Abstract
With growing accounts of inflammatory diseases such as sepsis, greater understanding the immune system and the mechanisms of cellular immunity have become primary objectives in immunology studies. High mobility group box 1 (HMGB1) is a ubiquitous nuclear protein that is implicated in various aspects of the innate immune system as a damage-associated molecular pattern molecule and a late mediator of inflammation, as well as in principal cellular processes, such as autophagy and apoptosis. HMGB1 functions in the nucleus as a DNA chaperone; however, it exhibits cytokine-like activity when secreted by injurious or infectious stimuli. Extracellular HMGB1 acts through specific receptors to promote activation of the NF-κB signaling pathway, leading to production of cytokines and chemokines. These findings further implicate HMGB1 in lethal inflammatory diseases as a crucial regulator of inflammatory, injurious, and infectious responses. In this paper, we summarize the role of HMGB1 in inflammatory and non-inflammatory states and assess potential therapeutic approaches targeting HMGB1 in inflammatory diseases.
High mobility group box 1 (HMGB1, previously HMG-1 or amphoterin) is a highly conserved, non-histone chromosomal protein that serves various roles in intracellular and extracellular microenvironments.1 HMGB1 was discovered 40 years ago as part of a group of chromatin proteins. This group of proteins was designated as the "high mobility group," due to their rapid electrophoretic mobility properties in polyacrylamide gels.2 Since its first discovery as a chromatin-associated protein, extensive studies of this group have led to the identification of several critical functions of HMGB1. In eukaryotic cells, this ubiquitous protein mainly remains in the nucleus, where it functions as a DNA chaperone facilitating DNA replication, V(D)J recombination, transcription, DNA repair, and the stabilization of nucleosome formation by bending the DNA helical structure and promoting the binding of regulatory complexes to DNA.3,4,5,6,7 Within the cytoplasm, HMGB1 takes part in regulating autophagy and maintaining balance between autophagy and apoptosis.8 Recently, cytoplasmic HMGB1 was also shown to act as a chaperon in the cytoplasm, using a model of Huntington's expanded polyglutamine traits, by reducing protein aggregation caused by heat or chemical stress.5 Apart from prevalent roles in the cell, extracellular HMGB1 also acts as a proinflammatory cytokine, secreted by activated macrophages, as a late mediator of inflammation.9
HMGB1 is crucial to embryogenesis and neonatal growth. In a previous study, Hmgb1 gene-knockout mice were born normal, but soon became less active and died of hypoglycemia within 24 h after birth. This was mainly due to impairment of glucocorticoid receptors that failed to activate gene expression and utilize glycogen stored in the liver.10 Hmgb1-/- mice also showed defects in endochondral ossification in skeletal development during embryogenesis, suggesting that HMGB1 may play a role in regulating endochondral ossification during osteogenesis.11 Furthermore, in an HMGB1 conditional knockout mouse study, conditional ablation of HMGB1 in mice showed higher vulnerability to lipopolysaccharide (LPS)-induced endotoxemia, along with crucial amount of macrophage cell death in tissues.12 This study further revealed the significance of HMGB1 in regulation of bacteria-induced autophagic processes and cell death in macrophages, suggesting a critical role of intracellular HMGB1 in protection against bacterial infection by modulating autophagy.12
Following its discovery, several subgroups of HMG proteins were identified in comprehensive studies. These additional members of the protein family were found to have different functions than those of nuclear HMG proteins. For clarification purposes, the nomenclature of HMG family proteins was revised, and HMG proteins were divided into three superfamilies based on their physicochemical properties.13 Each HMG superfamily is characterized by a distinguished functional sequence motif: HMGB superfamily with "HMG-box," HMGN with "nucleosomal binding domain," and HMGA with "AT-hook" (Table 1). HMGB1 is a member of the HMGB superfamily, which is characterized by the functional motif of a DNA-binding domain, or HMG box. Members of this superfamily, such as HMGB1 and HMGB2, nucleolar transcription factor upstream binding factor (UBF), lymphoid transcription factors T-cell factor (TCF)-1 and lymphoid enhancer factor (LEF)-1, fungal mating genes mat-Mc and MATa1, and mammalian sex-determining gene SRY, all comprise this DNA-binding domain in a single-sequence specific or non-sequence specific manner.14 This paper summarizes the role of HMGB1 as a damage-associated molecular pattern (DAMP) molecule in inflammatory and non-inflammatory conditions and discusses therapeutic potential of HMGB1 in various immune diseases.
HMGB1 is a 25-30 kDa protein that is structurally composed of two homologous DNA-binding domains, the A box and B box-each comprising approximately 80 amino acid residues-and a negatively charged acidic C-terminal tail that consists of approximately 30 consecutive aspartate and glutamate residues (Fig. 1A).15 The box A domain of HMGB1 is involved in the binding of HMGB1 to damaged DNA and acts as a specific antagonist to HMGB1, exhibiting an anti-inflammatory effect.16 It also consists of a heparin-binding domain (6-12) and a proteolytic cleavage site (10-11) mediated by thrombin. Reportedly, HMGB1 cleaved by thrombin-thrombomodulin complex leads to a reduction in proinflammatory activity and binding affinity with heparin.17 The box B domain, on the other hand, is related to the cytokine activity of full length HMGB1 by stimulating the release of tumor necrosis factor (TNF)-α and other proinflammatory cytokines in macrophages, in addition to its role in DNA binding.18 It contains two important binding sites for toll-like receptor (TLR) (89-108) and receptor for advanced glycation end products (RAGE) (105-183) that are crucial in the activation of macrophage cytokine release, where the first 20 residues represent the minimum amount of peptides required to induce proinflammatory response.18,19,20 Another peptide in the B box (130-139) is responsible for promoting erythroleukemia cell differentiation, where the presence of extracellular HMGB1 is essential in erythroid differentiation.21 The C-terminal tail is responsible for DNA damage repair and DNA bending, which then promotes other transcription factors to bind DNA.22,23 The significance of this C-terminal tail lies on its role in increasing the structural stability of HMGB1 and binding to A and B box alternatively.24,25 Additionally, HMGB1 consists of two LPS-binding peptide regions in the A box (3-15) and B box (80-96) that are important in HMGB1-LPS-mediated TLR4 signaling.26 The unique shape and structure of the protein defines the many roles HMGB1 plays in the cell. HMGB1 has three α-helices arranged in an L-shaped configuration that accounts for 75% of its total residues, and contains a significant amount of conserved basic and aromatic residues that specifically characterize the HMG-box.15 Within the 215 amino acid residues are target residues for post-translational modification sites, which are crucial in mediating the translocation of HMGB1 by acetylation, phosphorylation, methylation, and oxidation of HMGB1 (Fig. 1B).27,28,29,30
HMGB1 exhibits cytokine-like function as a proinflammatory mediator when released from the cell. This secretion of HMGB1 to the extracellular milieu occurs in two principal ways: actively by inflammatory cells and passively by necrotic and apoptotic cells (Fig. 2A). When the cytokine-like function of HMGB1 was first discovered, researchers reported that HMGB1 is secreted by macrophages and monocytes as a late response to activation by proinflammatory stimuli, such as LPS, interleukin (IL)-1, interferon (IFN)-γ, or TNF.9,31,32 These inflammatory cells modify HMGB1 by acetylation of lysine residues, which induces relocalization of HMGB1 from the nucleus to the cytosol.27 Hyperacetylated HMGB1 in the cytosol is then secreted via secretory vesicles or lysosomes when an appropriate secondary signal is received.27 HMGB1 is also released when TNF-α-induced phosphorylation occurs on the serine residues in the nuclear localization signals by classical protein kinase C (cPKC) in a calcium-dependent mechanism.29,33 Hyperphosphorylated HMGB1 translocates to the cytoplasm and shows decreased binding affinity with karyopherin-α1, a nuclear import protein, preventing itself from re-entering the nucleus.29 When this phosphorylation activity is inhibited, it restrains the secretion of HMGB1 by blocking the LPS-stimulated phosphoinositide 3-kinase (PI3K) and cPKC signaling pathways.34 Another posttranslational modification that induces active secretion of HMGB1 in neutrophils is through methylation at Lys42, which reduces DNA binding affinity by a conformational change in the box A domain of HMGB1, thereby causing diffusion out from the nucleus to the cytoplasm.30 HMGB1 also consists of three cysteine residues: Cys23, Cys45, and Cys106. Cys23 and Cys45 in the A box undergo a conformational change in response to oxidative stress, whereas Cys106 was recently identified to be involved in nucleocytoplasmic translocation of HMGB1.28 The proinflammatory cytokine activity of HMGB1 is dependent upon the redox modification state of these three cysteine residues (Fig. 2B). Recombinant HMGB1 is freely reduced and oxidized in the presence of electron donors or acceptors, such as oxygen.35 The reduced form of HMGB1 exhibits all-thiol groups that induce chemokine activity and increase autophagy level, specifically in cancer cells.36,37 The disulfide form of HMGB1, with Cys23 and Cys45 forming a disulfide bridge, is responsible for inducing proinflammatory cytokine activity, as well as activation of NF-κB.35 HMGB1 in its fully oxidized form loses its functions of inducing proinflammatory effect and activating caspase-dependent apoptotic cell death.36,37 Aside from the secretion of HMGB1, Cys106 also plays a role in binding TLR4, which will be further discussed later.
The cytokine activity of extracellular HMGB1 is not solely dependent on active secretion by inflammatory cells, as it is also passively released by necrotic and apoptotic cells. In a normal state HMGB1 is loosely associated with nucleosomes in the chromosomes. HMGB1 is diffused out of the nuclei when membrane permeability is lost or when cells go through necrosis.38,39,40 HMGB1 secreted by necrotic cells transmits demise signals to neighboring cells and triggers inflammatory response.39,40 Another passive secretion mechanism of HMGB1 involves cells dying via apoptosis. Initially, early investigators concluded that apoptotic cells do not significantly secrete HMGB1.39 Further studies have showed that cells that undergo apoptosis do release considerable amounts of HMGB1, except those that are immunologically inactive because they fail to stimulate TNF production from responding macrophages.41 When cells undergo apoptosis, a programmed cell death process, they activate macrophages that engulf apoptotic cells which secrete HMGB1 and release nuclear molecules including DNA that binds HMGB1.41,42
Once secreted from cells through active and passive mechanisms, HMGB1 induces inflammatory responses by signal transduction pathways through many binding receptors (Fig. 3). When binding to its major transmembrane receptors, HMGB1 associates and shows interactions with several molecules, such as IL-1β, RNA and DNA, LPS, lipoteichoic acid (LTA), CXCL12 (stromal cell-derived factor-1), and nucleosomes.43,44,45,46,47 HMGB1 physically interacts with a polygamous molecule of RAGE, which is a cell surface, multi-ligand member of the immunoglobulin superfamily.20 HMGB1 bound to RAGE mediates signals for neuronal outgrowth and promotes activation of TLR9-mediated cytokine production.20,48 HMGB1 actively secreted by maturing dendritic cells also signals through RAGE; this signal is responsible for proliferation and polarization of T cells, as well as up-regulation of other cell-surface receptors, such as TLR4 and RAGE.49 As HMGB1 binds to RAGE, it triggers activation of Ras, PI3K, and Rho, which are major downstream signaling molecules towards NF-κB activation.50 The proinflammatory effect of HMGB1 is significant in the signal transduction pathway of NF-κB, which involves extracellular signal-regulated kinase 1 and 2, and p38 mitogen-activated protein kinase, by increasing the nuclear translocation of NF-κB and thus enhancing proinflammatory cytokine expressions.45,51,52
Subsequently, HMGB1 signals to other multi-ligand receptors of TLR4 and TLR2 by direct binding of TLR4 or by binding to LPS.46,53,54 It is well known that LPS is recognized by LPS-binding protein (LBP) and transferred to CD14 for signaling to TLR4/MD2 complex.53,54 Here, HMGB1 acts as a sensor molecule by binding to LPS, as well as a transferring molecule that brings the complex to CD14 and, consequently, to TLR4 for downstream signaling that leads to NF-κB activation, providing a synergistic effect on LPS-TLR4 signaling.26,46 Similarly, LTA, a Gram (+) bacteria cell wall component, is transferred to CD14 by LBP for signaling through TLR2-mediated NF-κB activation.55 The function of HMGB1 in the transfer of LTA to CD14 needs further investigation. HMGB1 also interacts with TLR9, a member of the TLR family located in the endoplasmic reticulum-Golgi intermediate compartment.56 HMGB1 is an essential molecule of the DNA complex that promotes cytokine release through binding to TLR9 and signaling through downstream pathways involving MyD88 and NF-κB.48 Moreover, HMGB1 interaction with CpG-DNA enhances redistribution of TLR9 to early endosomes and augmented cytokine production.48,57 The MyD88-dependent mechanism of NF-κB activation by HMGB1, therefore, allows the transcription of proinflammatory genes, such as IL-1, IL-6, and TNF.45 Extracellular HMGB1 also functions in chemotaxis and cell migration through its interaction with CXCL12 and its binding receptor, CXCR4. CXCL12 is a chemokine protein that binds to CXCR4 and mediates signaling pathways that induce chemotaxis, cell migration, and cell proliferation, as well as the recruitment of inflammatory cells.58 HMGB1 secreted from migrating dendritic cells (DCs) and macrophages enhances these CXCL12-induced activities by physically associating with CXCL12 to form a heterocomplex that helps maintain its conformational structure under reduced conditions.47,59 According to a previous report, this CXCL12-induced cell migration was exclusive to CXCR4 alone, and neither RAGE nor TLR showed synergistic effects on HMGB1 binding to CXCL12.59
The activity of HMGB1-triggered cytokine production via TLR4 is sensitive to the redox state of HMGB1 as it binds to TLR4 in a structure-specific manner. The recombinant B box domain of HMGB1 interacts specifically with the TLR4/MD2 complex, where a reduced form of HMGB1 cysteine 106 is required.19 This hints that the pivotal difference in immune activity between necrotic cells and apoptotic cells might lie on the redox state of HMGB1. During necrosis, HMGB1 is released with a reduced form of Cys106, which can initiate inflammation by binding to TLR4. HMGB1 released from apoptotic cells, however, comprises an oxidized form of Cys106 that prevents its interaction with TLR4.60,61 Along with the reduced form of Cys106, disulfide linkages between Cys23 and Cys45 are also required for HMGB1 binding to TLR.62 However, the direct interaction between Cys106 and TLR4 has not been confirmed, and the exact mechanism of the role in TLR4 binding still remains unclear. The TLR family, including TLR4, is known to transmit proinflammatory signals through a ligand binding-induced oligomerization, as well as the oligomerization of the partner ligands.63 We have identified that HMGB1 oligomerization occurs through Cys106-mediated disulfide bond in oxidative conditions and that oligomerized-HMGB1 increasingly binds with TLR4 and enhances inflammatory responses (unpublished data). Thus, Cys106 mediates oligomerization of HMGB1 in oxidative conditions induced by bacterial or viral infections in the cell, and may play a critical role in inducing proinflammatory signals through the solid binding of oligomerized-HMGB1 and TLR4.
Recently, over the past few years, there has been rising interest on a group of endogenous danger signals that play a critical proinflammatory role in innate immunity, called DAMP molecules.64 These molecules, also known as "alarmins," are released by stressed or damaged cells and contribute to induction of inflammatory responses by binding to pattern recognition receptors (PRRs).64 Immune alarmins share a few characteristic in the immune system: they enhance adaptive immune responses, activate antigen-presenting cells, and are rapidly released upon tissue injury.64,65 DAMP molecules such as defensins and cathelicidin are rapidly released by epithelial cells or leukocytes when they encounter with pathogen-associated molecular patterns (PAMP) molecules or proinflammatory cytokines.64,65,66 HMGB1 is one of the best known DAMP molecules. HMGB1 is secreted by injured cells, specifically upon necrotic cell death.39,40 Aside from its capability in enhancing antigen-specific immune response, HMGB1 also acts as a chemoattractant and activator of dendritic cells that recruits DCs to their site of release.67,68,69 In a previous study, inflammatory responses induced by DAMPs showed high similarity with responses induced by PAMPs, suggesting that they may use common receptors and pathways to initiate host defense mechanism in cells of innate immune system.64 Major receptors that detect endogenous danger signals for inducing cytokine production include RAGE, TLR2, TLR4, and other cell surface binding sites, such as carboxylated glycans and heparin sulfate proteoglycans.70
Through binding to specific receptors, DAMP molecules induce early innate and adaptive immune inflammation without any infection. This early response, termed sterile inflammation, is triggered by cellular stress as a result of trauma, ischemia-reperfusion injury, or other chemically-induced tissue injury. Immune responses in sterile inflammatory conditions are characterized by recruitment of inflammatory cells, generation of memory T cell subsets, and production of proinflammatory cytokines and chemokines.71,72 Mechanisms by which DAMPs trigger sterile inflammation are through activation of PRRs and common downstream signaling, release of proinflammatory cytokines and chemokines, and binding to specific DAMP receptors that are not PRRs.
HMGB1 acts as a DAMP molecule when released by necrotic cells or secreted by activated macrophages. It controls maturation and migration of dendritic cells and activates T-cells through binding to RAGE, thereby functioning as a significant mediator of sterile inflammation in innate immune system.49,67,69 These monocytes and T cells produce proinflammatory signals and cytokines that augment inflammatory conditions.31 HMGB1 is also involved in the production of IL-1β, TNF-α, and IL-6 in murine macrophages by binding to RAGE.73 HMGB1 bound to DNA promoted maturation of immune cells and cytokine production through RAGE and TLR9-MyD88-dependent pathway.48,57 HMGB1 that remained bound to nucleosomes in secretion from apoptotic cells induced activation of antigen-presenting cells and secretion of cytokines by macrophages.45 Recently, emerging findings on the proinflammatory cytokine activity of HMGB1 show that it acts as a danger signal, especially in the pathogenesis of autoimmune diseases, including ischemia-reperfusion injury, trauma, rheumatoid arthritis, and lupus erythematosus.74,75,76,77 Studies have also suggested that HMGB1 is involved in tissue repair and remodeling activity, such as angiogenesis, a novel role of DAMP molecules.78,79
HMGB1 is also implicated in non-inflammatory conditions, such as cell metabolism through autophagy and mitophagy, cancer, angiogenesis, tolerance induction, complement binding, and other non-inflammatory states. HMGB1 is a critical regulator of autophagy, an important cellular mechanism for maintaining homeostasis and protecting organisms from pathologies of multiple diseases by degrading dysfunctional cellular components, thus enhancing survival of cells. HMGB1 translocated to the cytoplasm due to oxidative stress forms an intramolecular disulfide bridge between Cys23 and Cys45 that is essential in binding the autophagy protein Beclin1 and further enhancing the process of autophagy.8,80 Extracellular reduced HMGB1 signals through RAGE inhibits mammalian target of rapamycin (mTOR), which then increases Beclin1-PtdIns3KC3 interaction that further promotes autophagic processes.81 Recently, a discrepancy in this finding was reported by a group that have investigated the dispensability of HMGB1 in autophagy through a HMGB1 conditional knockout mouse study.82 HMGB1 is also important in regulation of mitochondrial activity. Nuclear HMGB1 enhances gene expression of heat shock protein beta 1 (HSPB1), and phosphorylation of HSPB1 induces formation of actin cytoskeleton that regulates cellular transport and mitochondria degradation in mitophagy through the Pink1-Parkin pathway.81
In cancer stem cells, HMGB1 was identified as binding to TLR2 and inducing NF-κB activation by phosphorylation of IκBα, as well as secretion of IL-6 and TGF-β and activation of STAT3 and Smad3.83 These cytokines regulate tumorigenesis through proliferation and self-renewal of cancer cells independent of tumor inflammation.84 Secreted IL-6 enhances malignant properties of cancer cells and TGF-β signaling contributes to metastasis and survival of cancer stem cells.85,86 HMGB1 also plays a pivotal proangiogenetic role in angiogenesis by stimulating cell proliferation and chemotaxis in endothelial cells during tissue damage.87 The production of these proangiogenic factors by HMGB1 are induced through the TLR4 signaling pathway and contribute to neovascularization and vascular growth.88 Additionally, HMGB1 regulates autocrine and paracrine signaling in angiogenic gene expression of endothelial cells, which suggests its proangiogenetic role in tumor angiogenesis.89
HMGB1 also functions as an immune tolerance-inducing molecule. HMGB1 induces the proinflammatory effect of LPS and endotoxin tolerance through a RAGE-dependent signaling pathway. These HMGB1-tolerant macrophages exhibit down regulation of intracellular signaling pathways, which subsequently decrease the activity of proinflammatory gene transcription and production of cytokines, such as TNF-α, IL-6, and IL-1β.90 Similarly, HMGB1 also induces LTA tolerance, but does not require RAGE for its induction.91 Further investigation is necessary to identify whether TLR4 or other receptors are involved in the induction of tolerance by preconditioning with HMGB1. HMGB1 is also implicated in epileptic seizures, as patients with epilepsy exhibit significantly higher serum levels of HMGB1, suggesting a potential pathological contribution of HMGB1 in febrile seizures and epilepsy.92 Besides the prevalent findings of the role of HMGB1 in various areas, the role of HMGB1 in activation of complement pathways and other binding receptors is currently being studied.
Since its discovery, HMGB1 has been a growing interest after its implication in the pathogenesis of sepsis. The proinflammatory cytokine activity of HMGB1 was first discovered when HMGB1 was secreted by activated macrophages as a late mediator of lethal sepsis in murine model.9 Sepsis is a lethal inflammatory disease that accounts for 20 to 30 million cases every year, with a higher mortality rate than prostate cancer, breast cancer, and HIV/AIDS combined.93 In a murine model of sepsis induced by endotoxin and surgical cecal ligation and puncture (CLP), HMGB1 was first detected after 8 h, followed by a continuous increase during the 16 h to 32 h period.9,16 The pathogenic role of HMGB1 in sepsis was further studied and confirmed when injection of neutralizing anti-HMGB1 antibodies significantly increased the survival rate of sepsis in a dose-dependent manner, suggesting that it has a protective role against endotoxin-induced tissue injury.16,42 The cytokine activity of HMGB1 was also inhibited in a CLP sepsis model by administering recombinant HMGB1 A box, which plays a role in binding HMGB1 to damaged DNA in a time- and dose-dependent manner.16 These findings gave insights to HMGB1 as a therapeutic target in sepsis, and suggested potential therapeutic roles for agents, such as Epigallocatechin gallate from green tea, that inhibit the release of HMGB1 (Fig. 4).94
HMGB1 is also implicated as an important mediator in cancer. HMGB1 expression is high in many tumor cells, especially in colon cancer, breast cancer, prostate cancer, and pancreatic cancer.95,96 Studies have shown that there is a correlation between HMGB1 and the malignant phenotypes of various cancers.97 The principal behind this correlation may be due to the role of extracellular HMGB1 in angiogenesis, cell migration, and recruitment of macrophages, which can all contribute to tumor growth and metastasis.79,87,98 As part of its proinflammatory cytokine activity, extracellular HMGB1 also activates vascular endothelial cells, stimulating proliferation and sprouting in tumor cells.87 In a precious study, inactivating this pathway by blocking the interaction between the binding receptor RAGE and HMGB1 lead to defects in tumor growth and metastasis in murine model, indicating that HMGB1 does contribute to the proliferation of tumor cells.52 This suggests that HMGB1 can be a potential target for cancer therapy in the future. One conflict about targeting HMGB1 inhibitor as a cancer therapy is that there may be cross-presentation of tumor antigens through TLR4, as HMGB1 is also rapidly released after chemotherapy and radiotherapy, playing a crucial role in DC recruitment and maturation, tumor-specific T cell activation, and antitumor immune response.99
HMGB1 is a ubiquitous protein that has diverse roles in mediating significant pathways of inflammation in the intra- and extra-cellular microenvironment. With the growing studies on HMGB1 and its cytokine-like function in innate immunity, HMGB1 is implicated as a key mediator in inflammatory mechanisms in the pathogenesis of many autoimmune and inflammatory diseases. It is also known that modification of HMGB1 and its interaction with various molecules are significant mediators of signaling pathways, suggesting that HMGB1 can serve as a new target for therapeutic purposes. However, the exact pathological mechanisms and direct involvement of HMGB1 in these diseases are still unknown. In order to further define the immunological activities of HMGB1 in inflammation, identification of exact mechanisms is necessary.
Figures and Tables
Fig. 1
Structure of HMGB1. (A) HMGB1 protein is composed of 215 amino acids in three structural domains: the A box (1-79), B box (89-162), and the acidic C tail (186-215). The A box functions in DNA binding and inducing anti-inflammatory effects, whereas the B box domain plays an important role in DNA binding and stimulating proinflammatory responses. The B box domain consists of two crucial binding sites for TLR4 and RAGE that mediate the release of proinflammatory cytokines. In particular, the 20 amino acids of the TLR4 binding site (89-108) are the minimal sequence needed to induce cytokine activity. Two peptide regions (3-15, 80-96) of HMGB1 bind to delipidated LPS and lipid A regions, respectively. (B) The functional component of HMGB1 is represented in a linear diagram underlining the amino acid residues that constitute the A box domain (pink), B box domain (purple), and C tail (blue). Symbols are used to denote specific sites for the four post-translational modifications that HMGB1 goes through during active secretion: methylation, oxidation, phosphorylation, and acetylation.
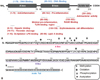
Fig. 2
Secretion mechanism and inflammatory role of HMGB1 in redox states. (A) HMGB1 is translocated to the extracellular environment via two secretion mechanisms: active secretion by inflammatory cells or passive release by necrotic or apoptotic cells. HMGB1 in the nucleus is actively secreted when immunologically competent cells are activated by inflammatory stimulus and undergo post-translation modifications such as acetylation, phosphorylation, methylation, and redox change. Passive release of HMGB1 is mediated by necrotic or apoptotic cell death caused by injury. Released HMGB1 triggers inflammatory responses in the body. (B) The inflammatory activity of released extracellular HMGB1 is dependent upon its redox state. HMGB1 consists of three cysteine residues (C23, C45, C106) that are modified during redox change. The reduced form of HMGB1 with all thiol groups defines the chemokine activity of HMGB1, whereas the disulfide-HMGB1 with C23 and C45, forming an intermolecular disulfide bond, induces cytokine activity. The fully oxidized form of HMGB1 has no known immune function in the cells.
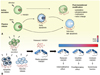
Fig. 3
HMGB1 binding to receptors and activating signal transduction pathway. Interaction of HMGB1 with RAGE, TLR2, TLR4, and TLR9 transduce cellular signals through a common pathway that leads to activation of NF-κB. HMGB1 interaction with LPS, LTA, and CpG enhances TLR4-, TLR2-, and TLR9-mediated signaling, respectively, and further leads to downstream signaling of NF-κB activation and proinflammatory cytokine production. Activated NF-κB gets released from IκB translocates to the nucleus and binds to the DNA in the most abundant form of NF-κB, the p65/p50 heterodimer form. HMGB1 also interacts with CXCL12, which binds to CXCR4 and induces chemotaxis and recruitment of inflammatory cells.
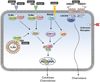
Fig. 4
Targeting HMGB1 as a potential therapeutic approach for inflammatory diseases. HMGB1 can be targeted in various areas to potentially block the proinflammatory cytokine effect of HMGB1 in inflammatory disease models. HMGB1 is actively released upon infection by inflammatory cells or passively released by necrotic cells. Inhibiting the release of HMGB1 and blocking the activity of extracellular HMGB1 can reduce the inflammatory responses induced by HMGB1. Blockade of HMGB1 binding receptors can inhibit the production of proinflammatory cytokines by HMGB1 in immune cells.
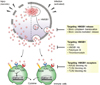
Table 1
HMG Chromosomal Protein Family and Its Nomenclature*
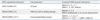
*Http://www.informatics.jax.org/mgihome/nomen/hmg_family.shtml.
†Names of canonical HMG proteins are matched old names in serial respectively.
ACKNOWLEDGEMENTS
This work was supported by grants from the National Research Foundation of Korea (NRF) grant funded by the Korea government (MEST) (2011-0017611 and 2014R1A4A 1008625).
References
1. Goodwin GH, Rabbani A, Nicolas PH, Johns EW. The isolation of the high mobility group non-histone chromosomal protein HMG 14. FEBS Lett. 1977; 80:413–416.


2. Goodwin GH, Sanders C, Johns EW. A new group of chromatin-associated proteins with a high content of acidic and basic amino acids. Eur J Biochem. 1973; 38:14–19.


3. Javaherian K, Liu JF, Wang JC. Nonhistone proteins HMG1 and HMG2 change the DNA helical structure. Science. 1978; 199:1345–1346.


4. Stros M. HMGB proteins: interactions with DNA and chromatin. Biochim Biophys Acta. 2010; 1799:101–113.


5. Min HJ, Ko EA, Wu J, Kim ES, Kwon MK, Kwak MS, et al. Chaperone-like activity of high-mobility group box 1 protein and its role in reducing the formation of polyglutamine aggregates. J Immunol. 2013; 190:1797–1806.


6. Bianchi ME, Beltrame M, Paonessa G. Specific recognition of cruciform DNA by nuclear protein HMG1. Science. 1989; 243(4894 Pt 1):1056–1059.


7. Giese K, Cox J, Grosschedl R. The HMG domain of lymphoid enhancer factor 1 bends DNA and facilitates assembly of functional nucleoprotein structures. Cell. 1992; 69:185–195.


8. Tang D, Kang R, Livesey KM, Cheh CW, Farkas A, Loughran P, et al. Endogenous HMGB1 regulates autophagy. J Cell Biol. 2010; 190:881–892.


9. Wang H, Bloom O, Zhang M, Vishnubhakat JM, Ombrellino M, Che J, et al. HMG-1 as a late mediator of endotoxin lethality in mice. Science. 1999; 285:248–251.


10. Calogero S, Grassi F, Aguzzi A, Voigtländer T, Ferrier P, Ferrari S, et al. The lack of chromosomal protein Hmg1 does not disrupt cell growth but causes lethal hypoglycaemia in newborn mice. Nat Genet. 1999; 22:276–280.


11. Taniguchi N, Yoshida K, Ito T, Tsuda M, Mishima Y, Furumatsu T, et al. Stage-specific secretion of HMGB1 in cartilage regulates endochondral ossification. Mol Cell Biol. 2007; 27:5650–5663.


12. Yanai H, Matsuda A, An J, Koshiba R, Nishio J, Negishi H, et al. Conditional ablation of HMGB1 in mice reveals its protective function against endotoxemia and bacterial infection. Proc Natl Acad Sci U S A. 2013; 110:20699–20704.


13. Bustin M. Revised nomenclature for high mobility group (HMG) chromosomal proteins. Trends Biochem Sci. 2001; 26:152–153.


14. Laudet V, Stehelin D, Clevers H. Ancestry and diversity of the HMG box superfamily. Nucleic Acids Res. 1993; 21:2493–2501.


15. Weir HM, Kraulis PJ, Hill CS, Raine AR, Laue ED, Thomas JO. Structure of the HMG box motif in the B-domain of HMG1. EMBO J. 1993; 12:1311–1319.


16. Yang H, Ochani M, Li J, Qiang X, Tanovic M, Harris HE, et al. Reversing established sepsis with antagonists of endogenous high-mobility group box 1. Proc Natl Acad Sci U S A. 2004; 101:296–301.


17. Ito T, Kawahara K, Okamoto K, Yamada S, Yasuda M, Imaizumi H, et al. Proteolytic cleavage of high mobility group box 1 protein by thrombin-thrombomodulin complexes. Arterioscler Thromb Vasc Biol. 2008; 28:1825–1830.


18. Li J, Kokkola R, Tabibzadeh S, Yang R, Ochani M, Qiang X, et al. Structural basis for the proinflammatory cytokine activity of high mobility group box 1. Mol Med. 2003; 9:37–45.


19. Yang H, Hreggvidsdottir HS, Palmblad K, Wang H, Ochani M, Li J, et al. A critical cysteine is required for HMGB1 binding to Toll-like receptor 4 and activation of macrophage cytokine release. Proc Natl Acad Sci U S A. 2010; 107:11942–11947.


20. Hori O, Brett J, Slattery T, Cao R, Zhang J, Chen JX, et al. The receptor for advanced glycation end products (RAGE) is a cellular binding site for amphoterin. Mediation of neurite outgrowth and co-expression of rage and amphoterin in the developing nervous system. J Biol Chem. 1995; 270:25752–25761.


21. Sparatore B, Passalacqua M, Patrone M, Melloni E, Pontremoli S. Extracellular high-mobility group 1 protein is essential for murine erythroleukaemia cell differentiation. Biochem J. 1996; 320(Pt 1):253–256.


22. Mitkova E, Ugrinova I, Pashev IG, Pasheva EA. The inhibitory effect of HMGB-1 protein on the repair of cisplatin-damaged DNA is accomplished through the acidic domain. Biochemistry. 2005; 44:5893–5898.


23. Belgrano FS, de Abreu da Silva IC, Bastos de Oliveira FM, Fantappié MR, Mohana-Borges R. Role of the acidic tail of high mobility group protein B1 (HMGB1) in protein stability and DNA bending. PLoS One. 2013; 8:e79572.


24. Knapp S, Müller S, Digilio G, Bonaldi T, Bianchi ME, Musco G. The long acidic tail of high mobility group box 1 (HMGB1) protein forms an extended and flexible structure that interacts with specific residues within and between the HMG boxes. Biochemistry. 2004; 43:11992–11997.


25. Watson M, Stott K, Thomas JO. Mapping intramolecular interactions between domains in HMGB1 using a tail-truncation approach. J Mol Biol. 2007; 374:1286–1297.


26. Youn JH, Kwak MS, Wu J, Kim ES, Ji Y, Min HJ, et al. Identification of lipopolysaccharide-binding peptide regions within HMGB1 and their effects on subclinical endotoxemia in a mouse model. Eur J Immunol. 2011; 41:2753–2762.


27. Bonaldi T, Talamo F, Scaffidi P, Ferrera D, Porto A, Bachi A, et al. Monocytic cells hyperacetylate chromatin protein HMGB1 to redirect it towards secretion. EMBO J. 2003; 22:5551–5560.


28. Hoppe G, Talcott KE, Bhattacharya SK, Crabb JW, Sears JE. Molecular basis for the redox control of nuclear transport of the structural chromatin protein Hmgb1. Exp Cell Res. 2006; 312:3526–3538.


29. Youn JH, Shin JS. Nucleocytoplasmic shuttling of HMGB1 is regulated by phosphorylation that redirects it toward secretion. J Immunol. 2006; 177:7889–7897.


30. Ito I, Fukazawa J, Yoshida M. Post-translational methylation of high mobility group box 1 (HMGB1) causes its cytoplasmic localization in neutrophils. J Biol Chem. 2007; 282:16336–16344.


31. Andersson U, Wang H, Palmblad K, Aveberger AC, Bloom O, Erlandsson-Harris H, et al. High mobility group 1 protein (HMG-1) stimulates proinflammatory cytokine synthesis in human monocytes. J Exp Med. 2000; 192:565–570.


32. Gardella S, Andrei C, Ferrera D, Lotti LV, Torrisi MR, Bianchi ME, et al. The nuclear protein HMGB1 is secreted by monocytes via a non-classical, vesicle-mediated secretory pathway. EMBO Rep. 2002; 3:995–1001.


33. Oh YJ, Youn JH, Ji Y, Lee SE, Lim KJ, Choi JE, et al. HMGB1 is phosphorylated by classical protein kinase C and is secreted by a calcium-dependent mechanism. J Immunol. 2009; 182:5800–5809.


34. Oh YJ, Youn JH, Min HJ, Kim DH, Lee SS, Choi IH, et al. CKD712, (S)-1-(α-naphthylmethyl)-6,7-dihydroxy-1,2,3,4-tetrahydroisoquinoline, inhibits the lipopolysaccharide-stimulated secretion of HMGB1 by inhibiting PI3K and classical protein kinase C. Int Immunopharmacol. 2011; 11:1160–1165.


35. Venereau E, Casalgrandi M, Schiraldi M, Antoine DJ, Cattaneo A, De Marchis F, et al. Mutually exclusive redox forms of HMGB1 promote cell recruitment or proinflammatory cytokine release. J Exp Med. 2012; 209:1519–1528.


36. Tang D, Billiar TR, Lotze MT. A Janus tale of two active high mobility group box 1 (HMGB1) redox states. Mol Med. 2012; 18:1360–1362.


37. Tang D, Kang R, Cheh CW, Livesey KM, Liang X, Schapiro NE, et al. HMGB1 release and redox regulates autophagy and apoptosis in cancer cells. Oncogene. 2010; 29:5299–5310.


38. Falciola L, Spada F, Calogero S, Langst G, Voit R, Grummt I, et al. High mobility group 1 protein is not stably associated with the chromosomes of somatic cells. J Cell Biol. 1997; 137:19–26.


39. Scaffidi P, Misteli T, Bianchi ME. Release of chromatin protein HMGB1 by necrotic cells triggers inflammation. Nature. 2002; 418:191–195.


40. Rovere-Querini P, Capobianco A, Scaffidi P, Valentinis B, Catalanotti F, Giazzon M, et al. HMGB1 is an endogenous immune adjuvant released by necrotic cells. EMBO Rep. 2004; 5:825–830.


41. Bell CW, Jiang W, Reich CF 3rd, Pisetsky DS. The extracellular release of HMGB1 during apoptotic cell death. Am J Physiol Cell Physiol. 2006; 291:C1318–C1325.


42. Qin S, Wang H, Yuan R, Li H, Ochani M, Ochani K, et al. Role of HMGB1 in apoptosis-mediated sepsis lethality. J Exp Med. 2006; 203:1637–1642.


43. Paull TT, Haykinson MJ, Johnson RC. The nonspecific DNA-binding and -bending proteins HMG1 and HMG2 promote the assembly of complex nucleoprotein structures. Genes Dev. 1993; 7:1521–1534.


44. Sha Y, Zmijewski J, Xu Z, Abraham E. HMGB1 develops enhanced proinflammatory activity by binding to cytokines. J Immunol. 2008; 180:2531–2537.


45. Urbonaviciute V, Fürnrohr BG, Meister S, Munoz L, Heyder P, De Marchis F, et al. Induction of inflammatory and immune responses by HMGB1-nucleosome complexes: implications for the pathogenesis of SLE. J Exp Med. 2008; 205:3007–3018.


46. Youn JH, Oh YJ, Kim ES, Choi JE, Shin JS. High mobility group box 1 protein binding to lipopolysaccharide facilitates transfer of lipopolysaccharide to CD14 and enhances lipopolysaccharide-mediated TNF-alpha production in human monocytes. J Immunol. 2008; 180:5067–5074.


47. Campana L, Bosurgi L, Bianchi ME, Manfredi AA, Rovere-Querini P. Requirement of HMGB1 for stromal cell-derived factor-1/CXCL12-dependent migration of macrophages and dendritic cells. J Leukoc Biol. 2009; 86:609–615.


48. Tian J, Avalos AM, Mao SY, Chen B, Senthil K, Wu H, et al. Toll-like receptor 9-dependent activation by DNA-containing immune complexes is mediated by HMGB1 and RAGE. Nat Immunol. 2007; 8:487–496.


49. Dumitriu IE, Baruah P, Valentinis B, Voll RE, Herrmann M, Nawroth PP, et al. Release of high mobility group box 1 by dendritic cells controls T cell activation via the receptor for advanced glycation end products. J Immunol. 2005; 174:7506–7515.


50. Huttunen HJ, Fages C, Rauvala H. Receptor for advanced glycation end products (RAGE)-mediated neurite outgrowth and activation of NF-kappaB require the cytoplasmic domain of the receptor but different downstream signaling pathways. J Biol Chem. 1999; 274:19919–19924.


51. Park JS, Arcaroli J, Yum HK, Yang H, Wang H, Yang KY, et al. Activation of gene expression in human neutrophils by high mobility group box 1 protein. Am J Physiol Cell Physiol. 2003; 284:C870–C879.


52. Taguchi A, Blood DC, del Toro G, Canet A, Lee DC, Qu W, et al. Blockade of RAGE-amphoterin signalling suppresses tumour growth and metastases. Nature. 2000; 405:354–360.


53. Park BS, Song DH, Kim HM, Choi BS, Lee H, Lee JO. The structural basis of lipopolysaccharide recognition by the TLR4-MD-2 complex. Nature. 2009; 458:1191–1195.


54. Kim S, Kim SY, Pribis JP, Lotze M, Mollen KP, Shapiro R, et al. Signaling of high mobility group box 1 (HMGB1) through toll-like receptor 4 in macrophages requires CD14. Mol Med. 2013; 19:88–98.


55. Schröder NW, Morath S, Alexander C, Hamann L, Hartung T, Zähringer U, et al. Lipoteichoic acid (LTA) of Streptococcus pneumoniae and Staphylococcus aureus activates immune cells via Toll-like receptor (TLR)-2, lipopolysaccharide-binding protein (LBP), and CD14, whereas TLR-4 and MD-2 are not involved. J Biol Chem. 2003; 278:15587–15594.


56. Latz E, Schoenemeyer A, Visintin A, Fitzgerald KA, Monks BG, Knetter CF, et al. TLR9 signals after translocating from the ER to CpG DNA in the lysosome. Nat Immunol. 2004; 5:190–198.


57. Ivanov S, Dragoi AM, Wang X, Dallacosta C, Louten J, Musco G, et al. A novel role for HMGB1 in TLR9-mediated inflammatory responses to CpG-DNA. Blood. 2007; 110:1970–1981.


58. Teicher BA, Fricker SP. CXCL12 (SDF-1)/CXCR4 pathway in cancer. Clin Cancer Res. 2010; 16:2927–2931.
59. Schiraldi M, Raucci A, Muñoz LM, Livoti E, Celona B, Venereau E, et al. HMGB1 promotes recruitment of inflammatory cells to damaged tissues by forming a complex with CXCL12 and signaling via CXCR4. J Exp Med. 2012; 209:551–563.


60. Kazama H, Ricci JE, Herndon JM, Hoppe G, Green DR, Ferguson TA. Induction of immunological tolerance by apoptotic cells requires caspase-dependent oxidation of high-mobility group box-1 protein. Immunity. 2008; 29:21–32.


61. Antoine DJ, Williams DP, Kipar A, Laverty H, Park BK. Diet restriction inhibits apoptosis and HMGB1 oxidation and promotes inflammatory cell recruitment during acetaminophen hepatotoxicity. Mol Med. 2010; 16:479–490.


62. Yang H, Lundbäck P, Ottosson L, Erlandsson-Harris H, Venereau E, Bianchi ME, et al. Redox modification of cysteine residues regulates the cytokine activity of high mobility group box-1 (HMGB1). Mol Med. 2012; 18:250–259.


63. Saitoh S, Akashi S, Yamada T, Tanimura N, Matsumoto F, Fukase K, et al. Ligand-dependent Toll-like receptor 4 (TLR4)-oligomerization is directly linked with TLR4-signaling. J Endotoxin Res. 2004; 10:257–260.


64. Oppenheim JJ, Yang D. Alarmins: chemotactic activators of immune responses. Curr Opin Immunol. 2005; 17:359–365.


65. Yang D, Oppenheim JJ. Antimicrobial proteins act as "alarmins" in joint immune defense. Arthritis Rheum. 2004; 50:3401–3403.


67. Yang D, Chen Q, Yang H, Tracey KJ, Bustin M, Oppenheim JJ. High mobility group box-1 protein induces the migration and activation of human dendritic cells and acts as an alarmin. J Leukoc Biol. 2007; 81:59–66.


68. Semino C, Angelini G, Poggi A, Rubartelli A. NK/iDC interaction results in IL-18 secretion by DCs at the synaptic cleft followed by NK cell activation and release of the DC maturation factor HMGB1. Blood. 2005; 106:609–616.


69. Messmer D, Yang H, Telusma G, Knoll F, Li J, Messmer B, et al. High mobility group box protein 1: an endogenous signal for dendritic cell maturation and Th1 polarization. J Immunol. 2004; 173:307–313.


70. Srikrishna G, Huttunen HJ, Johansson L, Weigle B, Yamaguchi Y, Rauvala H, et al. N -Glycans on the receptor for advanced glycation end products influence amphoterin binding and neurite outgrowth. J Neurochem. 2002; 80:998–1008.


71. Chen GY, Nuñez G. Sterile inflammation: sensing and reacting to damage. Nat Rev Immunol. 2010; 10:826–837.


72. Mbitikon-Kobo FM, Vocanson M, Michallet MC, Tomkowiak M, Cottalorda A, Angelov GS, et al. Characterization of a CD44/CD122int memory CD8 T cell subset generated under sterile inflammatory conditions. J Immunol. 2009; 182:3846–3854.


73. Kokkola R, Andersson A, Mullins G, Ostberg T, Treutiger CJ, Arnold B, et al. RAGE is the major receptor for the proinflammatory activity of HMGB1 in rodent macrophages. Scand J Immunol. 2005; 61:1–9.


74. Goldstein RS, Gallowitsch-Puerta M, Yang L, Rosas-Ballina M, Huston JM, Czura CJ, et al. Elevated high-mobility group box 1 levels in patients with cerebral and myocardial ischemia. Shock. 2006; 25:571–574.


75. Levy RM, Mollen KP, Prince JM, Kaczorowski DJ, Vallabhaneni R, Liu S, et al. Systemic inflammation and remote organ injury following trauma require HMGB1. Am J Physiol Regul Integr Comp Physiol. 2007; 293:R1538–R1544.


76. Taniguchi N, Kawahara K, Yone K, Hashiguchi T, Yamakuchi M, Goto M, et al. High mobility group box chromosomal protein 1 plays a role in the pathogenesis of rheumatoid arthritis as a novel cytokine. Arthritis Rheum. 2003; 48:971–981.


77. Popovic K, Ek M, Espinosa A, Padyukov L, Harris HE, Wahren-Herlenius M, et al. Increased expression of the novel proinflammatory cytokine high mobility group box chromosomal protein 1 in skin lesions of patients with lupus erythematosus. Arthritis Rheum. 2005; 52:3639–3645.


78. Straino S, Di Carlo A, Mangoni A, De Mori R, Guerra L, Maurelli R, et al. High-mobility group box 1 protein in human and murine skin: involvement in wound healing. J Invest Dermatol. 2008; 128:1545–1553.


79. Mitola S, Belleri M, Urbinati C, Coltrini D, Sparatore B, Pedrazzi M, et al. Cutting edge: extracellular high mobility group box-1 protein is a proangiogenic cytokine. J Immunol. 2006; 176:12–15.


80. Kang R, Livesey KM, Zeh HJ, Loze MT, Tang D. HMGB1: a novel Beclin 1-binding protein active in autophagy. Autophagy. 2010; 6:1209–1211.


81. Kang R, Livesey KM, Zeh HJ 3rd, Lotze MT, Tang D. Metabolic regulation by HMGB1-mediated autophagy and mitophagy. Autophagy. 2011; 7:1256–1258.


82. Huebener P, Gwak GY, Pradere JP, Quinzii CM, Friedman R, Lin CS, et al. High-mobility group box 1 is dispensable for autophagy, mitochondrial quality control, and organ function in vivo. Cell Metab. 2014; 19:539–547.


83. Conti L, Lanzardo S, Arigoni M, Antonazzo R, Radaelli E, Cantarella D, et al. The noninflammatory role of high mobility group box 1/Toll-like receptor 2 axis in the self-renewal of mammary cancer stem cells. FASEB J. 2013; 27:4731–4744.


84. Tye H, Kennedy CL, Najdovska M, McLeod L, McCormack W, Hughes N, et al. STAT3-driven upregulation of TLR2 promotes gastric tumorigenesis independent of tumor inflammation. Cancer Cell. 2012; 22:466–478.


85. Korkaya H, Liu S, Wicha MS. Regulation of cancer stem cells by cytokine networks: attacking cancer's inflammatory roots. Clin Cancer Res. 2011; 17:6125–6129.


86. Liu Z, Bandyopadhyay A, Nichols RW, Wang L, Hinck AP, Wang S, et al. Blockade of Autocrine TGF-β Signaling Inhibits Stem Cell Phenotype, Survival, and Metastasis of Murine Breast Cancer Cells. J Stem Cell Res Ther. 2012; 2:1–8.


87. Schlueter C, Weber H, Meyer B, Rogalla P, Röser K, Hauke S, et al. Angiogenetic signaling through hypoxia: HMGB1: an angiogenetic switch molecule. Am J Pathol. 2005; 166:1259–1263.
88. Lin Q, Yang XP, Fang D, Ren X, Zhou H, Fang J, et al. High-mobility group box-1 mediates toll-like receptor 4-dependent angiogenesis. Arterioscler Thromb Vasc Biol. 2011; 31:1024–1032.


89. van Beijnum JR, Nowak-Sliwinska P, van den Boezem E, Hautvast P, Buurman WA, Griffioen AW. Tumor angiogenesis is enforced by autocrine regulation of high-mobility group box 1. Oncogene. 2013; 32:363–374.


90. Aneja RK, Tsung A, Sjodin H, Gefter JV, Delude RL, Billiar TR, et al. Preconditioning with high mobility group box 1 (HMGB1) induces lipopolysaccharide (LPS) tolerance. J Leukoc Biol. 2008; 84:1326–1334.


91. Robert SM, Sjodin H, Fink MP, Aneja RK. Preconditioning with high mobility group box 1 (HMGB1) induces lipoteichoic acid (LTA) tolerance. J Immunother. 2010; 33:663–671.


92. Choi J, Min HJ, Shin JS. Increased levels of HMGB1 and pro-inflammatory cytokines in children with febrile seizures. J Neuroinflammation. 2011; 8:135.


93. Kissoon N, Carcillo JA, Espinosa V, Argent A, Devictor D, Madden M, et al. World Federation of Pediatric Intensive Care and Critical Care Societies: Global Sepsis Initiative. Pediatr Crit Care Med. 2011; 12:494–503.


94. Li W, Ashok M, Li J, Yang H, Sama AE, Wang H. A major ingredient of green tea rescues mice from lethal sepsis partly by inhibiting HMGB1. PLoS One. 2007; 2:e1153.


95. Ellerman JE, Brown CK, de Vera M, Zeh HJ, Billiar T, Rubartelli A, et al. Masquerader: high mobility group box-1 and cancer. Clin Cancer Res. 2007; 13:2836–2848.


96. Brezniceanu ML, Völp K, Bösser S, Solbach C, Lichter P, Joos S, et al. HMGB1 inhibits cell death in yeast and mammalian cells and is abundantly expressed in human breast carcinoma. FASEB J. 2003; 17:1295–1297.


97. Poser I, Golob M, Buettner R, Bosserhoff AK. Upregulation of HMG1 leads to melanoma inhibitory activity expression in malignant melanoma cells and contributes to their malignancy phenotype. Mol Cell Biol. 2003; 23:2991–2998.

