bone-screw interface and were inserted into the anterior third of the vertebral body through the pedicles. The pedicle screws did not violate the medial wall of the pedicle or the endplates. With the exception of the screw tip, the remaining surface of the screw was fixed to the bone without allowing relative motion. A "tie" contact condition was used to enable the screw threads and vertebrae to be permanently bonded together by full constraint. The diameters of all pedicle screws were assumed to be 5.0 mm, with a mean outer diameter of 6.5 mm (including thread height). The lengths of the screws in the L3 and L4 were 40 mm (Fig. 2). For the simulation of unilateral pedicle screw fixation, the pedicle screw-rod system was applied only at the right side, while the pedicle screw-rod system was applied to both sides for the bilateral pedicle screw fixation simulation.16
Four scenarios were simulated in total according to the extent of decompression or pedicle screw fixation. The BF-HL model represented hemi-laminectomy with bilateral pedicle screw fixation in the L3-4 segment, and the BF-TL model represented total laminectomy with bilateral pedicle screw fixation. Furthermore, in the UF-HL model, hemi-laminectomy was simulated with unilateral pedicle screw fixation and total laminectomy was simulated with unilateral pedicle screw fixation in the UF-TL model (Fig. 1).
This FE investigation included two types of loading conditions corresponding to 1) loads used in the experimental part of the studies28,29,30 for model validation and 2) model predictions for clinically relevant loading scenarios. For model validation, the experimental and simulated loading protocols were identical. For four pure moments, the results of range of motion were compared to those of previous in vitro studies by Renner, et al.28 and Wilke, et al.30 The nodes of the inferior surfaces of the most inferior vertebral body were completely fixed in all directions. To validate the model for pure moments, we applied the same loading conditions as Renner, et al.28 and Wilke, et al.,30 respectively. Nodes on top of the L2 vertebra were defined as coupling nodes. A reference node was created and connected to all coupling nodes. A coupling element was created to distribute moments on the reference node. For comparison with the results of study by Renner, et al.,28 8 Nm in flexion and 6 Nm in extension were imposed on the L2 vertebral body without preload and with an 800 N follower preload. ±4 Nm in torsion and ±6 Nm in lateral bending moments were imposed on the L2 vertebral body without preload. For comparison with the results of study by Wilke, et al.,30 7.5 Nm were imposed on the L2 vertebral body in flexion, extension, lateral bending, and torsion moment, respectively. Furthermore, the model was also validated for using intradiscal pressure. The intradiscal pressure of the current model was compared with the results in the experimental study by Schilling, et al.29 The experimental and simulated loading protocols were identical.
For model prediction for clinically relevant scenarios, the loading condition followed the hybrid testing protocol implemented during the flexibility testing of the FE models as previously described for the study of adjacent level biomechanics.31 The follower load technique was used to simulate the vector sum of the trunk muscle co-activation using a single internal force vector acting tangent to the curvature of the spine passing through each segmental center of rotation.32 The 400 N compressive follower load was simulated at each motion segment in the model by a pair of 2-node thermo-isotropic truss elements. The trusses were attached bilaterally to the cortical shell of the vertebrae at each motion segment. Each truss spanned the disc space passing through the instantaneous center of rotation at each motion segment.28 The hybrid testing protocol involved the application of the pure moment to the intact and fusion models until the L2-4 rotation (displacement) equaled the intact load control case values, which was achieved by imposing 7.5 Nm flexion, extension, lateral bending and torsion moments on the L2 vertebral body in the intact model.
For model validation, the experimental and simulated loading protocols were identical. For four pure moments, the results of range of motion were compared to those of previous in vitro studies by Renner, et al.28 and Wilke, et al.30 (Fig. 2A and B). For the intradiscal pressure, the results were compared to those in the study by Schilling, et al.29 (Fig. 2C). We performed a mesh convergence test with spinal segment (L2-4). As critical result parameters, mesh densities about range of motion were selected to test for difference among the intact models. The finest mesh density was chosen since the change was within 1.8% (<0.2°). Finally, the intact spine model was converged using 52955 nodes and 47213 elements.
The range of motion (ROM) at each corresponding level was compared among the 4 fusion models. The change of ROM at the adjacent segment was described as the percent change from the intact model in each moment under a hybrid protocol, and the change of ROM at the fusion segment was described as the percent of remaining ROM (100-percent change) from the intact model in each moment under a hybrid protocol (Figs. 3 and 4).
Under flexion moment, the ROM at the adjacent segment increased by 15.4%, 15.4%, 7.69%, 7.69% in the BF-HL, BF-TL, UF-HL, and UF-TL models, respectively, compared to the intact model (Fig. 3). Under extension moment, the ROM at the adjacent segment increased by 16.7%, 16.7%, 8.3%, 8.3% in the BF-HL, BF-TL, UF-HL, and UF-TL models, respectively, compared to the intact model. Therefore, under flexion and extension moments, the unilateral fixation leads to about a 50% reduction in the ROM increase at the adjacent segment after fusion when compared to bilateral fixation. Under torsional moments, the ROM at the L2-3 segment increased by 14.9%, 14.9%, 9.9%, and 4.9% in the BF-HL, BF-TL, UF-HL, and UF-TL models, respectively, compared to the intact model. Under lateral bending moments, the ROM at the adjacent segment increased by 6.25%, 6.25%, 4.17%, and 2.08% in the BF-HL, BF-TL, UF-HL, and UF-TL models, respectively, compared to the intact model (Fig. 3). Therefore, the extent of decompression influenced the percent increment only in the unilateral fixation models and only under torsion and lateral bending.
Relatively larger motions were noted at the fusion segment (L3-4) in the unilateral fixation (UF-HL and UF-TL) models (Fig. 4). Under flexion moments, the remaining range of motion was 8.9%, 8.9%, 32.1%, and 32.1% in the BF-HL, BF-TL, UF-HL, and UF-TL models, respectively, compared to the intact model. Under extension moments, 16.0% motion remained in the BF-HL and BF-TL models, as compared to the intact model. However, 47.8% motion remained in the UF-HL and UF-TL models. Under torsion moments, bilateral fixation models (BF-HL and BF-TL) showed 50.5% remaining motion at the fusion segment, while 61.1% and 77.7% range of motion remained at the fusion segment in the UF-HL and UF-TL models, respectively. Under lateral bending, the remaining range of motion was 26.8%, 26.8%, 31.7%, and 34.1% in the BF-HL, BF-TL, UF-HL, and UF-TL models, respectively, compared to the intact model (Fig. 4).
The four models showed increased maximal von Mises stress on the AF at superior adjacent L2-3 segments under the four moments, similar to the increases in ROM. The bilateral pedicle screw models, BF-HL and BF-TL, experienced the largest increase in AF stress at the adjacent L2-3 segments under all four moments. Compared to the intact model, both the BF-HL and BF-TL models yielded increases in AF stress of 34.1%, 125.0%, 84.2%, and 87.5% at the L2-3 segment under moments of flexion, extension, torsion, and lateral bending, respectively (Fig. 5). Following the bilateral pedicle screw fixation models, both the UF-HL and UF-TL models demonstrated 23.1% and 112.5% increases in AF stress under flexion and extension moments, respectively. Furthermore, under torsion and lateral bending moments, the unilateral fixation models demonstrated different increments in AF stress related to the extent of decompression. The UF-HL and the UF-TL model demonstrated 71.2% and 57.9% increases in AF stress, respectively, under torsion moments. Under lateral moments, the UF-HL and the UF-TL model showed 50.0% and 37.5% increases, respectively. Generally, unilateral fixation models led to a prominent reduction of increased AF stress at the adjacent L2-3 segment under the four moments (Fig. 5).
The current study clearly showed that the extent of decompression had a critical role in the postoperative stability following unilateral instrumented lumbar fusion surgery, even though the majority of clinical studies on unilateral pedicle screw fixation have demonstrated satisfactory and nearly identical outcomes and fusion rates compared with bilateral fixation.9,10,13 Furthermore, this study suggests that the decrease in fusion segment stiffness following unilateral pedicle screw fixation could potentially be an advantage of reducing the stress concentration at the adjacent segment following lumbar fusion surgery, which is consistent with previous findings.33
In the UF-HL and UF-TL models, which represented unilateral pedicle screw fixation, the remaining ROM at the fusion level increased under flexion, extension, torsion, and lateral bending moments, compared with bilateral fixation models. The UF-TL model yielded the largest remaining motion, where 30% to 50% of motion remained under flexion, extension, lateral bending moments, compared to the intact model. Under torsion moments, about 80% of motion remained in the UF-TL model. These findings can be interpreted as being a result of the inadequate stability of the UF-TL model, and suggest that bilateral, wide or extensive decompression combined with unilateral fixation which represented by the UF-TL would not have enough stability to accomplish solid lumbar fusion, in agreement with a previous biomechanical study regarding unilateral fixation, in which unilateral fixation allowed for an excessive range of motion and produced off-axis movement.15 In contrast to the UF-TL model, the UF-HL model demonstrated comparable stability to the bilateral fixation models under torsion moment. Therefore, these findings mean that the initial stability of unilateral fixation for lumbar fusion surgery depends on the extent of decompression.
The UF-TL model represented unilateral pedicle screw fixation combined with bilateral total laminectomy, and the findings obtained with this model demonstrated a vulnerability to torsional moments, with an increase in remaining motion at the fusion segment (78.8% compared to the intact model) under torsional moments, compared to both the UF-HL and the BF-TL models (61.1% and 50.6%, respectively). This instability under torsion moment was likely associated with the loss of bilateral facet joints. Because facet joints can protect the corresponding segment against torsion,34 total laminectomy with unilateral fixation (the UF-TL model) had detrimental effects on spinal stability under torsion. This may have clinical relevance in light of patient selection for unilateral instrumentation. If unilateral pedicle screw fixation can be warranted in combination with unilateral decompression surgery, this can be reasonable option for patients who have the unilateral lesion and consistent unilateral radiculopathy.
The present study also found that unilateral fixation has a beneficial effect on the adjacent segment. The ROM and annulus stress at the adjacent segment in both unilateral fixation models, the UF-HL and UF-BL models, were less than those at the matched segment in the both BF-HL and BF-TL models. This implies that the stress at the adjacent segment could be expected to be less concentrated than bilateral pedicle screw fixation in cases of fusion surgery using unilateral pedicle screw fixation. The possible mechanisms for the decrease in stress at the adjacent segment in the unilateral fixation models concern the decrease of facet joint stress at superior adjacent segment and the decrease of the stiffness of fusion segments,16,33 which would seem to affect the mechanical loading of adjacent segments. This is in agreement with previous results which showed that the absence of pedicle screws after the complete achievement of lumbar fusion can decrease the stress of the facet joint and disc at the adjacent segment.5,6,16 A recent retrospective study also showed that unilateral pedicle screw instrumentation leads to a lower rate of radiologic ASD and a better clinical outcome in a minimum 10-year follow-up.35 Therefore, unilateral fixation would appear to have yet another advantage over bilateral fixation in terms of alleviating stress concentration at the adjacent segment after fusion surgery.
It should be kept in mind that there are several shortcomings to the present study. First, the present models were simulated under single moments. Even though many biomechanical studies have been performed regarding this loading condition, there are different biomechanical behaviors related to loading conditions depending on single or repetitive loadings. Therefore, this study cannot guarantee that unilateral pedicle screws have similar strengths to bilateral pedicle screw fixation under cyclic repetitive loading conditions. Second, the current study simulated only posterolateral or intertransverse fusion surgeries. Posterior lumbar interbody fusion surgery is another popular procedure for lumbar fusion. However, considering that the posterior lumbar interbody fusion provides as much sound biomechanical stability as the posterolateral fusion,36 the current finding could be applicable to the posterior lumbar interbody fusion. Third, the current model simulated L3-4 fusion model for future consecutive related research even though most common fusion level is L4-5 in degenerative lumbar disease.
In conclusion, when bilateral total laminectomy is necessary, unilateral fixation would provide less stability than in case of unilateral laminectomy. Therefore, unilateral pedicle screw fixation can be considered in cases of limited unilateral decompression and fusion surgery. However, the reduced fusion segment stiffness would benefit the adjacent segment in terms of decreasing overstress at the adjacent segment after fusion surgery.
Figures and Tables
Fig. 1
FE models in the current study. (A) The BF-HL model. (B) The BF-TL model. (C) The UF-HL model. (D) The UF-TL model. The BF-HL model, bilateral fixation and hemilaminectomy model; The BF-TL model, bilateral fixation and total laminectomy model; The UF-HL model, unilateral fixation and hemilaminectomy model; The UF-TL model, unilateral fixation and total laminectomy model.
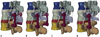
Fig. 3
The percent change of ROM at the adjacent segment (L2-3) from intact model under 4 pure moments. The BF-HL model, bilateral fixation and hemilaminectomy model; The BF-TL model, bilateral fixation and total laminectomy model; The UF-HL model, unilateral fixation and hemilaminectomy model; The UF-TL model, unilateral fixation and total laminectomy model; ROM, range of motion.

Fig. 4
The percent change of remaining ROM (100-percent change) at the fusion segment from the intact model under 4 pure moments. The BF-HL model, bilateral fixation and hemilaminectomy model; The BF-TL model, bilateral fixation and total laminectomy model; The UF-HL model, unilateral fixation and hemilaminectomy model; The UF-TL model, unilateral fixation and total laminectomy model; ROM, range of motion.
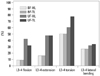
Fig. 5
Comparison of percent increment of AF stress at the adjacent segment (L2-3) from intact model among four models under 4 pure moments. AF, annulus fibrosus; The BF-HL model, bilateral fixation and hemilaminectomy model; The BF-TL model, bilateral fixation and total laminectomy model; The UF-HL model, unilateral fixation and hemilaminectomy model; The UF-TL model, unilateral fixation and total laminectomy model.
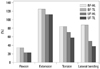
References
1. Bono CM, Lee CK. Critical analysis of trends in fusion for degenerative disc disease over the past 20 years: influence of technique on fusion rate and clinical outcome. Spine (Phila Pa 1976). 2004; 29:455–463.


2. Fischgrund JS, Mackay M, Herkowitz HN, Brower R, Montgomery DM, Kurz LT. 1997 Volvo Award winner in clinical studies. Degenerative lumbar spondylolisthesis with spinal stenosis: a prospective, randomized study comparing decompressive laminectomy and arthrodesis with and without spinal instrumentation. Spine (Phila Pa 1976). 1997; 22:2807–2812.
3. Martin CR, Gruszczynski AT, Braunsfurth HA, Fallatah SM, O'Neil J, Wai EK. The surgical management of degenerative lumbar spondylolisthesis: a systematic review. Spine (Phila Pa 1976). 2007; 32:1791–1798.
4. Hilibrand AS, Rand N. Degenerative lumbar stenosis: diagnosis and management. J Am Acad Orthop Surg. 1999; 7:239–249.


5. Kim HJ, Chun HJ, Moon SH, Kang KT, Kim HS, Park JO, et al. Analysis of biomechanical changes after removal of instrumentation in lumbar arthrodesis by finite element analysis. Med Biol Eng Comput. 2010; 48:703–709.


6. Kim HJ, Kang KT, Moon SH, Chun HJ, Kim HS, Park JO, et al. The quantitative assessment of risk factors to overstress at adjacent segments after lumbar fusion: removal of posterior ligaments and pedicle screws. Spine (Phila Pa 1976). 2011; 36:1367–1373.


7. Kim HJ, Moon SH, Chun HJ, Kang KT, Kim HS, Moon ES, et al. Comparison of mechanical motion profiles following instrumented fusion and non-instrumented fusion at the L4-5 segment. Clin Invest Med. 2009; 32:E64–E69.


8. Park P, Garton HJ, Gala VC, Hoff JT, McGillicuddy JE. Adjacent segment disease after lumbar or lumbosacral fusion: review of the literature. Spine (Phila Pa 1976). 2004; 29:1938–1944.


9. Kabins MB, Weinstein JN, Spratt KF, Found EM, Goel VK, Woody J, et al. Isolated L4-L5 fusions using the variable screw placement system: unilateral versus bilateral. J Spinal Disord. 1992; 5:39–49.
10. Suk KS, Lee HM, Kim NH, Ha JW. Unilateral versus bilateral pedicle screw fixation in lumbar spinal fusion. Spine (Phila Pa 1976). 2000; 25:1843–1847.


11. Xue H, Tu Y, Cai M. Comparison of unilateral versus bilateral instrumented transforaminal lumbar interbody fusion in degenerative lumbar diseases. Spine J. 2012; 12:209–215.


12. Beringer WF, Mobasser JP. Unilateral pedicle screw instrumentation for minimally invasive transforaminal lumbar interbody fusion. Neurosurg Focus. 2006; 20:E4.


13. Fernández-Fairen M, Sala P, Ramírez H, Gil J. A prospective randomized study of unilateral versus bilateral instrumented posterolateral lumbar fusion in degenerative spondylolisthesis. Spine (Phila Pa 1976). 2007; 32:395–401.


14. Yücesoy K, Yüksel KZ, Baek S, Sonntag VK, Crawford NR. Biomechanics of unilateral compared with bilateral lumbar pedicle screw fixation for stabilization of unilateral vertebral disease. J Neurosurg Spine. 2008; 8:44–51.


15. Slucky AV, Brodke DS, Bachus KN, Droge JA, Braun JT. Less invasive posterior fixation method following transforaminal lumbar interbody fusion: a biomechanical analysis. Spine J. 2006; 6:78–85.


16. Kim HJ, Chun HJ, Kang KT, Moon SH, Kim HS, Park JO, et al. The biomechanical effect of pedicle screws' insertion angle and position on the superior adjacent segment in 1 segment lumbar fusion. Spine (Phila Pa 1976). 2012; 37:1637–1644.


17. Abumi K, Panjabi MM, Kramer KM, Duranceau J, Oxland T, Crisco JJ. Biomechanical evaluation of lumbar spinal stability after graded facetectomies. Spine (Phila Pa 1976). 1990; 15:1142–1147.


18. Lee KK, Teo EC, Qiu TX, Yang K. Effect of facetectomy on lumbar spinal stability under sagittal plane loadings. Spine (Phila Pa 1976). 2004; 29:1624–1631.


19. Kim HJ, Chun HJ, Lee HM, Kang KT, Lee CK, Chang BS, et al. The biomechanical influence of the facet joint orientation and the facet tropism in the lumbar spine. Spine J. 2013; 13:1301–1308.


20. Chen CS, Cheng CK, Liu CL, Lo WH. Stress analysis of the disc adjacent to interbody fusion in lumbar spine. Med Eng Phys. 2001; 23:483–491.


21. Goel VK, Kim YE, Lim TH, Weinstein JN. An analytical investigation of the mechanics of spinal instrumentation. Spine (Phila Pa 1976). 1988; 13:1003–1011.


22. Pintar FA, Yoganandan N, Myers T, Elhagediab A, Sances A Jr. Biomechanical properties of human lumbar spine ligaments. J Biomech. 1992; 25:1351–1356.


23. Shirazi-Adl SA, Shrivastava SC, Ahmed AM. Stress analysis of the lumbar disc-body unit in compression A three-dimensional nonlinear finite element study. Spine (Phila Pa 1976). 1984; 9:120–134.


25. Shirazi-Adl A, Ahmed AM, Shrivastava SC. Mechanical response of a lumbar motion segment in axial torque alone and combined with compression. Spine (Phila Pa 1976). 1986; 11:914–927.


26. Polikeit A, Ferguson SJ, Nolte LP, Orr TE. Factors influencing stresses in the lumbar spine after the insertion of intervertebral cages: finite element analysis. Eur Spine J. 2003; 12:413–420.


27. Schmidt H, Galbusera F, Rohlmann A, Zander T, Wilke HJ. Effect of multilevel lumbar disc arthroplasty on spine kinematics and facet joint loads in flexion and extension: a finite element analysis. Eur Spine J. 2012; 21:Suppl 5. S663–S674.


28. Renner SM, Natarajan RN, Patwardhan AG, Havey RM, Voronov LI, Guo BY, et al. Novel model to analyze the effect of a large compressive follower pre-load on range of motions in a lumbar spine. J Biomech. 2007; 40:1326–1332.


29. Schilling C, Krüger S, Grupp TM, Duda GN, Blömer W, Rohlmann A. The effect of design parameters of dynamic pedicle screw systems on kinematics and load bearing: an in vitro study. Eur Spine J. 2011; 20:297–307.


30. Wilke HJ, Heuer F, Schmidt H. Prospective design delineation and subsequent in vitro evaluation of a new posterior dynamic stabilization system. Spine (Phila Pa 1976). 2009; 34:255–261.


31. Goel VK, Grauer JN, Patel TCh, Biyani A, Sairyo K, Vishnubhotla S, et al. Effects of charité artificial disc on the implanted and adjacent spinal segments mechanics using a hybrid testing protocol. Spine (Phila Pa 1976). 2005; 30:2755–2764.


32. Patwardhan AG, Havey RM, Meade KP, Lee B, Dunlap B. A follower load increases the load-carrying capacity of the lumbar spine in compression. Spine (Phila Pa 1976). 1999; 24:1003–1009.


33. Goel VK, Lim TH, Gwon J, Chen JY, Winterbottom JM, Park JB, et al. Effects of rigidity of an internal fixation device. A comprehensive biomechanical investigation. Spine (Phila Pa 1976). 1991; 16:3 Suppl. S155–S161.


34. Adams MA, Hutton WC. The effect of posture on the role of the apophysial joints in resisting intervertebral compressive forces. J Bone Joint Surg Br. 1980; 62:358–362.

