Abstract
Purpose
This study aimed to investigate useful parameters for estimating gastrocnemius (GCM) muscle volume (MV) using ultrasonography (US) and anthropometry in children with spastic cerebral palsy (CP).
Materials and Methods
Eighteen legs from nine children with spastic CP aged 2 to 6 years were investigated in this study. Tibial length (TL) of each leg was measured and muscle thickness (MT) and anatomical cross-sectional area (aCSA) of GCM muscles were assessed using US. The volume of the GCM was measured by magnetic resonance imaging (MRI) scans. The relationship of TL, MT, and aCSA with MV measured by MRI was investigated. Simple and multiple regression analyses were performed to establish muscle volume prediction equations.
Results
Resting MT, aCSA, and TL were highly related to MV of both medial and lateral head of GCM determined by MRI. The MV prediction equation based on simple regression analysis resulted in r2 values ranging from 0.591 to 0.832 (p<0.05). The r2 values were higher using aCSA as independent variable than using MT. The MV prediction equation based on multiple regression analysis resulted in r2 values ranging from 0.779 to 0.903 (p<0.05). However, the relatively high standard error of the estimate values ranged from 18.0-33.6% on simple regression and 15.5-25.6% on multiple regression. The contribution of aCSA was higher than that of MT for predicting MV of GCM.
Cerebral palsy (CP) describes a group of disorders affecting the development of movement and posture, and thus limiting activity, that are attributed to non-progressive disturbances in the developing fetal or infant brain.1 According to published literature, there is consistent evidence for a reduction of muscle volume (MV) in paretic limbs of children with CP, compared with both non-paretic limbs of children with CP and limbs of typically developing peers.2 Deficits in MV in children with spastic CP may increase as they grow older.3
MV is a good predictor of maximal force generating capacity,4,5,6,7,8,9 and accordingly, the lack of growth in muscle volume may contribute to muscle weakness, which may in turn be related to functional limitations in these children.10,11 A previous report suggested that measurement of muscle size may be an alternative measure of quantitative muscle evaluation for people with severe CP in whom direct measurement of muscle strength is difficult.11 In this context, measurement of MV in a clinical setting would seem to be useful as a means of selection for intervention and for delineating the consequences of interventions, such as botulinum toxin injection or strengthening exercises, on muscle volume.12,13
Previous attempts were made to estimate MV using anthropometric data,14,15 as well as by magnetic resonance imaging (MRI), computed tomography (CT), and ultrasonography (US); CT and MRI are considered the "gold standards" of MV measurement.16,17 More recently, three-dimensional (3D) US was shown to be a valid and reliable measurement of muscle volume.18,19,20 However, two-dimensional brightness mode (2D B-mode) US can be used to produce high-quality images of muscle morphology, and has the same advantages as MRI in distinguishing between muscle and fat tissues.17 Moreover, 2D B-mode US is simple and easy to perform and requires less time for analysis, compared with MRI, CT, and 3D US. Consequently, 2D B-mode US is commonly used to measure muscle size, such as anatomical cross-sectional area (aCSA) and muscle thickness (MT). In the published literature, measurement of aCSA and MT using 2D US exhibited excellent reliability in evaluating children and adolescents with CP.21,22 Additionally, there have been some attempts to estimate MV based on parameters obtained from 2D US scan images.23,24,25,26,27 MV prediction equations have been developed based on MT measurement using US and measured tibial length against muscle weight of cadaveric human legs23,24 or MV measured by MRI in healthy adults.25,26,27 The results of such studies suggest that MT measurement using US is reliable and useful for estimating MV.25,26,27,28
Anatomical CSA of a muscle is one of several muscle architecture parameters that are closely related to maximum muscle strength. According to Blazevich, et al.,6 aCSA is the second-best predictor of maximal muscle torque. However, MV estimation using aCSA measured from a single US scan image has not yet been investigated in children with cerebral palsy.
Equinus foot is the most common deformity in children with spastic CP, and thus, the gastrocnemius (GCM) muscle is the most common target for botulinum toxin injection. The optimal dose of the toxin may be related to MV. Recently, muscle architectural changes after toxin injection have become an interesting issue with respect to the influence of the toxin on growth in muscle volume.29,30,31 Nevertheless, the suitability of 2D B-mode US for MV estimation of the GCM in children with spastic CP has not been investigated. Therefore, the aim of this study was to investigate the application of US and identify useful parameters for the prediction of GCM MV in children with spastic CP.
Children with spastic CP who were admitted or visited our out-patient clinic from January 2010 to May 2012 were recruited for this study upon fulfilling the following inclusion criteria: 1) children with spastic cerebral palsy, 2) Gross Motor Function Classification System (GMFCS) level I to IV, and 3) age of 2 to 6 years. The exclusion criteria were as follows: 1) previous history of GCM muscle trauma, 2) combined with muscular disease, 3) previous history of surgical intervention of lower extremity, 4) any metal implant in the body, or 5) chemodenervation therapy within 6 months.
Ethical approval was granted by the Institutional Review Board and ethics committee of Severance Hospital (# 4-2009-0650). As all the children in this study were younger than 18 years, informed consent was obtained from their parents for participation in the study.
US images of the GCM were taken by a physiatrist using 2D B-mode and real-time ultrasonography (ACCUVIX V10 system, Samsung Medison Co, Seoul, Korea) with a linear-array probe (5 to 12 MHz).
The subjects were positioned prone on the examination table with their feet hanging over the edge of the table. US images over the muscle belly of both medial and lateral heads of GCM muscles were taken in a resting ankle position. For image acquisition, the ultrasound probe was transversely positioned on the skin with minimal compression. US images were obtained following the recommendations of Bénard, et al.32 in order to minimise measurement error. The ultrasound probe was centred on the mid-longitudinal axis, at 25% of the tibial length from the popliteal fossa. Ultrasound images were taken over a range of tilt and rotation angles of the ultrasound probe at 5° increments. Tilt and rotation are expressed as deviations from the longitudinal orientation perpendicular to the skin for all conditions. This was performed three times for each muscle by the same investigator to obtain images of maximal MT.
MT was defined as the longest distance between the upper muscular fascia and the lower muscular fascia visible on the image. Anatomical CSA represents the cross-section perpendicular to the longitudinal axis of a muscle, and is defined as the area surrounded by the upper muscular fascia, the lower muscular fascia, and the intramuscular septum.33 We calculated anatomical CSA using the same image of maximal MT. Fig. 1 shows an example of imaging measurement of MT and aCSA.
The entire GCM muscle was imaged using axial, gapless, T1-weighted sequences. MR images were viewed and processed using OsiriX® software (version 3.6.1, University Hospital of Geneva, Switzerland, 32-bit, http://www.osirix-viewer.com). The MRI images were obtained in the resting ankle position. Volumetry of the GCM muscle was performed manually. A physiatrist manually outlined the junction of the GCM muscle fascia and intermuscular septum, first cranially and then caudally, in the axial image stack. OsiriX® was used to calculate the GCM muscle volume by multiplying all outlined areas of the slices by the slice thickness.8
Univariate analysis was performed to determine the correlation between muscle volume of medial and lateral heads of the GCM and the TL, MT, or aCSA using Pearson correlation analysis. Simple and multiple regression analyses were performed using muscle volume measured by MRI as the dependent variable and [π×(MT/2)2×TL] or (CSA×TL) as the independent variables. Multiple regression analyses were also performed using TL, MT, and aCSA as independent variables. Using the established muscle-volume prediction equations, the muscle volume for medial and lateral heads of the GCM muscle was calculated for each leg. To assess the criterion validity of the equations in predicting the measured muscle volume, the standard error of the estimate (SEE) and validity correlation (r2) were calculated using the measured muscle volume as the criterion. In multiple regression analysis, each independent variable was calculated as an index representing its relative contribution to estimating the measured muscle volume. Contribution was calculated by partial r2. The SEE was calculated using the equation SD diff /√2, where SD diff is the standard deviation of the difference scores between the estimated and measured muscle volume. The SEEs were expressed as absolute values and values relative to the measured muscle volume.
Nine children with bilateral involvement spastic CP (six boys and three girls; mean age±SD, 3.8±1.5 years, range, 2-6 years) were included in this study. The distribution of GMFCS was as follows: Level I (n=5), Level II (n=1), Level III (n=1), and Level IV (n=2). Only one patient had received BoNT-A injection within 6 months before the study.
In total, 18 lower limbs were evaluated. For anthropometric measurements, TL was measured from the lateral knee-joint line to the most prominent point of the lateral malleolus. Mean TL was 19.9±2.7 cm (range 17.1-25.0 cm).
The MV of GCM measured by MRI was 19.70±9.29 cm3 (range; 7.18-38.95 cm3) for the medial head and 11.92±9.12 cm3 (range; 4.14-28.05 cm3) for the lateral head. The MT of GCM muscles measured using US was 0.99±0.27 cm (range; 0.48-1.31 cm) for medial head and 0.82±0.20 cm (range; 0.46-1.17 cm) for lateral head. The aCSA of GCM muscles was 3.05±1.03 cm2 (range; 1.35-4.72 cm2) for medial head and 1.92±0.73 cm2 (range; 0.76-2.98 cm2) for lateral head (Table 1).
Tibial length, MT and aCSA from US imaging, were highly related to MV determined by MRI for both the medial heads (r=0.793, 0.659, 0.810, respectively, p<0.05) and lateral heads (r=0.769, 0.621, 0.824, respectively, p<0.05) of GCM muscles.
In simple regression analyses, [π×(MT/2)2×TL] and (CSA×TL) were significantly correlated with the measured MV for both the medial and lateral heads. Simple regression equations using MT as an independent variable, based on the relationship between MT and measured MV, resulted in values of r2=0.653 (p<0.05) for medial heads and r2=0.591 (p<0.05) for lateral heads. The SEE values in the equations were 5.6 cm2 (28.6%) for medial heads and 4.0 cm2 (33.8%) for lateral heads. The MV prediction equation using aCSA as independent variable resulted in r2=0.862 (p<0.05) for medial heads and r2=0.832 (p<0.05) for lateral heads. The SEE values of the equations were 3.5 cm2 (18.0%) and 2.6 cm2 (21.6%) (Table 2). The relationship between estimated and measured MV using simple regression analysis of children with cerebral palsy is shown in Fig. 2.
Multiple regression analysis revealed that MT, aCSA, and TL were significant contributors to the prediction of MV measured by MRI. The muscle volume prediction equations based on multiple regression analyses are given in Table 3. The final equation based on multiple regression analysis using two independent variables of TL and MT resulted in r2=0.831 (p<0.05) for medial heads and r2=0.779 (p<0.05) for lateral heads. Based on this equation, the relative contribution of MT to the variation in MV measured by MRI was 20.1% for the medial head and 18.8% for the lateral head.
The final equation based on multiple regression analysis using two independent variables of TL and aCSA resulted in r2=0.903 (p<0.05) for medial heads and r2=0.858 (p<0.05) for lateral heads. Based on this equation, the relative contribution of aCSA to the variation in MV measured by MRI was 65.6% for the medial head and 67.8% for the lateral head (Table 3).
In published literature, MV in children with CP was measured using MRI34,35,36 and 3-D US.3,12,21,37 Using these techniques MV can be reliably and validly measured in children with CP. On the other hand, 2D US allows for excellent differentiation between the connective tissue, fat, and muscle tissue, and is also easier to perform and less time-consuming for analysis. Therefore, 2D US is commonly used as a means of assessing muscle size. Previous studies showed that MT measurements obtained using 2D US are useful for estimating MV in different muscle groups.24,26,27 To our knowledge, two studies have tried to estimate MV of ankle plantar flexor from a single 2D US image.24,27 One study investigated the suitability of MT measurement by US for estimation of MV of ankle plantar flexor against MV measured by water displacement of dissected muscles in cadaveric human legs, and the regression between estimated MV and measured MV was r2=0.373 by a simple regression equation and r2=0.497 by a multiple regression equation. However, the results obtained in cadavers may differ from data obtained from living humans because of cell shrinkage resulting from freezing of human tissue, which might account for the discrepancy in their data. On the other hand, Miyatani, et al.27 investigated the accuracy of estimating ankle plantar flexor MV in healthy adults using MT measurement from a single US image, and demonstrated that the regression between MV estimated by a MV prediction equation and that measured by MRI was r2=0.649 by a simple regression equation and r2=0.820 by a multiple regression equation.27 They concluded that MT on US images is useful for estimating ankle plantar flexor MV. The results of our study are in accordance with those of Miyatani, et al.,27 and confirmed that MT on 2D US is a useful parameter when combined with TL for estimating GCM MV in children with spastic CP.
The functional importance of changes in the aCSA of a muscle can be seen in its contribution to total force production,38 and aCSA is therefore often analysed in studies of strength training, in which it shows a strong relationship with the maximum capacity of muscle force production.4,39,40 In the literature, aCSA measured using 2D B-mode US scanning shows a high correlation with other imaging techniques such as MRI or CT.16,25,41 Therefore, US has been commonly used to measure human skeletal muscle aCSA in vivo in different subjects and different muscles.25,40,42 For children and adolescents with CP, aCSA measurement using 2D US had excellent reliability.21 Using this technique, aCSA of large muscles can be reliably and validly measured using 2D US.17 We measured aCSA of each head of GCM muscles from routine single transverse plane US images without use of the panoramic scanning technique because the aCSA of each head of the GCM muscles can be shown in whole in young children. In multiple regression equations using measured aCSA and TL, the r2 values were 0.903 for medial heads and 0.858 for lateral heads. These high values of r2 suggest the usefulness of aCSA measurement for predicting MV. According to a previous study, estimations of MV from the aCSA of the tibialis anterior measured by 11 axial US scans were highly reliable and valid when compared with MV measured by MRI.25 In addition, the study of Morse, et al.43 demonstrated that aCSA from a single MRI scan was highly correlated with the MV of quadriceps measured by 11 continuous transverse plane MRI scans along the entire length of the femur. These findings suggest that aCSA may be useful for estimating MV. Compared with MRI and the multiple axial US scans used in previous studies, aCSA measurements from a single US image are easier to perform and access, and also require much less time for analysis. In this context, the clinical implication of the MV prediction equation obtained in our study is noteworthy. As far as we are aware, this is the first study to show the suitability of aCSA measurements using 2D US for estimating MV in children with CP.
Nevertheless, the relatively high SEE values, ranging from 18.0-33.6% in simple regression and 15.5-25.6% in multiple regression, suggest a limitation in the MV prediction equation for use in individual assessment in longitudinal studies. To reduce the SEE values, increasing the number of levels of measurement and standardisation of US imaging with respect to the ankle joint position, the severity of spasticity and ankle joint limitations in motion should be taken into consideration. Further studies are needed to find the most efficient and accurate way to estimate MV for individual assessment in longitudinal studies.
In conclusion, MV prediction equations using MT and aCSA of GCM muscles measured by 2D US combined with TL were obtained based on MV of GCM measured by MRI in children with spastic CP. The high values of r2 in each multiple regression MV prediction equation obtained in our study indicate that MV estimates using MT or aCSA measurement using 2D US are suitable when combined with tibial length. In addition, aCSA is more useful than MT for estimating the MV of GCM. The final MV prediction equations obtained in our study may provide a quick and easy way to estimate the volume of GCM muscles in these children, which may ultimately be useful for population studies or field surveys. However, at present the SEE values observed in our study indicate that it might be better to limit use of these prediction equations to individual assessment in longitudinal studies. Further attempts to improve the accuracy of MV estimation using 2D US are needed.
Figures and Tables
Fig. 1
Ultrasonographic measurement of muscle thickness and anatomical cross-sectional area. Muscle thickness (MT): the longest distance between the upper muscular fascia and the lower muscular fascia. Anatomical cross-sectional area (aCSA): the area surrounded by the upper muscular fascia, the lower muscular fascia, and the intramuscular septum.
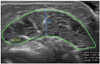
Fig. 2
Relationship between estimated (based on ultrasound and arthropometry) and measured (MRI) muscle volume for gastrocnemius muscle, using simple and multiple regression analyses. (A) y1=0.868×TL×π(MT/2)2+5.112, r2=0.653 (p=0.000), SEE=5.645 cm3 (28.6%). (B) y1=0.332×TL×CSA-0.958, r2=0.862 (p=0.000), SEE=3.559 cm3 (18.0%). (C) y2=0.802×TL×π(MT/2)2+2.641, r2=0.591 (0.000), SEE=4.1 cm3 (33.8%). (D) y2=0.298×TL×CSA+0.203, r2=0.832 (p=0.000), SEE=2.6 (21.6%). (E) Y1=2.271×TL+15.982×MT-41.493, r2=0.831 (0.001), SEE=4.1 (20.6%). (F) Y1=1.896×TL+5.192×CSA-34.050, r2=0.903 (0.000), SEE=3.1 (15.5%). (G) Y2=1.479×TL+13.347×MT-28.676, r2=0.779 (0.003), SEE=3.1 (25.6%). (H) Y2=1.101×TL+4.903×CSA-19.516, r2=0.858 (0.001), SEE=2.4 (20.5%). TL, tibial length; MT, muscle thickness; CSA, cross-sectional area; SEE, standard error of the estimate.
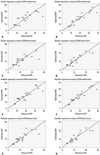
References
1. Bax M, Goldstein M, Rosenbaum P, Leviton A, Paneth N, Dan B, et al. Proposed definition and classification of cerebral palsy, April 2005. Dev Med Child Neurol. 2005; 47:571–576.


2. Barrett RS, Lichtwark GA. Gross muscle morphology and structure in spastic cerebral palsy: a systematic review. Dev Med Child Neurol. 2010; 52:794–804.


3. Barber L, Hastings-Ison T, Baker R, Barrett R, Lichtwark G. Medial gastrocnemius muscle volume and fascicle length in children aged 2 to 5 years with cerebral palsy. Dev Med Child Neurol. 2011; 53:543–548.


4. Fukunaga T, Miyatani M, Tachi M, Kouzaki M, Kawakami Y, Kanehisa H. Muscle volume is a major determinant of joint torque in humans. Acta Physiol Scand. 2001; 172:249–255.


5. Fukunaga T, Ito M, Ichinose Y, Kuno S, Kawakami Y, Fukashiro S. Tendinous movement of a human muscle during voluntary contractions determined by real-time ultrasonography. J Appl Physiol (1985). 1996; 81:1430–1433.


6. Blazevich AJ, Coleman DR, Horne S, Cannavan D. Anatomical predictors of maximum isometric and concentric knee extensor moment. Eur J Appl Physiol. 2009; 105:869–878.


7. Gadeberg P, Andersen H, Jakobsen J. Volume of ankle dorsiflexors and plantar flexors determined with stereological techniques. J Appl Physiol (1985). 1999; 86:1670–1675.


8. Akagi R, Takai Y, Ohta M, Kanehisa H, Kawakami Y, Fukunaga T. Muscle volume compared to cross-sectional area is more appropriate for evaluating muscle strength in young and elderly individuals. Age Ageing. 2009; 38:564–569.


9. Pitcher CA, Elliott CM, Williams SA, Licari MK, Kuenzel A, Shipman PJ, et al. Childhood muscle morphology and strength: alterations over six months of growth. Muscle Nerve. 2012; 46:360–366.


10. McBurney H, Taylor NF, Dodd KJ, Graham HK. A qualitative analysis of the benefits of strength training for young people with cerebral palsy. Dev Med Child Neurol. 2003; 45:658–663.


11. Ohata K, Tsuboyama T, Ichihashi N, Minami S. Measurement of muscle thickness as quantitative muscle evaluation for adults with severe cerebral palsy. Phys Ther. 2006; 86:1231–1239.


12. McNee AE, Gough M, Morrissey MC, Shortland AP. Increases in muscle volume after plantarflexor strength training in children with spastic cerebral palsy. Dev Med Child Neurol. 2009; 51:429–435.


13. Tok F, Ozçakar L, Safaz I, Alaca R. Effects of botulinum toxin-A on the muscle architecture of stroke patients: the first ultrasonographic study. J Rehabil Med. 2011; 43:1016–1019.


14. Heymsfield SB, McManus C, Smith J, Stevens V, Nixon DW. Anthropometric measurement of muscle mass: revised equations for calculating bone-free arm muscle area. Am J Clin Nutr. 1982; 36:680–690.


15. Rice CL, Cunningham DA, Paterson DH, Lefcoe MS. A comparison of anthropometry with computed tomography in limbs of young and aged men. J Gerontol. 1990; 45:M175–M179.


16. Reeves ND, Narici MV, Maganaris CN. Effect of resistance training on skeletal muscle-specific force in elderly humans. J Appl Physiol (1985). 2004; 96:885–892.


17. Ahtiainen JP, Hoffren M, Hulmi JJ, Pietikäinen M, Mero AA, Avela J, et al. Panoramic ultrasonography is a valid method to measure changes in skeletal muscle cross-sectional area. Eur J Appl Physiol. 2010; 108:273–279.


18. Linney AD, Deng J. Three-dimensional morphometry in ultrasound. Proc Inst Mech Eng H. 1999; 213:235–245.


19. Fenster A, Downey DB. Three-dimensional ultrasound imaging. Annu Rev Biomed Eng. 2000; 2:457–475.


20. Barber L, Barrett R, Lichtwark G. Validation of a freehand 3D ultrasound system for morphological measures of the medial gastrocnemius muscle. J Biomech. 2009; 42:1313–1319.


21. Moreau NG, Teefey SA, Damiano DL. In vivo muscle architecture and size of the rectus femoris and vastus lateralis in children and adolescents with cerebral palsy. Dev Med Child Neurol. 2009; 51:800–806.


22. Mohagheghi AA, Khan T, Meadows TH, Giannikas K, Baltzopoulos V, Maganaris CN. In vivo gastrocnemius muscle fascicle length in children with and without diplegic cerebral palsy. Dev Med Child Neurol. 2008; 50:44–50.


23. Infantolino BW, Gales DJ, Winter SL, Challis JH. The validity of ultrasound estimation of muscle volumes. J Appl Biomech. 2007; 23:213–217.


24. Bandholm T, Sonne-Holm S, Thomsen C, Bencke J, Pedersen SA, Jensen BR. Calf muscle volume estimates: implications for botulinum toxin treatment? Pediatr Neurol. 2007; 37:263–269.


25. Esformes JI, Narici MV, Maganaris CN. Measurement of human muscle volume using ultrasonography. Eur J Appl Physiol. 2002; 87:90–92.


26. Miyatani M, Kanehisa H, Kuno S, Nishijima T, Fukunaga T. Validity of ultrasonograph muscle thickness measurements for estimating muscle volume of knee extensors in humans. Eur J Appl Physiol. 2002; 86:203–208.


27. Miyatani M, Kanehisa H, Ito M, Kawakami Y, Fukunaga T. The accuracy of volume estimates using ultrasound muscle thickness measurements in different muscle groups. Eur J Appl Physiol. 2004; 91:264–272.


28. Miyatani M, Kanehisa H, Fukunaga T. Validity of bioelectrical impedance and ultrasonographic methods for estimating the muscle volume of the upper arm. Eur J Appl Physiol. 2000; 82:391–396.


29. Choi WH, Song CW, Kim YB, Ha CS, Yang GH, Woo HD, et al. Skeletal muscle atrophy induced by intramuscular repeated dose of botulinum toxin type A in rats. Drug Chem Toxicol. 2007; 30:217–227.


30. Cosgrove AP, Corry IS, Graham HK. Botulinum toxin in the management of the lower limb in cerebral palsy. Dev Med Child Neurol. 1994; 36:386–396.


31. Williams SA, Reid S, Elliott C, Shipman P, Valentine J. Muscle volume alterations in spastic muscles immediately following botulinum toxin type-A treatment in children with cerebral palsy. Dev Med Child Neurol. 2013; 55:813–820.


32. Bénard MR, Becher JG, Harlaar J, Huijing PA, Jaspers RT. Anatomical information is needed in ultrasound imaging of muscle to avoid potentially substantial errors in measurement of muscle geometry. Muscle Nerve. 2009; 39:652–665.


33. e Lima KM, da Matta TT, de Oliveira LF. Reliability of the rectus femoris muscle cross-sectional area measurements by ultrasonography. Clin Physiol Funct Imaging. 2012; 32:221–226.


34. Lampe R, Grassl S, Mitternacht J, Gerdesmeyer L, Gradinger R. MRT-measurements of muscle volumes of the lower extremities of youths with spastic hemiplegia caused by cerebral palsy. Brain Dev. 2006; 28:500–506.


35. Williams SA, Elliott C, Valentine J, Gubbay A, Shipman P, Reid S. Combining strength training and botulinum neurotoxin intervention in children with cerebral palsy: the impact on muscle morphology and strength. Disabil Rehabil. 2013; 35:596–605.


36. Riad J, Modlesky CM, Gutierrez-Farewik EM, Broström E. Are muscle volume differences related to concentric muscle work during walking in spastic hemiplegic cerebral palsy? Clin Orthop Relat Res. 2012; 470:1278–1285.


37. Fry NR, Gough M, McNee AE, Shortland AP. Changes in the volume and length of the medial gastrocnemius after surgical recession in children with spastic diplegic cerebral palsy. J Pediatr Orthop. 2007; 27:769–774.


38. Morse CI, Thom JM, Reeves ND, Birch KM, Narici MV. In vivo physiological cross-sectional area and specific force are reduced in the gastrocnemius of elderly men. J Appl Physiol (1985). 2005; 99:1050–1055.


39. Masuda K, Kikuhara N, Takahashi H, Yamanaka K. The relationship between muscle cross-sectional area and strength in various isokinetic movements among soccer players. J Sports Sci. 2003; 21:851–858.


40. Bemben MG. Use of diagnostic ultrasound for assessing muscle size. J Strength Cond Res. 2002; 16:103–108.


41. Noorkoiv M, Nosaka K, Blazevich AJ. Assessment of quadriceps muscle cross-sectional area by ultrasound extended-field-of-view imaging. Eur J Appl Physiol. 2010; 109:631–639.

