Abstract
Purpose
The aim of this work was to evaluate nuclear histone acetylation level and total histone acetyltransferase (HAT) and deacetylase (HDAC) activity in ejaculated sperm and their relevance to conventional sperm parameters.
Materials and Methods
Thirty-three normozoospermic men were included in this study. Semen samples were processed by swim-up and then immunostained by six acetylation antibodies (H3K9ac, H3K14ac, H4K5ac, H4K8ac, H4K12ac, and H4K16ac). Our preliminary study verified the expression of HAT/HDAC1 in mature human sperm. From vitrified-warmed sperm samples, total HAT/HDAC activity was measured by commercially available kits. Nuclear DNA integrity was also measured by TUNEL assay.
Results
The levels of six acetylation marks were not related with conventional sperm parameters including sperm DNA fragmentation index (DFI) as well as HAT/HDAC activity. However, sperm DFI was positively correlated with HAT activity (r=0.038 after adjustment, p<0.02). HAT activity showed a negative relationship with HDAC activity (r=-0.51, p<0.01). Strict morphology was negatively correlated with acetylation enzyme index (=HAT activity/HDAC activity) (r=-0.53, p<0.01).
Epigenetic mechanisms regulate the expression of DNA by activation or repression of transcriptional process. This form of gene regulation has now been implicated in mammalian gametogenesis or embryogenesis.1,2,3 Histone acetylation and DNA methylation has also been known to play an important role in spermatogenesis.4
Histone acetylation of lysine residues, clustered at the amino-terminal end of core histones, is regulated by a balance between histone acetyltransferases (HATs) to facilitate acetylation and histone deacetylases (HDACs) to decrease acetylation.5 When histones are hyperacetylated, its affinity to DNA is decreased by losing their positive charges, and the decreased affinity of hyperacetylated histones for DNA leads to a more relaxed chromatin structure that is more amenable to transcription, thus active transcription occurs.6 Generally, HATs act as a transcriptional coactivator and HDACs induce transcriptional repression.7,8
In a murine model, treatment with trichostatin A, an HDAC inhibitor, induces histone hyperacetylation and also inhibits normal spermatogenesis.9 This suggests that hypoacetylated state is important in the differentiation of spermatocytes within testes. Nonetheless, hyperacetylation of histone H4 is observed during the stage of spermatogonia in which active mitosis occurs.10
As sperm is matured during spermiogenesis, the majority of nuclear histones are substituted by protamine, thereby sperm nuclear DNA being more tightly compacted.11 Histone-to-protamine transition is initiated at the stage of elongating spermatids and the hyperacetylation of H4 could be observed again during this stage in a murine model.10 In human elongating spermatids, the positive H4 hyperacetylation signal was significantly lower in infertile men exhibiting normal spermatogenesis or those with impaired spermatogenesis.12 Impaired spermatogenesis has been reported to be associated with aberrant H4 acetylation.13 Thus, hyperacetylation of core histones appears to play an important role for correct histone-to-protamine exchange and complete differentiation of spermatids into mature sperm.
In a fully matured human sperm, approximately 15% of the DNAs are still found to histone, therefore, remaining histones have been proposed to carry essential marks for the establishment of epigenetic information in the offspring.14,15 Various studies suggested that retained histones bind specific regions and convey epigenetic information to the early embryo.16,17,18,19,20,21
There is some evidence that histones in the ejaculated human sperm are relatively more highly acetylated than previously expected, and sperm might possess active transcriptional activity.22,23,24 However, epigenetic modifications of ejaculated sperm have not been well understood in the area of male infertility and assisted reproductive technologies (ART). The expression of H4K5ac, H4K8ac, H4K12ac, and H4K16ac were identified in human testis samples from azoospermic men and ejaculated spermatozoa from fertile donors.22 A previous report denoted that testicular expressions of H3K9ac are similar between men with normal and impaired spermatogenesis.24
Currently, there have been no reports regarding the correlation of routine semen parameters with the histone acetylation state as well as the acetylation-associated enzyme activity in ejaculated human sperm. The aim of this work was to evaluate the sperm nuclear histone acetylation levels and the HAT/HDAC activity in ejaculated sperm from normozoospermic men and their relevance to conventional sperm parameters.
Thirty-three male partners participating IVF treatment during 2012 were recruited in this prospective study. We included the men who showed a total motile sperm count (TMC) ≥5 million for securing adequate amount of sperm to perform the assay. There were no exclusion criteria in the etiology of female infertility. All men were normozoospermic, and the conventional sperm parameters were presented in Table 1. An informed consent was obtained from all of the subjects, and the use of human semen for this study was approved by the Institutional Review Board of the Seoul National University Bundang Hospital (B-1112/141-005). All the male subjects had no history of genital inflammation or surgery. No subjective symptoms or self-reported medical risk factors were identified. Nobody had taken prescribed medications. Nineteen male partners were identified as smoker by self-report and/or urine cotinine measurement.
After avoiding coitus for at least 3 days, semen samples were obtained by masturbation at the time of oocyte pick-up. After liquefaction for 30 min at room temperature (RT), routine sperm quality was assessed by using computer-assisted semen analysis (SAIS-PLUS 10.1, Medical Supply Co., Seoul, Korea) and classified according to standard procedures of the World Health Organization (WHO, 2010). None had significant numbers of round cells or leukocytospermia as per WHO guidelines (<1 million/mL). Sperm morphology was evaluated according to the strict criteria proposed by Kruger/Tygerberg and adopted by the WHO. The semen was processed by conventional swim-up method and utilized for fertilization. The remaining sperm were used for the following experiments.
Nuclear DNA integrity was measured by the TUNEL assay as described before.25 The samples were smeared onto a silane-coated slide (DAKO, Glostrup, Denmark) and air-dried. Sperm were fixed with 4% neutral buffered formalin for 1 hr at 15-25℃, and then washed with phosphate buffered saline (PBS). Sperm were permeabilized with 0.1% Triton X-100 in 0.1% sodium citrate (Sigma, St. Louis, MO, USA). A commercial apoptosis detection kit (In Situ Cell Death Detection Kit; Roche Diagnostics GmbH, Mannheim, Germany) was used. The remaining procedures were performed as directed by the instructions in the kits. Counterstaining was performed using a mounting medium with 4',6-diamidino-2-phenylindole (DAPI; Vector Laboratories, Burlingame, CA, USA). The sperm with fragmented DNA had their nuclei stained in green, whereas the nuclei of the other cells were blue. Sperm heads with >50% of the area stained green were considered positive. At least 500 sperm were counted per experimental set and the percentage of sperm with fragmented DNA was determined as DNA fragmentation index (DFI).
Semen was washed with PBS three times and smears were prepared on glass slides. The smears were fixed in 4% paraformaldehyde for 20 min and air-dried. Smears were rehydrated with PBS twice and decondensed in 5 mM dithiotreitol and 0.3 µg/mL heparin for 30-60 min. Smears were permeabilized with PBS containing 0.5% Triton X-100 (PBS-T) for 10 min at RT and then washed twice with PBS. Smears were blocked with 3% bovine serum albumin (BSA) in PBS for 30 min at RT. After washing twice with PBS, the smears were incubated with the primary antibodies (1:100) for 2 hrs at RT. All of six primary antibodies (H3K9ac, H3K14ac, H4K5ac, H4K8ac, H4K12ac, and H4K16ac) were rabbit polyclonal antibodies purchased from Abcam (Cambridge, UK). Smears were then washed three times with PBS and incubated with fluorescein isothiocyanate-conjugated goat anti-rabbit antibody (1:100) for 1.5 hours at RT (Invitrogen, Carlsbad, CA, USA). After washing three times with PBS, smears were mounted with DAPI and observed under a Carl Zeiss Axiophot microscope (exciter filter BP450-490, emission filter BP520) at ×400 and ×1000 magnification. All sperm were stained with either 'weak' or 'strong' signal intensity (Fig. 1). We considered the strong signal as hypercetylated state. The signal intensity was judged by an independent investigator. On each slide, at least 400 sperm were counted, and the percentage of sperm exhibiting strong intensity was determined. Negative controls were performed in the absence of primary antibody.
A proportion of processed sperm was vitrified for the measurement of total HAT/HDAC activity. We performed a preliminary western blot assay from 5 men to verify the expression of HAT/HDAC in mature human sperm. For investigating the HAT/HDAC, polyclonal rabbit anti-HAT antibody (1:2000, orb128159; Biorbyt Ltd., Cambridge, UK) and polyclonal rabbit anti-HDAC1 antibody (1:1000, #2062; Cell Signaling Technology, Danvers, MA, USA) were used. A 50 kDa of HAT band and a 62 kDa of HDAC1 band were clearly identified in the mature human sperm lysate (Fig. 2). From the warmed semen samples, nuclear extracts were obtained using the Nuclear Extract kit (Active Motif, Carlsbad, CA, USA) according to the manufacturer's instructions. Pellets were re-suspended in 50 µL of Complete Lysis Buffer and centrifuged at 14000×g for 10 min at 4℃; supernatants were saved as the nuclear fractions. The protein concentration of each sample was measured (Bradford Bio-Rad Protein Assay kit, Bio Rad, San Diego, CA, USA), with BSA as a standard.
Total HAT activity was measured using a commercially available kit (Histone Acetyltransferase Activity Assay Kit, ab65352, Abcam) according to the detailed instructions provided by the manufacturer. Briefly, 30 µg of nuclear extracts was incubated with HAT substrates I/II and NADH-generating enzyme in HAT assay buffer for 4 hrs at 37℃. Absorbance was determined at 450 nm in an ELISA plate reader, with active nuclear extract used as a positive control and standard. The HAT activity was expressed as nmol/min/µg as indicated by the manufacturer. All experiments were performed in duplicate and the mean values were obtained.
Total HDAC activity was measured using the EpiQuik HDAC Activity/Inhibition Assay Kit (Epigentek, New York, USA). In brief, the 10 µg of sperm nuclear protein extract were incubated with a specific substrate for 1 hr at 37℃, followed by incubation with capture antibodies for 60 min and detection antibodies for 30 min at RT. Absorbance was determined using a microplate spectrophotometer at 450 nm. HDAC activity was calculated according to the manufacturer's instructions using the equation: activity (ng/h/mL)=[optical density (OD) (control-blank)-OD (sample-blank)]/slope×reaction time (0.5-1 hr)×sample dilution. All experiments were performed in duplicate and mean values were obtained. We arbitrary determined 'acetylation enzyme index' as HAT activity divided by HDAC activity.
Statistical analysis was performed using statistical software (MedCalc ver. 10.4, MedCalc Software, Mariakerke, Belgium). Data are always expressed as mean±SD. The Spearman correlation test was used to assess an association between different numerical parameters. Intergroup differences were assessed by nonparametric Wilcoxon test. A p-value of <0.05 (two-tailed) was considered statistically significant.
The mean immunopositivities and their ranges for six acetylation antibodies as well as HAT/HDAC activity are listed in Table 2, and the correlations between variable sperm parameters are presented in Table 3.
A univariate analysis revealed that each immunopositivity for six acetylation antibodies was not related with male age, sperm concentration, motility, TMC, strict morphology or sperm DFI. Moreover, each immunopositivity for six acetylation antibodies was not related with HAT or HDAC activity. HAT activity showed a negative relationship with HDAC activity (r=-0.51, p<0.01). Among the conventional sperm parameters, a negative correlation was observed between strict morphology and sperm DFI (r=-0.43, p<0.02).
Strict morphology was insignificantly correlated with HAT (r=-0.34, p=0.053) and HDAC (r=0.34, p=0.052) activity, but showed a negative correlation with acetylation enzyme index (r=-0.53, p<0.01). This relation still exists after adjusting sperm DFI (p<0.01) or after adjusting sperm DFI, HAT and HDAC activity (p<0.01) by multivariate analysis.
Sperm DFI was positively correlated with HAT activity (r=0.44, p<0.02), but not with HDAC activity. A multivariate analysis was performed to show a relation of sperm DFI with HAT or HDAC activity, and the relation was found still be maintained; sperm DFI=0.2 (HAT)-1.1 (HDAC)-32.1 (p<0.05).
Nineteen men were smokers (57.6%, 19/33), and the conventional semen parameters, six acetylation levels and HAT/HDAC activity were not different between smokers and non-smokers, except semen volume (3.0±1.2 mL vs. 3.8±1.2 mL, p=0.04).
For the first time, our work demonstrated a relationship between acetylation-associated enzyme activity and strict morphology or sperm DFI in ejaculated human sperm. Notably, a positive relationship between sperm DFI and HAT activity was found. Because HAT and HDAC activity had also a negative relationship, we performed a multivariate analysis, and the result showed that sperm DFI still had a statistically significant positive relationship with HAT activity after adjusting HDAC activity.
Increased sperm DNA damage has been reported to be associated with a late paternal effect during the activation of male genome,26 and sperm DFI has currently been implicated to be associated with IVF failure or miscarriage.27,28 Nonetheless, there have been no reports regarding the association of sperm DNA damage with sperm nuclear histone acetylation. Although we failed to show direct association between DNA damage and histone acetylation, we demonstrated a relationship between acetylation-associated enzyme activity and sperm DFI, implying some association between nuclear histone acetylation and DNA damage.
Approximately 15% of the DNA in ejaculated human sperm still bind to histone.15 When histones are hyperacetylated, its affinity to DNA is decreased by losing their positive charges, thus active transcription might occur. However, if histones are hyperacetylated, the histone-bound DNAs are less tightly compacted, thus being more susceptible to DNA damage. Our finding, a positive correlation between sperm DFI and HAT activity, strongly support this idea.
Nonetheless, it is unclear why each acetylation level at six acetylation residue sites examined in the present study was not correlated directly with the sperm DFI. Furthermore, each acetylation level at six acetylation residue sites was not related directly with HAT or HDAC activity. Possibly, other specific residue sites that were not examined in the present study might exist, therefore, further study is needed to explore the acetylation of specific regions to give rise to higher susceptibility to DNA damage. A global measure of acetylation would clarify this issue since cross-reactivity might occur between the six acetylation antibodies, and further studies are required.
In the present study, HAT activity showed a strong negative relationship with HDAC activity in the ejaculated human sperm. Therefore, we propose the 'acetylation enzyme index' as defined by HAT activity divided by HDAC activity; the higher this index, the more tendencies towards hyperacetylation would be expected in certain cells or tissues. Large variations in the acetylation enzyme index in this study might be due to a small number of study subjects. In the present study, the acetylation enzyme index was correlated only with strict morphology. Strict morphology in fact showed a negative relation with HAT activity and a positive relation with HDAC activity, but they did not reach statistical significance. A multivariate analysis also revealed a significantly negative relationship between acetylation enzyme index and strict morphology after adjusting for sperm DFI, HAT and HDAC activity. Hence, the acetylation enzyme index could serve as an independent predictor related with sperm morphology.
In the present study, we measured total activity of HAT and HDAC. Mammalian HDACs are classified into two major classes.29 Class I HDACs (HDACs 1, 2, 3, 8) are found exclusively in nucleus, whereas Class II HDACs (HDACs 4 to 7 and 9) are found in both nucleus and cytoplasm. Currently, there have been no reports whether specific sub-types of these enzymes exist in ejaculated human sperms, although it was previously reported that HDAC1 and HDAC6 are present in mouse testis.10
H3K9ac has been reported to be present in spermatocytes as well as in elongating spermatids during murine spermatogenesis.30 It was also demonstrated to be present in spermatogonia, spermatocytes, elongating spermatids, and even ejaculated sperm of fertile and infertile men.24 In that study, H3K9ac marks on retained histones are associated with specific regions of the sperm genome, defining an epigenetic code that may influence gene expression directly after fertilization. Although the exact expression levels were not described in that study, they did not find any differences in the H3K9ac expression pattern between normal and impaired spermatogenesis, or between ejaculated sperm from fertile donors and infertile patients.
Hecht, et al.22 investigated the expression of H4K5ac, H4K8ac, H4K12ac, and H4K16ac in human testis samples from azoospermic men undergoing biopsy and ejaculates from fertile donors. All of those antibodies revealed positive signals in round and elongating spermatids as well as ejaculated sperm. Human spermatogonia showed positive signals for H4K8ac and H4K16ac. In that report, the mean positivity in ejaculated sperm differed greatly between the four antibodies; 17.4% for H4K5ac, 14.4% for H4K8ac, 23.6% for H4K12ac, and 92% for H4K16ac. It is noted that the mean expression levels of H4K5ac (15.6%), H4K8ac (15.9%), and H4K12ac (15.4%) in our study were similar but H4K16ac positivity (18.1%) was much lower than those reported by Hecht, et al.22
The reason for such discrepancy is not clear. Hecht, et al.22 assessed H4 acetylation patterns in ejaculates from fertile donors, whereas our samples were obtained entirely from men participating in IVF treatment and after swim-up procedure, and all exhibited normozoospermic feature at routine semen analysis. The potential bias might occur within this cohort as a result of being recruited from an IVF setting. It is unclear whether different characteristics of study subjects or different sperm processing procedures lead to such discrepancy. Currently, we are in a process to measure sperm acetylation state and histone-protamine configuration before and after swim-up in both fertile donors and infertile males because normative data in human are still lacking.
Since male smokers are approximately 50% in our IVF clinics, such a high percent (57.6%) in our cohort is not surprising. Although our sample size of subjects was rather small, semen volume was significantly lower in smokers. The impact of smoking on female fecundity and reproduction has become apparent, but the relation between smoking and decreased male fertility is not conclusive.31 Nonetheless, a few studies reported that cigarette smoking is associated with a decrement of ejaculate volume, sperm concentration, motility, percentage of normal forms, and vitality.32,33,34
In IVF treatment, routine analysis for sperm characteristics has been known to be a poor predictive factor for fertilization, embryo development, and even implantation and miscarriage.15 Nonetheless, it has been understood that some cases of IVF failure appear to be due to paternal factors.35,36 Epigenetic modification of the ejaculated sperm has not been well understood from the viewpoint of male infertility and the area of ART. Further research is required to evaluate the clinical relevance of a sperm acetylation test. Further understanding of the transfer of epigenetic mechanisms from sperm to embryos will provide more insight into potential contribution of paternal factor.
Figures and Tables
Fig. 1
Representative microphotographs showing ejaculated sperm immnunostained by fluorescent antibody for histone H3K9, H3K14, H4K5, H4K8, H4K12, and H4K16 acetylation (×1000). Thick arrows indicate 'strong' intensity and thin arrows indicate 'weak' intensity; All sperm are stained with either 'weak' or 'strong' signal intensity, and immunopositivity was determined as the percentage of sperm showing 'strong' signal per total counted sperms. Negative controls were performed in the absence of primary antibody. Counterstaining was performed using a mounting medium with DAPI. FITC, fluorescein isothiocyanate.
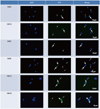
Fig. 2
Detection of histone acetyltransferase (HAT) and histone deacetylase 1 (HDAC1) in mature human sperm. Western blot assay demonstrates 50 kDa band of HAT and 62 kDa band of HDAC1 in mature human sperm lysates obtained from five men.

Table 2
Histone Acetylation Levels and Histone Acetyltransferase/Deacetylase Activity in Ejaculated Sperm from Enrolled Thirty Three Males
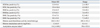
ACKNOWLEDGEMENTS
This work was supported by Grant No. 800-20110058 from the Seoul National University College of Medicine Research Fund.
References
1. Akiyama T, Nagata M, Aoki F. Inadequate histone deacetylation during oocyte meiosis causes aneuploidy and embryo death in mice. Proc Natl Acad Sci U S A. 2006; 103:7339–7344.


2. Klose RJ, Bird AP. Genomic DNA methylation: the mark and its mediators. Trends Biochem Sci. 2006; 31:89–97.


3. Ma P, Schultz RM. Histone deacetylase 1 (HDAC1) regulates histone acetylation, development, and gene expression in preimplantation mouse embryos. Dev Biol. 2008; 319:110–120.


4. Omisanjo OA, Biermann K, Hartmann S, Heukamp LC, Sonnack V, Hild A, et al. DNMT1 and HDAC1 gene expression in impaired spermatogenesis and testicular cancer. Histochem Cell Biol. 2007; 127:175–181.


5. Davie JR. Covalent modifications of histones: expression from chromatin templates. Curr Opin Genet Dev. 1998; 8:173–178.


7. de Ruijter AJ, van Gennip AH, Caron HN, Kemp S, van Kuilenburg AB. Histone deacetylases (HDACs): characterization of the classical HDAC family. Biochem J. 2003; 370(Pt 3):737–749.


8. Spencer VA, Davie JR. Role of covalent modifications of histones in regulating gene expression. Gene. 1999; 240:1–12.


9. Fenic I, Sonnack V, Failing K, Bergmann M, Steger K. In vivo effects of histone-deacetylase inhibitor trichostatin-A on murine spermatogenesis. J Androl. 2004; 25:811–818.


10. Hazzouri M, Pivot-Pajot C, Faure AK, Usson Y, Pelletier R, Sèle B, et al. Regulated hyperacetylation of core histones during mouse spermatogenesis: involvement of histone deacetylases. Eur J Cell Biol. 2000; 79:950–960.


11. Kistler WS, Henriksén K, Mali P, Parvinen M. Sequential expression of nucleoproteins during rat spermiogenesis. Exp Cell Res. 1996; 225:374–381.


12. Sonnack V, Failing K, Bergmann M, Steger K. Expression of hyperacetylated histone H4 during normal and impaired human spermatogenesis. Andrologia. 2002; 34:384–390.


13. Faure AK, Pivot-Pajot C, Kerjean A, Hazzouri M, Pelletier R, Péoc'h M, et al. Misregulation of histone acetylation in Sertoli cell-only syndrome and testicular cancer. Mol Hum Reprod. 2003; 9:757–763.


14. Gatewood JM, Cook GR, Balhorn R, Schmid CW, Bradbury EM. Isolation of four core histones from human sperm chromatin representing a minor subset of somatic histones. J Biol Chem. 1990; 265:20662–20666.


15. Steger K, Cavalcanti MC, Schuppe HC. Prognostic markers for competent human spermatozoa: fertilizing capacity and contribution to the embryo. Int J Androl. 2011; 34(6 Pt 1):513–527.


16. Dada R, Kumar M, Jesudasan R, Fernández JL, Gosálvez J, Agarwal A. Epigenetics and its role in male infertility. J Assist Reprod Genet. 2012; 29:213–223.


17. Emery BR, Carrell DT. The effect of epigenetic sperm abnormalities on early embryogenesis. Asian J Androl. 2006; 8:131–142.


18. Grunewald S, Paasch U, Glander HJ, Anderegg U. Mature human spermatozoa do not transcribe novel RNA. Andrologia. 2005; 37:69–71.


19. Hammoud SS, Nix DA, Zhang H, Purwar J, Carrell DT, Cairns BR. Distinctive chromatin in human sperm packages genes for embryo development. Nature. 2009; 460:473–478.


20. Krawetz SA. Paternal contribution: new insights and future challenges. Nat Rev Genet. 2005; 6:633–642.


21. Sutovsky P, Schatten G. Paternal contributions to the mammalian zygote: fertilization after sperm-egg fusion. Int Rev Cytol. 2000; 195:1–65.


22. Hecht N, Behr R, Hild A, Bergmann M, Weidner W, Steger K. The common marmoset (Callithrix jacchus) as a model for histone and protamine expression during human spermatogenesis. Hum Reprod. 2009; 24:536–545.


23. Rousseaux S, Reynoird N, Escoffier E, Thevenon J, Caron C, Khochbin S. Epigenetic reprogramming of the male genome during gametogenesis and in the zygote. Reprod Biomed Online. 2008; 16:492–503.


24. Steilmann C, Paradowska A, Bartkuhn M, Vieweg M, Schuppe HC, Bergmann M, et al. Presence of histone H3 acetylated at lysine 9 in male germ cells and its distribution pattern in the genome of human spermatozoa. Reprod Fertil Dev. 2011; 23:997–1011.


25. Jee BC, Suh CS, Shin MS, Lee HJ, Lee JH, Kim SH. Sperm nuclear DNA fragmentation and chromatin structure in one-day-old ejaculated sperm. Clin Exp Reprod Med. 2011; 38:82–86.


26. Tesarik J, Greco E, Mendoza C. Late, but not early, paternal effect on human embryo development is related to sperm DNA fragmentation. Hum Reprod. 2004; 19:611–615.


27. Robinson L, Gallos ID, Conner SJ, Rajkhowa M, Miller D, Lewis S, et al. The effect of sperm DNA fragmentation on miscarriage rates: a systematic review and meta-analysis. Hum Reprod. 2012; 27:2908–2917.


28. Sakkas D, Alvarez JG. Sperm DNA fragmentation: mechanisms of origin, impact on reproductive outcome, and analysis. Fertil Steril. 2010; 93:1027–1036.


29. Grozinger CM, Schreiber SL. Deacetylase enzymes: biological functions and the use of small-molecule inhibitors. Chem Biol. 2002; 9:3–16.
30. Delaval K, Govin J, Cerqueira F, Rousseaux S, Khochbin S, Feil R. Differential histone modifications mark mouse imprinting control regions during spermatogenesis. EMBO J. 2007; 26:720–729.


31. Practice Committee of the American Society for Reproductive Medicine. Smoking and infertility: a committee opinion. Fertil Steril. 2012; 98:1400–1406.
32. Künzle R, Mueller MD, Hänggi W, Birkhäuser MH, Drescher H, Bersinger NA. Semen quality of male smokers and nonsmokers in infertile couples. Fertil Steril. 2003; 79:287–291.


33. Pasqualotto FF, Umezu FM, Salvador M, Borges E Jr, Sobreiro BP, Pasqualotto EB. Effect of cigarette smoking on antioxidant levels and presence of leukocytospermia in infertile men: a prospective study. Fertil Steril. 2008; 90:278–283.


34. Said TM, Ranga G, Agarwal A. Relationship between semen quality and tobacco chewing in men undergoing infertility evaluation. Fertil Steril. 2005; 84:649–653.


35. Carrell DT. Contributions of spermatozoa to embryogenesis: assays to evaluate their genetic and epigenetic fitness. Reprod Biomed Online. 2008; 16:474–484.


36. Tesarik J. Paternal effects on cell division in the human preimplantation embryo. Reprod Biomed Online. 2005; 10:370–375.