This article has been retracted. See "Retraction: Hydrogen Peroxide as an Effective Disinfectant for Pasteurella multocida. Yonsei Med J 2014;55:1152-6" in Volume 57 on page 274.
Abstract
Pasteurella multocida (P. multocida) infections vary widely, from local infections resulting from animal bites and scratches to general infections. As of yet, no vaccine against P. multocida has been developed, and the most effective way to prevent pathogenic transmission is to clean the host environment using disinfectants. In this study, we identified which disinfectants most effectively inhibited environmental isolates of P. multocida. Three readily available disinfectants were compared: 3% hydrogen peroxide (HP), 70% isopropyl alcohol, and synthetic phenol. In suspension tests and zone inhibition tests, 3% HP was the most promising disinfectant against P. multocida.
Pasteurella multocida (P. multocida) has been deemed as a zoonotic disease agent by the World Health Organization (WHO 1959). Pasteurellosis is caused by P. multocida and Mannheimia haemolytica and frequently manifests as respiratory infections in small farmed ruminants, which has major worldwide economic and welfare implications.1 It has long been known that P. multocida is a frequent cause of infection following animal bites or scratches.2,3 Recently reported cases of P. multocida infection in Japan have predominantly involved local infections; respiratory infections from sputum of human contact with animals are less common.4,5
No vaccine against this bacterium has been developed thus far.6 Therefore, regular cleaning of the host environment with disinfectants that are inhibitory to microorganism transmission is, currently, the best way to prevent disease induced by this pathogen.7
Traditionally, disinfectants have been classified into five agent types: permeable, dehydrating, sulfhydryl-combining, alkylating, and oxidizing. In this study, three of these were considered "challenged disinfectants": hydrogen peroxide (an oxidizing agent);8,9 alcohol (a dehydrating agent);10,11 and phenol (a permeable agent).
The objective of this study was to determine the most effective disinfectant for the prevention of pathogenic infections by P. multocida. To address this, three readily available disinfectants were tested: 3% hydrogen peroxide (HP), 70% isopropyl alcohol (IA), and synthetic phenol (SP) (0.07% 2-phenylphenol, 0.06% p-tertiary amylphenol). An environmental isolate of P. multocida was obtained from Konkuk University and the following microorganisms were used as representative controls: Bacillus subtilis (spore-forming, gram-positive bacterium); Micrococcus luteus and Pseudomonas aeruginosa (gram-negative bacteria); Candida albicans (yeast); and Aspergillus niger (mold).
For the disinfectant efficacy test, three commercial disinfectants were selected: 3% HP (Sanosil-S006; Sanosil Ltd., CH-8634 Hombrechtikon, Switzerland), SP, and 70% IA. SP was composed of 0.07% 2-phenylphenol and 0.06% p-tertiary amylphenol. In the suspension test, disinfectants at double concentration (for HP and SP) or original concentration (for IA) were diluted with microbial suspensions or tryptone water (Scharlau, Barcelona, Spain) to the final disinfectant concentrations. In the zone inhibition test, each disinfectant was adjusted to its final concentration with sterile hard water (0.442 g/L CaCl2·2H2O) before experimental use. All disinfectants were prepared and used on the same day.
For the suspension test, AFNOR standard NF T 72-151 (in distilled water) and AFNOR standard NF T 72-171 (in the presence of protein) were used as references to measure bactericidal activity, and AFNOR standards NF T 72-201 and NF T 72-231 were used as references for fungicidal and sporicidal activity, respectively.12 The concentrations of microbial suspensions cultured in Tryptone Soya Broth or Sabouraud Chloramphenicol Broth were determined by serial dilution in tryptone water. In the absence of the added protein, 1 mL of the microbial suspension (diluted to 1×108 CFU/mL in distilled water) was added to a tube containing 4 mL of tryptone water, followed by 5 mL of 2×-concentrated disinfectant solution. For 70% IA, the volumes of the microorganisms and disinfectants that were added differed because of the different original concentration (99%). For tests performed in the presence of protein, 1 mL of the microbial suspension containing 1×108 CFU/mL with 0.3% bacteriological milk (Scharlau, Barcelona, Spain) was used. The final cell concentration was 1×107 CFU/mL. Cultures were incubated for 5, 15, and 30 min and 1, 1.5, 2, 2.5, 3, 3.5, 4, 4.5, and 5 h at room temperature (20℃). At each time point, 1 mL of the mixed suspension was dispensed into 100 mL sterile water, filtered using a membrane filtration system (MF System, Millipore, Bedford, MA, USA), and washed three times with sterile water. After filtration, the membrane was incubated for 3-5 days at either 32.5℃ on Tryptone Soya Agar (Scharlau) for vegetative bacteria, bacterial spores, and environmental isolates or at 22.5℃ on Sabouraud Chloram Mod. Agar (Scharlau) for fungi. Colonies were then counted. Counts were recorded for exposure to disinfectant from a minimum of 5 min to a maximum of 5 h. Bactericidal activity, sporicidal activity, and fungicidal activity were expressed as the exposure time needed to achieve a 5-log reduction (for bactericidal and sporicidal activity) or 4-log reduction (for fungicidal activity) in viability, in accordance with AFNOR guidelines.
For the zone inhibition test, 0.1 mL of the P. multocida suspension (1×107 CFU/mL) was spread onto Tryptone Soya Agar plates. Next, filter paper discs (Whatman, Middlesex, UK) with 6-mm diameters were placed on the plates, and then 0.01 mL disinfectant solution was carefully dispensed onto the discs. As a negative control, 0.01 mL hard water was substituted for the disinfectant. The plates were incubated at 32.5℃ for 3-5 days, and the zones of inhibition were measured with vernier calipers (Mitutoyo, Kanagawa, Japan). Each test was repeated three times.
In the zone inhibition test, statistical analyses for susceptibility to disinfectants were conducted using one-way ANOVA with SPSS 12.0.1 for windows (SPSS Inc., Chicago, IL, USA). p-values <0.05 were considered statistically significant.
To identify the disinfectant resistance levels of P. multocida, its viability reduction time in the suspension test was compared with that of other microorganisms. Microorganism suspensions were incubated with each disinfectant solution for 5, 15, and 30 min, and 1, 1.5, 2, 2.5, 3, 3.5, 4, 4.5, and 5 h at room temperature (20.0℃) and then filtered. The filtered membrane was cultured on Tryptone Soya Agar or Sabouraud Chloram Mod. Agar plates for 3-5 days, and the number of colonies was counted in order to find the exposure time required for a 5-log reduction (for vegetative bacteria, bacterial spores and the environmental isolate) or a 4-log reduction (for fungi) of each microorganism, in accordance with AFNOR guidelines. Mixed solution with tryptone water and microorganisms without disinfectants were employed as a positive control, and tryptone water without microorganisms was used as a negative control. To simulate environmental conditions, another experiment was conducted in which 0.3% bacteriological milk was added as an organic material. The suspension test results are summarized in Table 1. Four- or 5-log viability reduction times were recorded for the ATCC-derived bacteria and fungi, including P. aeruginosa, M. luteus, C. albicans, and A. niger. When SP, 70% IA, or 3% HP were used, the reduction times for the ATCC microorganisms ranged from 5 min to 15 min, whereas P. multocida did not show a 4- to 5-log reduction, even after 5 h of exposure, at which time the tests were discontinued. Similar results were obtained with B. subtilis spores. In contrast, when 3% HP was used, a 4- or 5-log viability reduction was achieved in 30 min for P. multocida and in 1.5-2 h for B. subtilis spores. These results were not affected by the addition of milk. These data indicate that P. multocida isolated from the environment exhibits stronger disinfectant resistance than the ATCC microorganisms used in the study, both in the presence and absence of organic material. Furthermore, P. multocida was only slightly less disinfectant-resistant than B. subtilis spores and was susceptible only to 3% HP.
Zone inhibition tests were performed to confirm the effectiveness of 3% HP against P. multocida. The size of the zone of inhibition relative to that of the negative control (hard water) was taken as an index of disinfectant strength (Fig. 1). The inhibition effect of each disinfectant against P. multocida is presented in Fig. 2. The zone of inhibition for 3% HP was significantly larger than that for the other disinfectants. The relative zone sizes were 3.17±0.08 for 3% HP, 1.49± 0.05 for SP, and 1.57±0.04 for IA 70% (p<0.001 vs. negative control). These results confirmed the susceptibility of P. multocida to 3% HP.
The appropriate use of effective disinfectants is very important for preventing the transmission of pathogenic bacteria commonly present in the environment. Particularly at risk of infection are elderly individuals, neonates, and immunocompromised individuals. P. multocida is an example of a pathogenic environmental bacterium, and because no vaccine is yet available against it, the use of appropriate disinfectants is currently the most effective way to prevent infections.
Several steps were taken to make this study relevant for practical applications. First, several readily available disinfectants with different modes of action were tested: 3% HP (oxidant), 70% IA (dehydrant), and SP (permeable denaturant). The disinfectant that was found to be most effective against P. multocida, 3% HP, is inexpensive; therefore, it does not create an economic barrier to P. multocida elimination. Second, a variety of representative microorganisms were used as controls: B. subtilis (spores) and M. luteus (gram-positive bacteria), P. aeruginosa (gram-negative bacteria), C. albicans (yeast), and A. niger (mold). Among the microorganisms tested, P. multocida showed the strongest resistance to disinfectants. Third, 0.3% bacteriological milk was included in the tests as an organic material to simulate more realistic conditions for practical applications. Log viability reduction times were compared with and without the addition of milk; however, no difference was observed. Thus, under realistic conditions, P. multocida was shown to possess the strongest disinfectant resistance among the microorganisms tested; however, it was effectively inhibited by 3% HP.
Bacterial resistance may originate from endogenous genes or may be acquired by mutation or the acquisition of plasmids or transposons.9 Bacterial resistance to disinfectants typically results from cellular changes that impact biocide accumulation.13 It is therefore possible that the disinfectant resistance exhibited in this study by the environmental isolate of P. multocida was the result of alterations by external factors. Further studies are recommended to determine the mechanisms of P. multocida disinfectant resistance. Traditionally, most studies of bacterial disinfectant resistance have analyzed only reference strains from the ATCC; however, the susceptibility of environmental or clinical isolates to disinfectants is now attracting considerable attention.14 According to Herruzo-Cabrera, et al.,15 ATCC strains that are laboratory adapted may not be good predictors of the susceptibility of strains extracted from patients; thus, disinfectant efficacy should be confirmed with recently isolated organisms. It is interesting that the resistance levels of the environmentally isolated P. multocida used in this study were very different from those of the ATCC strains, except for the spore-forming B. subtilis. On the basis of these results, it is recommended that future studies on the efficacy of disinfectants use isolates that are extracted directly from the environment rather than ATCC strains. From our results, it is clear that 3% HP is more effective than SP and 70% IA for preventing growth of the pathogenic P. multocida in the environment.
It is clear that the above-mentioned WHO warning concerning the increase in pasteurellosis is more important today as a consequence of changing patterns in human behavior. Specifically, the acceptance of pets as members of the family has resulted in closer human contact with pets that may be carrying human pathogens. In addition, P. multocida infections in the past were mostly due to animal bites or scratches, but now predominantly involve local infections and, less commonly, respiratory infections. P. multocida is now one of the most important human pathogens.
In conclusion, the results of this study suggest that until an effective vaccine or antibiotic against P. multocida is available, cleaning of hospitals and other public places with hydrogen peroxide as a disinfectant is the most effective and economical way to prevent pasteurellosis.
Figures and Tables
![]() | Fig. 1Results of the zone inhibition test against Pasteurella multocida. The inhibition zone sizes for each disinfectant were measured with vernier calipers. *Significant difference vs. negative control (p<0.001). †The index of disinfectant strength was calculated as the ratio between the mean inhibition zone size (mm) for each disinfectant and that of hard water (negative control). |
![]() | Fig. 2Representative result of a zone inhibition test against Pasteurella multocida. a, hard water (negative control); b, synthetic phenol (0.07% 2-phenylphenol and 0.06% p-tertiary amylphenol); c, 70% isopropyl alcohol; d, 3% hydrogen peroxide. |
Table 1
Summary of the Results of the Suspension Test
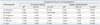
SP, synthetic phenol; HP, hydrogen peroxide; IA, isopropyl alcohol.
*5-log reduction for the ATCC bacteria (B. subtilis, P. aeruginosa, and M. luteus) and the environmental isolate (P. multocida), and 4-log reduction for the ATCC fungi (C. albicans and A. niger).
†Distilled water was used without organic material according to AFNOR standard NF T 72.151.
‡0.3% bacteriological milk as organic material was used according to AFNOR standard NF T 72.171.
§Synthetic phenols (0.07% 2-phenylphenol and 0.06% p-tertiary amylphenol).
∥70% isopropyl alcohol.
¶3% hydrogen peroxide.
References
1. Brogden KA, Lehmkuhl HD, Cutlip RC. Pasteurella haemolytica complicated respiratory infections in sheep and goats. Vet Res. 1998; 29:233–254.
2. Weber DJ, Wolfson JS, Swartz MN, Hooper DC. Pasteurella multocida infections Report of 34 cases and review of the literature. Medicine (Baltimore). 1984; 63:133–154.
3. Hubbert WT, Rosen MN. Pasteurella multocida infections. II. Pasteurella multocida infection in man unrelated to animal bite. Am J Public Health Nations Health. 1970; 60:1109–1117.


4. Arashima Y, Kubo N, Iwasaki Y, Okuyama K, Kumasaka K, Tsuchiya T, et al. [Human respiratory tract infection by Pasteurella multocida subsp. multocida presumably derived from the cat]. Kansenshogaku Zasshi. 1990; 64:1200–1204.


5. Arashima Y, Kumasaka K, Tsuchiya T, Kawano K, Yamazaki E. [Current status of Pasteurella multocida infection in Japan]. Kansenshogaku Zasshi. 1993; 67:791–794.


6. Yun JH, Back HJ, Jin HJ, Son YW, Kwak SY, Yang HJ, et al. Bacterial distribution and antimicrobial resistance pattern of PC room (Internet Cafe) in Seoul, Korea. Korean J Microbiol. 2005; 41:183–187.
7. Cozad A, Jones RD. Disinfection and the prevention of infectious disease. Am J Infect Control. 2003; 31:243–254.
8. Block SS. Disinfection, sterilization and preservation. Philadelphia: Lea & Febiger;1991. p. 167–181.
9. McDonnell G, Russell AD. Antiseptics and disinfectants: activity, action, and resistance. Clin Microbiol Rev. 1999; 12:147–179.


10. Larson EL, Morton HE. Disinfection, sterilization, and preservation. Philadelphia: Lea & Febiger;1991. p. 191–203.
11. HE M. Disinfection, sterilization and preservation. Philadelphia: Lea & Febiger;1983. p. 225–239.
12. Herńndez A, Martró E, Matas L, Martín M, Ausina V. Assessment of in-vitro efficacy of 1% Virkon against bacteria, fungi, viruses and spores by means of AFNOR guidelines. J Hosp Infect. 2000; 46:203–209.


13. Poole K. Mechanisms of bacterial biocide and antibiotic resistance. Symp Ser Soc Appl Microbiol. 2002; (31):55S–64S.

