Abstract
Purpose
Vaccine strategies utilizing dendritic cells (DCs) to elicit anti-tumor immunity are the subject of intense research. Although we have shown that DCs pulsed with heat-treated tumor lysate (HTL) induced more potent anti-tumor immunity than DCs pulsed with conventional tumor lysate (TL), the underlying molecular mechanism is unclear. In order to explore the molecular basis of this approach and to identify potential antigenic peptides from pancreatic cancer, we analyzed and compared the major histocompatibility complex (MHC) ligands derived from TL- and HTL-pulsed dendritic cells by mass spectrophotometry.
Materials and Methods
Human monocyte-derived dendritic cells were pulsed with TL or HTL prior to maturation induction. To delineate differences of MHC-bound peptide repertoire eluted from DCs pulsed with TL or HTL, nanoflow liquid chromatography-electrospray ionization-tandem mass spectrometry (nLC-ESI-MS-MS) was employed.
Results
HTL, but not TL, significantly induced DC function, assessed by phenotypic maturation, allostimulation capacity and IFN-γ secretion by stimulated allogeneic T cells. DCs pulsed with TL or HTL displayed pancreas or pancreatic cancer-related peptides in context of MHC class I and II molecules. Some of the identified peptides had not been previously reported as expressed in pancreatic cancer or cancer of other tissue types.
Conclusion
Our partial lists of MHC-associated peptides revealed the differences between peptide profiles eluted from HTL-and TL-loaded DCs, implying that induced heat shock proteins in HTL chaperone tumor-derived peptides enhanced their delivery to DCs and promoted cross-presentation by DC. These findings may aid in identifying novel tumor antigens or biomarkers and in designing future vaccination strategies.
Dendritic cell-based tumor immunotherapy became a promising approach in the past decade by the generation of large numbers of immunopotent dendritic cells (DCs) in vitro.1,2 Furthermore, identification of major histocompatibility complex (MHC) class I-associated tumor antigens that can prime antigen-specific CD8 T cells has the potential to facilitate tumor-targeted DC-based vaccine strategies for systemic cytotoxic T lymphocyte (CTL) responses.3,4 While these studies analyzed the efficacy of antigen cross-presentation by DC to CD8+ T cells via MHC class I molecules, optimal systemic anti-tumor immunity and immunological memory involves priming of both CD4+ and CD8+ T cells recognizing tumor-associated antigens.5,6 MHC class II-restricted activation of antigen-specific CD4+ helper T cells are essential for DC maturation that is functionally competent to prime naïve CD8+ T cells.7,8,9,10 Thus, utilization of DC as successful tumor vaccines requires both MHC class I and II-restricted epitopes.
However, the limited availability of tumor-specific MHC class I and II-restricted peptide antigens is still one of the major obstacles.11,12 At present, the great majority of tumors, including pancreatic cancer, are beyond the realm of peptide-based immunotherapy. Accordingly, researchers explored an alternative approach to DCs loaded with peptides by using DCs loaded tumor lysate as a source of tumor antigens to induce protective antitumor responses.13,14 While DCs loaded with tumor lysates exhibited modest protective immunity in various animal tumor models,15,16 and provided limited objective clinical responses in a number of clinical trials,17,18,19 some concerns have been raised about the relative immunogenicity of different modes of tumor lysate preparation.20,21,22,23 Previously, we and others found that heat-treated tumor lysate (HTL) was a better approach for antigen-delivery and tumor immunity compared to conventional tumor lysate (TL) in inducing CD8+ T cells to differentiate into cytotoxic T cells in vitro as well as in vivo murine pancreatic cancer,24 colon cancer,25 or medullary thyroid carcinoma.26 Heat treatment is known to enhance the immunogenicity of tumor cells, which is in part ascribed to heat shock proteins (HSPs), and HSP-chaperoned proteins and peptides released from tumor cells may target DCs with the assistance of HSP receptors and can be taken up by antigen presenting cells (such as dendritic cells and macrophages) through receptor-mediated endocytosis.27 While this approach shares key aspects of tumor lysate in antigen diversity as well as polyclonal CD4 and CTL responses, heat-treated tumor lysate provides additional advantages in stimulating DCs during antigen delivery and possibly in expanded repertoire of presented antigens. Although enhanced delivery of more diverse antigen by this strategy was speculated, quantitative or qualitative analysis of antigen profiles on MHC molecules upon application of this approach has not been performed.
In the present study, we showed that HTL significantly enhanced maturation of DCs through upregulation of antigen-presenting molecules as well as costimulatory molecules. Furthermore, we found that there are qualitative differences in profiles of antigenic peptides eluted from DCs pulsed with TL or HTL from same cell sources by the power of tandem mass spectrometry. While we detected several over-presented peptides in cancer cells, some peptides were identified only in HTL, indicating that HSPs facilitate transfer of specific sets of antigenic peptides onto MHC molecules of DCs. The approach described herein provides a powerful identification method of naturally processed tumor-associated peptides that can aid to formulate tumor-specific vaccines for clinical use.
Panc-1 (human pancreatic ductal adenocarcinoma cell line) and NCI-N87 (human gastric carcinoma) obtained from American Type Culture Collection (Manassas, VA, USA) were maintained in RPMI 1640 supplemented with 20 mM HEPES, pH 7.2, 1 mM sodium pyruvate, 2 mM glutamine, and 10% heat-inactivated fetal calf serum (Life Technologies, Grand Island, NY, USA). The recombinant human cytokines (GM-CSF, IL-4, IL-1β, IL-6, and TNF-α) were purchased from Peprotech (Rocky Hill, NJ, USA) and PGE2 was from Sigma Chemicals (St. Louis, MO, USA).
TL was prepared according to the protocol described by Schnurr, et al.15 Briefly, Panc-1 cells at 90% confluency were digested with 0.02% Trypsin-EDTA and washed once with PBS. After cell counting, cells resuspended in serum-free medium were disrupted by 4 freeze (liquid nitrogen) and thaw (37℃ water bath) cycles. Large particles were removed by centrifugation (10 min, 500×g), and supernatants were passed through a 0.2 µm syringe filter (Pall Corp, Ann Arbor, MI, USA). The protein content of the lysate was determined and aliquots were stored at -80℃. For the HTL generation, Panc-1 cells at 70% confluency were heat-treated for 2 hr at 42℃. Cells were allowed to recover for 24 hr at 37℃ prior to detachment and lysate preparation. Lysates were tested for bacterial endotoxin contamination with the Limulus amoebocyte lysate assay according to manufacturer's instruction (Charles River Endosafe, Charleston, SC, USA) and found to contain less than 0.01 EU/µg protein.
Samples of TL and HTL were separated by 10% SDS-PAGE and transferred onto Immobilin PVDF membrane (Millipore, Bedford, CA, USA). After blocking with blocking reagent (Roche Diagnostics, Manheim, Germany), HSPs were detected using antibodies against HSP70, HSC-70, HSP90, and gp96 (StressGen Biotechnologies, Victoria, Canada), followed by anti-mouse IgG HRP (Santa Cruz Biotechnology, Santa Cruz, CA, USA). Specific bands were developed using ECL (Amersham Biosciences, Buckinghamshire, UK).
All human subjects participated in this study after providing informed consent that was reviewed and approved by the Internal Review Board of Yonsei University College of Medicine. Peripheral blood mononuclear cells (PBMC) were isolated from heparinized venous blood of healthy volunteers by using Ficoll-Hypaque density centrifugation. Monocytes were isolated using CD14 microbeads (Miltenyi Biotech, Auburn, CA, USA), and DCs were differentiated from monocytes as described.28 Briefly, monocytes were cultured in complete RPMI 1640 medium containing GM-CSF (100 ng/mL) and IL-4 (10 ng/mL). On day 6, cells were incubated with TL or HTL for 4 hr. Lysate pulsing was stopped by changing the medium. Subsequently, DC maturation was induced for additional 48 hr by adding a cytokine cocktail consisting of TNF-α (10 ng/mL), IL-1β (10 ng/mL), IL-6 (1000 U/mL), and 10-7 M PGE2.
The phenotype of DCs was examined at the start of the culture and after DC maturation (day 8). Monoclonal antibodies against the following antigens were used: CD1a, CD83, CD86, HLA-DR, CD40, and CD80 (all from BD Biosciences, San Diego, CA, USA). Cells were collected and analyzed using Cytomics™ Flow Cytometer (Beckman Coulter, Fullerton, CA, USA). Non-relevant antibodies of recommended isotypes were included as controls. Data analysis was performed by WinMDI (Scripps Institute, La Jolla, CA, USA) or CXP and FCS 3.0 software for FC500 (Beckman Coulter). Cells were electronically gated according to light-scatter properties to exclude cell debris or dead cells stained with propidium iodide (Sigma). Results were expressed as median fluorescence intensity.
DCs were harvested, washed, and resuspended in RPMI 1640+10% FBS. A total of 2×105 allogeneic T cells were incubated with irradiated DCs (30 Gy) at different responder/stimulator ratios ranging between 10:1 and 1280:1 in 96-well flat-bottom plates. After 4 days of coculture, the cells were pulsed with [3H] thymidine (1 µCi/mL final concentration) and incorporation was measured after 16 hr. Responses were reported as mean of triplicate counts per min (cpm)±SEM.
A parallel set of cultures was used to determine IFN-γ expression. After 5 days of cultures at 10:1 responder/stimulator ratio of T cells (1×106 cells/mL), T cells were harvested, washed extensively and expanded with IL-2 (10 U/mL, Peprotech) for 6 days. On day 11, T cells (5×105 cells/mL) were harvested and restimulated with IL-2 (10 U/mL) for 24 hr on 24-well plates coated with anti-CD3 antibody (1 µg/mL, BD Pharmingen, San Diego, CA, USA). Samples were assayed in triplicates. The culture supernatants were collected and frozen at -20℃ until measurement.
IL-12 (p70) and IFN-γ expression levels were determined by a sandwich ELISA assay (OptEIA, BD Biosciences, San Diego, CA, USA). For IL-12 measurement, the cell-free supernatants from immature DCs (day 6) or DCs pulsed with tumor lysate (TL or HTL) and matured by cytokine cocktail for 48 hr were collected. Samples were assayed in triplicates. The assays were carried out according to the instructions provided by the manufacturer. The analysis of significance was carried out using unpaired Student t-tests or one-way ANOVA.
Genomic DNA was extracted from cultured dendritic cells using QIAamp DNA mini kit (Qiagen, Valencia, CA, USA). DNA-based HLA typing was performed by Luminex Multi-Analyte Profiling system (xMAP) with a WAKFlow HLA typing kit (Wakunaga, Hiroshima, Japan). Briefly, the highly polymorphic exons 2 and 3 of HLA-A, -B, and -C genes and exon 2 of HLA-DRB1 were amplified by polymerase chain reaction (PCR) with biotinylated primers. The PCR product was hybridized with probes immobilized on fluorescent coded microsphere beads. At the same time, biotinylated PCR product was labeled with streptavidin-conjugated R-phycoerythrin and immediately examined with the Luminex (Luminex, Austin, TX, USA). Genotype determination and data analysis were performed automatically using the WAKFlow Typing software according to the manufacturer's instructions.
Peptides were extracted from the surface of 1×107 cells of TL-DC or HTL-DC pelleted in a 15-mL polypropylene tube (Falcon, Oxnard, CA, USA) as described by Santin, et al.29 with minor modifications. Briefly, 2 mL of citrate-phosphate buffer was added directly to pelleted cells and incubated for 5 min at room temperature. After centrifugation at 500×g for 3 min, supernatant was collected and further clarified by centrifugation at 1800×g for 5 min at 4℃. Peptides were stored at -80℃ until further processing.
Each MHC-associated peptide eluted from TL and HTL was purified using an Oasis HLB cartridge (Waters, Milford, MA, USA), lyophilized using a Modulspin 40 from BioTron (Seoul, Korea), and sequentially resuspended in 100 µL of 2% CH3CN in water for nLC-ESI-MS-MS analysis. Peptides were stored at -80℃ before use.
The nanoflow LC-ESI-MS-MS system utilized was CapLC equipped with Q-TOF Ultima mass spectrometer (Waters, Milford, MA, USA). For nanoflow reversed phase liquid chromatography (nRPLC or nLC), eluted peptides from TL-DC or HTL-DC was separated using a homemade pulled tip capillary column [75 µm inner diameter (i.d.)×360 µm outer diameter (o.d.)×15 cm long] which was packed with a methanol slurry of Magic C18AQ (3 µm, 100 Å) resins from Michrom BioResources Inc. (Auburn, CA, USA) in our laboratory. Prior to RPLC run, each peptide sample was first loaded to a trapping column (200 µm i.d.×360 µm o.d.×1.0 cm long) for on-line sample desalting. The trapping column was prepared by packing Magic C18AQ (5 µm, 200 Å) resins in capillary tubing ended with frit (2 mm in length) which was made by a sol-gel preparation. The trapping column and analytical column were connected via a PEEK microcross, and Pt wire was used as an electrode to supply electric voltage for the electrospray ionization as described in literature.30
A 10 µL aliquot of each eluent from TL-DC or HTL-DC was injected via autosampler to the trapping column, followed by a binary gradient separation as previously reported.31 Briefly, 2% acetonitrile (buffer A) and 95% cetonitrile in water containing 0.1% formic acid (buffer B) were used for binary gradient nLC runs. The flow rate for nLC run was adjusted to 200 nL/min and binary gradient run conditions were as follows: buffer B increased from 2 to 15% for 15 min, then linearly increased to 12% over 10 min, to 32% over 60 min, ramped to 80% over 3 min, and then it was held at 80% for 10 min to clean the RP column. Finally, buffer B was decreased to 2% and was held for 20 min to re-equilibrate the column. Eluted peptides from the analytical capillary column were directly introduced into MS via electrospray ionization (ESI) in positive ion mode at an ESI voltage of 1.8 kV.
MS analysis of MHC-peptide ions was carried out with a precursor scan (300-1800 atomic mass unit, amu) followed by 3 data-dependent MS-MS scans. Collected MS-MS spectra of peptides obtained from collision-induced dissociation experiment were analyzed using the Mascot Search program against NCBI human databases. The mass tolerance between measured monoisotopic mass and calculated mass was 1.0 amu for precursor ions and 0.5 amu for fragment ions. Peptides were accepted only if a MOWSE score higher than 50 and E-value less than 0.05 were satisfied when screening search data. In addition, no fixed and variable modifications and no enzymatic cleavage were selected, including doubly miss cleavages.
Tumor cell lysate potentially containing multiple tumor-associated antigens are most frequently exploited for DC-based tumor immunotherapy, particularly in cases where suitable tumor-associated antigens are not defined. In our previous study, we showed that DCs pulsed with tumor lysate preparation after heat treatment (HTL) significantly enhanced the antitumor immune responses compared to that of TL. One possible explanation for the superiority of HTL to their counterpart (TL) in transferring antigens onto DC in vitro as well as in antitumor immunity in vivo is the fact that HSPs are involved. HSPs are molecular chaperone of nascent polypeptides including antigenic peptides generated inside the cell. Upon release from dying cells, these peptide-bound HSPs are recognized and endocytosed by antigen presenting cells (such as DCs) via receptor-mediated endocytosis. The molecular interaction has been reported to stimulate antigen presenting cells to secrete proinflammatory cytokines and mediate DC maturation.32 To verify the expression of HSPs resulting from heat-treatment of Panc-1 cells, TL and HTL were subjected to immunoblot analysis. For heat treatment, Panc-1 and N87 cells at 70% confluency were incubated for 1 hr at 42℃. Upon culture for 24 hr at 37℃, cells were evaluated for HSP expression, using antibodies against the most prominently studied HSPs (Fig. 1). Expression of inducible HSP70, gp96, and HSP90 shown to elicit antitumor immunity32 increased dramatically in HTL, while the expression of HSC70 remained relatively unchanged. These data indicate that HTL of Panc-1 contains inducible HSP families that may play a pivotal role in augmenting tumor antigenicity.
To examine whether HTL might affect the expression of surface markers involved in maturation, immature DCs (iDCs) were incubated with TL, HTL (at 100 µg/mL), or media for 4 hr. After pulsing, iDCs were cultured with GM-CSF+IL-4 in the presence or absence of cytokine cocktail (TNF-α, IL-1β, IL-6, and PGE2) for an additional 48 hr to assess the effect of tumor lysate on DC maturation. Finally, nonadherent cells were harvested, stained with antibodies against MHC class II molecules (HLA-DR), CD1a, CD83, CD86, CD40, and CD80 (Fig. 2). For clarity, mean fluorescence intensities of each staining are shown in Fig. 2. The cytokine cocktail induced DC maturation as evident from up-regulation of CD83, HLA-DR, costimulatory molecules (CD80 and CD86), and CD40 as compared to controls. Surface expression of HLA-DR and CD86 was upregulated 2- and 2.1-folds. When cultured in the presence of HTL, even iDC showed signs of maturation, i.e., there was increased expression of HLA-DR (18.6%), CD86 (8.8%), and CD40 (17.7%) on cell surfaces. While TL enhanced the expression of HLA-DR (10.5%), CD40 (7.1%), CD83 (15.6%), and CD80 (12.0%) only marginally, the expression of CD86 (-9.9%) was significantly downregulated by TL. When DCs were stimulated with cytokine cocktail upon lysate loading, the effect of HTL on the expression of maturation markers were more significant. While HTL upregulated the expression of HLA-DR (34.3%), CD40 (9.4%), CD83 (43.0%), CD86 (12.7%), and CD80 (11.9%), TL reduced the expression of HLA-DR (-28.8%), CD86 (14.9%), and CD80 (18/8%). These results imply that TL treatment of DCs decreases T cell activation capacity of either iDC or mature DC (mDC). Yields and viabilities of mDCs were not significantly different from each other (data not shown).
To evaluate the allostimulatory capacities of DCs loaded with TL or HTL, we performed allogeneic mixed leukocyte reaction assay. As shown in Fig. 3A, HTL-loaded iDCs led to stronger allogeneic T cell proliferation compared to that of unpulsed iDCs. Enhanced proliferation of responder T cells was observed in all DC/T cell ratios tested. While allostimulation by TL-loaded iDCs was comparable only to iDCs, DC loaded with HTL and matured with cytokine cocktail (HTL-DCs) were more effective in stimulating a proliferative response than that with TL-DC or control. There was no significant difference in allostimulatory capacity between mDC (control) or TL-mDC. These data show that HTL is more effective in inducing phenotypic and functional maturation of dendritic cells than TL, suggesting that the function of enriched HSPs in HTL may be involved in the induction of DC maturation.
Since IL-12 plays a crucial role in Th1 priming, we then examined the level of IL-12 production by DCs pulsed with TL or HTL. While iDC produced low level of IL-12, maturation with proinflammatory cytokine consistently increased IL-12 production. The production of IL-12 was significantly enhanced in HTL-DC compared to that of unpulsed DC, while no significant difference between TL-DC and HTL-DC was observed (Fig. 3B). We further evaluated the nature of primary allogeneic T cell responses stimulated by mature unpulsed DC, TL-DC and HTL-DC. In line with IL-12 production capacity, HTL-DC significantly stimulated the secretion of IFN-γ from allogeneic T cells compared to that of TL-DC and unpulsed DC (Fig. 3C).
To rule out the possibility that the capacity of tumor lysate to induce DC maturation was due to mycoplasma contamination, as proposed by others,33 lysates were tested for the presence of different mycoplasma strains and proved to be negative (data not shown).
For the identification of MHC-restricted potential tumor antigens as well as tumor biomarkers on DCs pulsed with tumor antigens, we utilized a MHC-associated peptide proteomics that allows the identification of HLA-restricted peptides on as little as 1×106 dendritic cells. Thus, we exposed dendritic cells to TL or HTL derived from Panc-1 cells and stimulated them with the cytokine cocktail. Dendritic cells were allowed to ingest tumor lysate for 48 hr, whereupon HLA-bound peptides were eluted from dendritic cells with mild acid and analyzed by nLC-ESI-MS-MS.
Furthermore, to explore whether there are any qualitative differences between TL-DC and HTL-DC, the whole HLA-associated peptide repertoire of DCs was investigated. Thus, MHC-associated peptides were sequentially filtered through a 10-kDa cut-off device, and fractionated by RPLC on a capillary C18 column. Fig. 4 shows base peak chromatograms (BPCs) on MHC-associated peptides derived from TL-DC and HTL-DC. The 2 BPCs appear to be significantly different from each other, indicating that HTL-DC displays distinct-peptide repertoire compared to that of TL-DC. HLA genotype of the DCs employed for the peptide elution in the present study was HLA-A*2402, 2901, B*0705, 2705, Cw*0102, 1505, and DRB1*0101, 0803.
Table 1 shows a list of peptides that were present on TL-DC and HTL-DC. Among 200 identified peptides, the list include only those present in 2 independent measurements of TL and HTL-pulsed dendritic cells. Subsequent evaluation and selection by several quality criteria (see Materials and Methods) led to identification of 194 peptides derived from 64 different proteins (Supplementary Table 1, only online). Proteomic profiling of human monocyte-derived dendritic cells revealed that a number of these peptides are derived from ubiquitously expressed proteins (e.g., from house-keeping, cytoskeletal, or matrix proteins).34,35,36,37,38,39,40,41,42,43 These include ribosomal proteins, actin and actin-related proteins, aldolase A, annexin A2, β2-micoglobulin, calmodulin, cathepsin proteins, superoxide dismutase, systatins, elonase 1, galectin 1, glycolysis-related enzymes, histones, HSP70, IL-1RA, kynureninase, macrophage migrating inhibitory factor, peroxiredoxin 6, phosphoglycerated kinase 1, S100 calcium binding proteins and vimentin. Others are derived from proteins that have been shown to be overexpressed in certain cancers, like thymosin beta 4,44 L-plastin,45 makorin-1,46 transmembrane glycoprotein nonmetastatic melanoma protein B (gpnmb),47 cofilin48 or S100A4.49 As a representative example of shared peptides between TL-DC and HTL-DC, the candidate ion of m/z 828.32 (M+2H+)2+ of TL-DC was found to have peptide sequence DKDGNGYISAAELRHVM belonging to human S100 calcium binding protein A4 (residues 94-110, NCBI accession number gi|75765831). The identical peptide was also identified from m/z 625.94 ion ([M+3H]3+) from HTL-DC-eluted samples (Fig. 5).
In the list, there are a number of peptides that are known to be associated with pancreatic cancer including galectin 1,50 cofilin,48 oncogene DJ-1,51 coactosin-like 1,52 cathepsin,53 and S100A454 (Table 1). Exactly the same peptides could also be detected in an independent experiment in which the same dendritic cells were pulsed with different batches of TL and HTL from Panc-1 cells (data not shown), suggesting that epitopes are HLA-restricted and very abundant on MHC class I or II molecules of dendritic cells after uptake of TL or HTL derived from Panc-1 cells. While most of the peptides listed were present in both eluents from HTL-DC and TL-DC that are derived from either ubiquitously expressed proteins (i.e., housekeeping gene products) or from endogenously processed proteins from dendritic cells, MARKS, makorin-1 (zfn-xp protein), CECR-1, thyroid adenoma-associated protein isoform b and transmembrane glycoprotein nonmetastatic melanoma protein B (gpnmb) were detected only in eluents from HTL-DC. On the other hand, prothymosin alpha and cofilin 1 were detected only in TL-DC. Furthermore, comparison of peptides shared by HTL-DC and TL-DC revealed that there are delicate differences in the list of peptides from same proteins. For example, shorter peptides from AHNAK nucleoprotein isoform 1 (desmoyokin) and gelsolin were more abundant in HTL-DC than TL-DC, suggesting that these peptides are derived from class I HLA molecules via cross-presentation. While association with other types of tumor has been reported, AHNAK, SH3 domain binding glutamic acid-rich protein like 3, calpastatin, hnRNP-E1, thyroid adenoma-associated protein isoform b and gpnmb were new additions to pancreatic cancer-associated proteins. Among these, makorin-1, CECR1 and thyroid adenoma-associated protein isoform b protein were previously unrecognized for pancreatic and/or other types of tumors.
One of the challenges in DC-mediated cancer immunotherapy is how to load tumor-associated antigens (TAAs) to DCs in order to generate effector T cells that are powerful enough to overcome systemic and local immunosuppression imposed by tumor. Strategies for antigen-loading to DC include synthetic antigenic peptides,55 freeze/thaw lysate,13 apoptotic tumor cells,56 DC-tumor cell fusion,57 and DC priming with tumor RNA.58 The anti-tumor activity of tumor cell lysate-pulsed DCs was first reported in 1998 and was utilized to treat malignant diseases including melanoma, prostate cancer, renal cancer, lung cancer, lymphoma, brain tumor, liver cancer, and colorectal cancer.59 Theoretically, whole tumor priming strategies providing full repertoire of tumor-specific and tumor-associated antigens are advantageous, as they lower the chance of tumor evasion of the immune response by antigen deletion or mutation and circumvent the need for identification of TAAs from individual cancers. In an effort to further enhance the anti-tumor effect of whole tumor cell lysate-pulsed DCs, we reported previously that tumor lysate prepared from heat-treated tumor cells containing abundant chaperoned TAAs generated a more potent immune response by eliciting higher IFN-γ secretion, and stronger CTL response with greater tumor growth retardation against non-immunogenic murine pancreatic ductal adenocarcinoma as compared with tumor lysate.24 While this approach shares key aspects of tumor lysate in inducing polyclonal cellular immune responses, heat-treated tumor lysate provides additional advantages in stimulating DCs during antigen delivery and possibly in expanded spectrum of presented antigens, resulting in a broader repertoire of elicited T-cell responses.
What mechanisms could account for the higher efficiency of HTL in antigen delivery and DC activation over TL? We favor the concept that composition of HTL greatly differs from TL in the variety and abundance of molecules they contain, thereby making available for antigen presenting cell (APC). It was shown that heat stress induces the expression of HSPs that can carry peptides and proteins derived from tumor-associated proteins60 and are efficiently taken up by APCs with the assistance of the cell surface HSP receptors,61,62,63 helping the antigenic peptide presentation on MHC-I as well as promoting the function of antigen-presenting cells, especially dendritic cells, to generate antigen-specific CD8+ T cells.64,65,66 Thus, HSP-chaperoned TAAs in HTL may represent specific fingerprints of tumor cells which are different from conventional TL. Furthermore, heat-induced HSPs are known to serve as endogenous danger signals to drive DC maturation.67,68 Our findings that the immunostimulatory capacity of heat-treated tumor lysate-loaded DCs (HTL-DC) is more potent than from tumor lysate-loaded DC (TL-DC) clearly supports the postulation.24 Compared to TL-pulsed DCs, immunization with HTL-pulsed DCs induced a large amount of specific CTLs with higher tumor specific cytotoxic activity in naïve mice in vivo. Accordingly, various strategies utilizing various formats of HSPs are currently being tested as vaccines in clinical trials against cancer.65,69 Herein, we addressed the question of whether these 2 types of lysate preparation differ in their capacities to promote maturation of DC and in their antigenic peptide repertoire available for DCs to present to T cells.
HTL, but not TL, induced partial DC maturation in the absence of maturation factors and this effect was further enhanced by the maturation factor (cytokine cocktail), as shown by DC phenotype, allostimulatory capacity and IFN-γ secretion from allogeneic T cells. Thus, increased HSPs in tumor cells may serve as chaperons for antigenic peptides and natural adjuvants for DC maturation during the process of DC antigen processing, presentation and enhancing naïve T cell stimulatory capacity of DCs. The ineffectiveness of TL in stimulating DC suggests that DCs are not responsive to constitutive HSP70 family proteins (HSC70). As HSP is known to facilitate the transfer of antigenic proteins or peptides from tumor lysate to DC, it is likely that peptides chaperoned by HSPs in TL may be taken up by DC with lower efficiency than that of HSPs in HTL. As reported recently, upregulation of HSP expression by cell stress elicited more potent anti-tumor immunity.70,71
In an effort to delineate differences in antigenic peptide profiles from HLA class I and II molecules on DCs pulsed with TL or HTL, we analyzed HLA-associated antigenic peptides derived from dendritic cells through a highly sensitive and well established tandem mass spectrometry (MS-MS) method. Even with this approach, however, only a fraction of the peptides in a complex sample can be successfully sequenced due to the limited dynamic range of MS instrumentation. To identify low abundant tumor cell-derived peptides among the total mixture of MHC class I and II peptides presented by DCs, including self-peptides and tumor-derived housekeeping proteins, peptides identified were compared to previously reported proteomic database of human monocyte-derived dendritic cells. From a single Panc-1 tumor line, more than 194 peptide ligands present in these 2 sets of DCs were identified. The peptides identified varied in size, ranging 8-29 amino acids. In general, MHC class I presents short peptide sequence (8-12 amino acids) and MHC class II present much longer peptides of 14-25 amino acids. However, there are longer peptides that can bind and be eluted off the MHC class I molecules by a protrusion mechanism.72,73 For example, the repertoire of peptides bound to a subset of HLA-B27 in vivo revealed the molecular mass range of 900 to 4000 Da (8 to 33 amino acid residues), unlike the major HLA-B27 with 900-1400 Da (8-12 amino acids). In agreement with the previous report of Weinschenk, et al.,74 the majority of peptides presented in MHC class I and II on the surface of DCs were from common housekeeping proteins (proteins associated with metabolic processes, cell growth and protein synthesis). Apparently, MHC-associated Panc-1-derived ligands contained numerous self-peptides reflecting a comprehensive summary of proteome of normal cells.75
Other peptides were derived from proteins that are overexpressed in pancreatic cancer. Examples in this category were L-plastin,45 profilin 1,76 cofilin 1,48,76 DJ-1,77 AHNAK,78 calpastatin,79 and oncogene prostate tumor inducing gene-1.80 We also identified proteins that have not previously been correlated with pancreatic cancer, including gpnmb, makorin-1 (znf-xp protein) and CECR1 protein (cat eye syndrome critical region protein 1 precursor). As DCs function as vehicles for antigen presentation to the immune system, characterization of MHC peptide antigens from tumor lysate-pulsed DCs would greatly facilitate the identification process of immunogenic peptides for tumor immunotherapy. These peptides in turn could serve as disease markers and indicators of tumor progression.
A most intriguing observation of this study was that the identified peptides eluted from DC upon co-culture with HTL and TL were not identical. First of all, there were differences in profile identified proteins from eluted peptides (i.e., repertoire). While some proteins (makorin-1, CECR1, thyroid adenoma-associated protein isoform b and gpnmb) were detected only in HTL-DC, other proteins (prothymosin alpha and cofilin 1) appeared exclusively in TL-DC. Furthermore, even in proteins shared by HTL-DC and TL-DC, peptides eluted from HTL-DC contained smaller peptides (8-12 amino acids) that were not present in eluents from TL-DC. This finding strongly suggests that DCs cross-presented HTL via receptor-mediated endocytosis (such as by HSPs) more efficiently than conventional TL.
In the present study, we performed the first successful comparative analysis of HLA-associated peptides, which are derived from TL and HTL-pulsed dendritic cells, by nLC-ESI-MS-MS, resulting in a sensitive and rapid sequencing of naturally processed HLA-associated peptides. To the best of our knowledge, a comparative study on HLA-presented tumor antigens, TL-DC and HTL-DC, by using LC-MALDI-MS and LC-ESI-MS has not previously been published. We believe that the approach introduced here provides a powerful complementation to the current methods for expanding the number of human tumor antigens. Vaccine strategies aim to inducing both CD4 and CD8 T cell responses with appropriate combinations of identified tumor antigens may contribute to increase the success rate of cancer immunotherapy.
Figures and Tables
Fig. 1
Western blot analysis for HSP family protein expression by TL and HTL. Human gastric cancer cell line (N87) and human pancreatic ductal adenocarcinoma cell line (Panc-1) were exposed to 42℃ for 1 hr, followed by recovery for 24 hr at 37℃. Tumor cell lysates from untreated tumor lysate (TL) or tumor lysate preparation after heat treatment (HTL) were prepared as described in the Materials and Methods section and immunoblotted with indicated antibodies. HTL, heat-treated tumor lysate; TL, tumor lysate; HSP, heat shock protein.
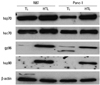
Fig. 2
Phenotypic maturation of dendritic cells induced by HTL, HSP-enriched tumor lysate prepared from heat-treated tumor cells. Human dendritic cells (5×105/mL) were incubated with 4 mg/mL of TL or HTL for 4 hr. After washing, cells were cultured in the presence of GM-CSF and IL-4 (iDC) or in the presence of GM-CSF, IL-4 with cytokine cocktail, composed of TNF-α, IL-1β, IL-6, and PGE2 (mDC), for 48 hr. DCs were collected and stained with monoclonal antibodies specific to HLA-DR, CD40, CD86, CD80, CD1a, and CD83. Gray histograms indicate untreated DCs, fine-line histograms, TL-pulsed DCs; and thick line histograms, HTL-pulsed DCs. Histograms show the expression of CD1a, CD40, CD80, CD86, HLA-DR, and CD83, which represent one representative experiment of four performed. Numbers denote MFI values. HTL, heat-treated tumor lysate; TL, tumor lysate; HSP, heat shock protein; DC, dendritic cell; iDC, immature DC; MFI, median fluorescence intensity; mDC, mature DC.
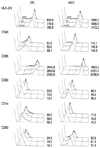
Fig. 3
HTL-pulsed DCs enhanced T cell proliferation, IL-12 production and IFN-γ production. (A) DC exhibits enhanced allostimulatory capacity after pulsing with HTL compared to that of TL-pulsed DC. Gamma-irradiated DC were added to CD3+ T cells at the indicated DC/T cell ratio and incubated for an 5 days before the addition of [3H] thymidine to assess T cell proliferation. Data represent one of three independent experiments with similar results. The error bars are the SEM of triplicate determinations. (B) The production of IL-12p70 was slightly increased by HTL-pulsing. Mature DCs were generated by stimulating immature DCs (iDC) with cytokine cocktail for 48 hr. The error bars are the SD of triplicate determinations. (C) Measurement of IFN-γ in supernatants of allogeneic T cells stimulated by unpulsed, TL-pulsed or HTL-pulsed mature DCs. Allogeneic T cells were stimulated as described in Materials and Methods. The error bars are the SD of triplicate determinations. *p<0.05, **p<0.01. HTL, heat-treated tumor lysate; TL, tumor lysate; DC, dendritic cell.
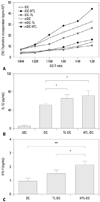
Fig. 4
Base peak chromatograms of naturally processed MHC class I- and II-associated peptides derived from TL-DC (A) and HTL-DC (B). Peptides were eluted from DCs by treatment with citrate-phosphate buffer (pH 3.3) and peptides were separated using a homemade pulled tip capillary column by binary gradient elution at 200 nL/min (see details in Materials and Method section). TL, tumor lysate; DC, dendritic cell; HTL, heat-treated tumor lysate; MHC, major histocompatibility complex.
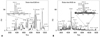
Fig. 5
Identification of tumor-associated peptides eluted from TL-DC or HTL-DC. The MS-MS spectra of m/z 625.94 ([M+3H]3+) from HTL-DC (A) and of m/z 828.32 ([M+2H]2+) from TL (B), which were found to originate from the same protein, human S100 calcium binding protein A4 (residues 94-110). HTL, heat-treated tumor lysate; TL, tumor lysate; DC, dendritic cell.
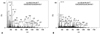
Table 1
Identified Human Pancreatic Cancer Cell-Associated Proteins from Acid-Eluted MHC-Associated Peptides from TL-DC and HTL-DC by Nanoflow LC-ESI-MS-MS
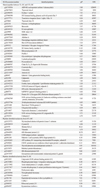
HDL, reated tumor lysate; DC, dendritic cell; TL, tumor lysate; MHC, major histocompatibility complex; LC-ESI-MS-MS, liquid chromatography-electrospray ionization-tandem mass spectrometry; MW, molecular weight; pI, isoelectric point.
*Values for the pI and MW for each protein are based on the database.
ACKNOWLEDGEMENTS
This study was supported by a grant of the Korean Health Technology R&D Project, Ministry of Health & Welfare, Republic of Korea (HI13C1270).
References
1. Berger TG, Feuerstein B, Strasser E, Hirsch U, Schreiner D, Schuler G, et al. Large-scale generation of mature monocyte-derived dendritic cells for clinical application in cell factories. J Immunol Methods. 2002; 268:131–140.


2. Kim S, Kim HO, Baek EJ, Choi Y, Kim HS, Lee MG. Monocyte enrichment from leukapheresis products by using the Elutra cell separator. Transfusion. 2007; 47:2290–2296.


3. Schreurs MW, Eggert AA, de Boer AJ, Vissers JL, van Hall T, Offringa R, et al. Dendritic cells break tolerance and induce protective immunity against a melanocyte differentiation antigen in an autologous melanoma model. Cancer Res. 2000; 60:6995–7001.
4. Hofmann S, Glückmann M, Kausche S, Schmidt A, Corvey C, Lichtenfels R, et al. Rapid and sensitive identification of major histocompatibility complex class I-associated tumor peptides by Nano-LC MALDI MS/MS. Mol Cell Proteomics. 2005; 4:1888–1897.


5. Ridge JP, Di Rosa F, Matzinger P. A conditioned dendritic cell can be a temporal bridge between a CD4+ T-helper and a T-killer cell. Nature. 1998; 393:474–478.


6. Hu HM, Winter H, Urba WJ, Fox BA. Divergent roles for CD4+ T cells in the priming and effector/memory phases of adoptive immunotherapy. J Immunol. 2000; 165:4246–4253.


7. Wang RF. The role of MHC class II-restricted tumor antigens and CD4+ T cells in antitumor immunity. Trends Immunol. 2001; 22:269–276.


8. Schuurhuis DH, Laban S, Toes RE, Ricciardi-Castagnoli P, Kleijmeer MJ, van der Voort EI, et al. Immature dendritic cells acquire CD8(+) cytotoxic T lymphocyte priming capacity upon activation by T helper cell-independent or -dependent stimuli. J Exp Med. 2000; 192:145–150.


9. Melief CJ, Van Der Burg SH, Toes RE, Ossendorp F, Offringa R. Effective therapeutic anticancer vaccines based on precision guiding of cytolytic T lymphocytes. Immunol Rev. 2002; 188:177–182.


10. Dolan BP, Gibbs KD Jr, Ostrand-Rosenberg S. Tumor-specific CD4+ T cells are activated by "cross-dressed" dendritic cells presenting peptide-MHC class II complexes acquired from cell-based cancer vaccines. J Immunol. 2006; 176:1447–1455.


11. Parmiani G, Castelli C, Dalerba P, Mortarini R, Rivoltini L, Marincola FM, et al. Cancer immunotherapy with peptide-based vaccines: what have we achieved? Where are we going? J Natl Cancer Inst. 2002; 94:805–818.


12. Admon A, Barnea E, Ziv T. Tumor antigens and proteomics from the point of view of the major histocompatibility complex peptides. Mol Cell Proteomics. 2003; 2:388–398.


13. Fields RC, Shimizu K, Mulé JJ. Murine dendritic cells pulsed with whole tumor lysates mediate potent antitumor immune responses in vitro and in vivo. Proc Natl Acad Sci U S A. 1998; 95:9482–9487.


14. Nestle FO, Alijagic S, Gilliet M, Sun Y, Grabbe S, Dummer R, et al. Vaccination of melanoma patients with peptide- or tumor lysate-pulsed dendritic cells. Nat Med. 1998; 4:328–332.


15. Schnurr M, Galambos P, Scholz C, Then F, Dauer M, Endres S, et al. Tumor cell lysate-pulsed human dendritic cells induce a T-cell response against pancreatic carcinoma cells: an in vitro model for the assessment of tumor vaccines. Cancer Res. 2001; 61:6445–6450.
16. Yu JS, Liu G, Ying H, Yong WH, Black KL, Wheeler CJ. Vaccination with tumor lysate-pulsed dendritic cells elicits antigen-specific, cytotoxic T-cells in patients with malignant glioma. Cancer Res. 2004; 64:4973–4979.


17. Geiger JD, Hutchinson RJ, Hohenkirk LF, McKenna EA, Yanik GA, Levine JE, et al. Vaccination of pediatric solid tumor patients with tumor lysate-pulsed dendritic cells can expand specific T cells and mediate tumor regression. Cancer Res. 2001; 61:8513–8519.
18. Höltl L, Zelle-Rieser C, Gander H, Papesh C, Ramoner R, Bartsch G, et al. Immunotherapy of metastatic renal cell carcinoma with tumor lysate-pulsed autologous dendritic cells. Clin Cancer Res. 2002; 8:3369–3376.
19. Hatfield P, Merrick AE, West E, O'Donnell D, Selby P, Vile R, et al. Optimization of dendritic cell loading with tumor cell lysates for cancer immunotherapy. J Immunother. 2008; 31:620–632.


20. Sauter B, Albert ML, Francisco L, Larsson M, Somersan S, Bhardwaj N. Consequences of cell death: exposure to necrotic tumor cells, but not primary tissue cells or apoptotic cells, induces the maturation of immunostimulatory dendritic cells. J Exp Med. 2000; 191:423–434.
21. Kotera Y, Shimizu K, Mulé JJ. Comparative analysis of necrotic and apoptotic tumor cells as a source of antigen(s) in dendritic cell-based immunization. Cancer Res. 2001; 61:8105–8109.
22. Galea-Lauri J, Wells JW, Darling D, Harrison P, Farzaneh F. Strategies for antigen choice and priming of dendritic cells influence the polarization and efficacy of antitumor T-cell responses in dendritic cell-based cancer vaccination. Cancer Immunol Immunother. 2004; 53:963–977.


23. Steinman RM, Turley S, Mellman I, Inaba K. The induction of tolerance by dendritic cells that have captured apoptotic cells. J Exp Med. 2000; 191:411–416.


24. Kim HS, Choo YS, Koo T, Bang S, Oh TY, Wen J, et al. Enhancement of antitumor immunity of dendritic cells pulsed with heat-treated tumor lysate in murine pancreatic cancer. Immunol Lett. 2006; 103:142–148.


25. Qiu J, Li GW, Sui YF, Song HP, Si SY, Ge W. Heat-shocked tumor cell lysate-pulsed dendritic cells induce effective anti-tumor immune response in vivo. World J Gastroenterol. 2006; 12:473–478.


26. Bachleitner-Hofmann T, Stift A, Friedl J, Pfragner R, Radelbauer K, Dubsky P, et al. Stimulation of autologous antitumor T-cell responses against medullary thyroid carcinoma using tumor lysate-pulsed dendritic cells. J Clin Endocrinol Metab. 2002; 87:1098–1104.


27. Arnold-Schild D, Hanau D, Spehner D, Schmid C, Rammensee HG, de la Salle H, et al. Cutting edge: receptor-mediated endocytosis of heat shock proteins by professional antigen-presenting cells. J Immunol. 1999; 162:3757–3760.
28. Babatz J, Röllig C, Oelschlägel U, Zhao S, Ehninger G, Schmitz M, et al. Large-scale immunomagnetic selection of CD14+ monocytes to generate dendritic cells for cancer immunotherapy: a phase I study. J Hematother Stem Cell Res. 2003; 12:515–523.


29. Santin AD, Bellone S, Ravaggi A, Pecorelli S, Cannon MJ, Parham GP. Induction of ovarian tumor-specific CD8+ cytotoxic T lymphocytes by acid-eluted peptide-pulsed autologous dendritic cells. Obstet Gynecol. 2000; 96:422–430.


30. Kang D, Moon MH. Development of non-gel-based two-dimensional separation of intact proteins by an on-line hyphenation of capillary isoelectric focusing and hollow fiber flow field-flow fractionation. Anal Chem. 2006; 78:5789–5798.


31. Kang D, Ji ES, Moon MH, Yoo JS. Lectin-based enrichment method for glycoproteomics using hollow fiber flow field-flow fractionation: application to Streptococcus pyogenes. J Proteome Res. 2010; 9:2855–2862.


32. Srivastava PK. Immunotherapy for human cancer using heat shock protein-peptide complexes. Curr Oncol Rep. 2005; 7:104–108.


33. Salio M, Cerundolo V, Lanzavecchia A. Dendritic cell maturation is induced by mycoplasma infection but not by necrotic cells. Eur J Immunol. 2000; 30:705–708.


34. Reynolds JL, Mahajan SD, Aalinkeel R, Nair B, Sykes DE, Schwartz SA. Proteomic analyses of the effects of drugs of abuse on monocyte-derived mature dendritic cells. Immunol Invest. 2009; 38:526–550.


35. Reynolds JL, Mahajan SD, Sykes DE, Schwartz SA, Nair MP. Proteomic analyses of methamphetamine (METH)-induced differential protein expression by immature dendritic cells (IDC). Biochim Biophys Acta. 2007; 1774:433–442.


36. Horlock C, Shakib F, Mahdavi J, Jones NS, Sewell HF, Ghaemmaghami AM. Analysis of proteomic profiles and functional properties of human peripheral blood myeloid dendritic cells, monocyte-derived dendritic cells and the dendritic cell-like KG-1 cells reveals distinct characteristics. Genome Biol. 2007; 8:R30.


37. Watarai H, Hinohara A, Nagafune J, Nakayama T, Taniguchi M, Yamaguchi Y. Plasma membrane-focused proteomics: dramatic changes in surface expression during the maturation of human dendritic cells. Proteomics. 2005; 5:4001–4011.


38. McIlroy D, Tanguy-Royer S, Le Meur N, Guisle I, Royer PJ, Léger J, et al. Profiling dendritic cell maturation with dedicated microarrays. J Leukoc Biol. 2005; 78:794–803.


39. Pereira SR, Faça VM, Gomes GG, Chammas R, Fontes AM, Covas DT, et al. Changes in the proteomic profile during differentiation and maturation of human monocyte-derived dendritic cells stimulated with granulocyte macrophage colony stimulating factor/interleukin-4 and lipopolysaccharide. Proteomics. 2005; 5:1186–1198.


40. Rivollier A, Perrin-Cocon L, Luche S, Diemer H, Strub JM, Hanau D, et al. High expression of antioxidant proteins in dendritic cells: possible implications in atherosclerosis. Mol Cell Proteomics. 2006; 5:726–736.
41. Ferret-Bernard S, Curwen RS, Mountford AP. Proteomic profiling reveals that Th2-inducing dendritic cells stimulated with helminth antigens have a 'limited maturation' phenotype. Proteomics. 2008; 8:980–993.


42. Ferreira GB, van Etten E, Lage K, Hansen DA, Moreau Y, Workman CT, et al. Proteome analysis demonstrates profound alterations in human dendritic cell nature by TX527, an analogue of vitamin D. Proteomics. 2009; 9:3752–3764.


43. Gundacker NC, Haudek VJ, Wimmer H, Slany A, Griss J, Bochkov V, et al. Cytoplasmic proteome and secretome profiles of differently stimulated human dendritic cells. J Proteome Res. 2009; 8:2799–2811.


44. Shiwa M, Nishimura Y, Wakatabe R, Fukawa A, Arikuni H, Ota H, et al. Rapid discovery and identification of a tissue-specific tumor biomarker from 39 human cancer cell lines using the SELDI ProteinChip platform. Biochem Biophys Res Commun. 2003; 309:18–25.


45. Park T, Chen ZP, Leavitt J. Activation of the leukocyte plastin gene occurs in most human cancer cells. Cancer Res. 1994; 54:1775–1781.
46. Shimada H, Shiratori T, Yasuraoka M, Kagaya A, Kuboshima M, Nomura F, et al. Identification of Makorin 1 as a novel SEREX antigen of esophageal squamous cell carcinoma. BMC Cancer. 2009; 9:232.


47. Li YN, Zhang L, Li XL, Cui DJ, Zheng HD, Yang SY, et al. Glycoprotein nonmetastatic B as a prognostic indicator in small cell lung cancer. APMIS. 2014; 122:140–146.


48. Kabbage M, Chahed K, Hamrita B, Guillier CL, Trimeche M, Remadi S, et al. Protein alterations in infiltrating ductal carcinomas of the breast as detected by nonequilibrium pH gradient electrophoresis and mass spectrometry. J Biomed Biotechnol. 2008; 2008:564127.


49. Gongoll S, Peters G, Mengel M, Piso P, Klempnauer J, Kreipe H, et al. Prognostic significance of calcium-binding protein S100A4 in colorectal cancer. Gastroenterology. 2002; 123:1478–1484.


50. Shen J, Person MD, Zhu J, Abbruzzese JL, Li D. Protein expression profiles in pancreatic adenocarcinoma compared with normal pancreatic tissue and tissue affected by pancreatitis as detected by two-dimensional gel electrophoresis and mass spectrometry. Cancer Res. 2004; 64:9018–9026.


51. Melle C, Ernst G, Escher N, Hartmann D, Schimmel B, Bleul A, et al. Protein profiling of microdissected pancreas carcinoma and identification of HSP27 as a potential serum marker. Clin Chem. 2007; 53:629–635.


52. Nakatsura T, Senju S, Ito M, Nishimura Y, Itoh K. Cellular and humoral immune responses to a human pancreatic cancer antigen, coactosin-like protein, originally defined by the SEREX method. Eur J Immunol. 2002; 32:826–836.


53. Wang B, Sun J, Kitamoto S, Yang M, Grubb A, Chapman HA, et al. Cathepsin S controls angiogenesis and tumor growth via matrix-derived angiogenic factors. J Biol Chem. 2006; 281:6020–6029.


54. Rosty C, Ueki T, Argani P, Jansen M, Yeo CJ, Cameron JL, et al. Overexpression of S100A4 in pancreatic ductal adenocarcinomas is associated with poor differentiation and DNA hypomethylation. Am J Pathol. 2002; 160:45–50.


55. Celluzzi CM, Mayordomo JI, Storkus WJ, Lotze MT, Falo LD Jr. Peptide-pulsed dendritic cells induce antigen-specific CTL-mediated protective tumor immunity. J Exp Med. 1996; 183:283–287.


56. Hoffmann TK, Meidenbauer N, Dworacki G, Kanaya H, Whiteside TL. Generation of tumor-specific T-lymphocytes by cross-priming with human dendritic cells ingesting apoptotic tumor cells. Cancer Res. 2000; 60:3542–3549.
57. Gong J, Avigan D, Chen D, Wu Z, Koido S, Kashiwaba M, et al. Activation of antitumor cytotoxic T lymphocytes by fusions of human dendritic cells and breast carcinoma cells. Proc Natl Acad Sci U S A. 2000; 97:2715–2718.


58. Ashley DM, Faiola B, Nair S, Hale LP, Bigner DD, Gilboa E. Bone marrow-generated dendritic cells pulsed with tumor extracts or tumor RNA induce antitumor immunity against central nervous system tumors. J Exp Med. 1997; 186:1177–1182.


59. Cranmer LD, Trevor KT, Hersh EM. Clinical applications of dendritic cell vaccination in the treatment of cancer. Cancer Immunol Immunother. 2004; 53:275–306.


60. Kammerer R, Stober D, Riedl P, Oehninger C, Schirmbeck R, Reimann J. Noncovalent association with stress protein facilitates cross-priming of CD8+ T cells to tumor cell antigens by dendritic cells. J Immunol. 2002; 168:108–117.


61. Delneste Y. Scavenger receptors and heat-shock protein-mediated antigen cross-presentation. Biochem Soc Trans. 2004; 32(Pt 4):633–635.


62. Becker T, Hartl FU, Wieland F. CD40, an extracellular receptor for binding and uptake of Hsp70-peptide complexes. J Cell Biol. 2002; 158:1277–1285.


63. Basu S, Binder RJ, Ramalingam T, Srivastava PK. CD91 is a common receptor for heat shock proteins gp96, hsp90, hsp70, and calreticulin. Immunity. 2001; 14:303–313.


64. Doody AD, Kovalchin JT, Mihalyo MA, Hagymasi AT, Drake CG, Adler AJ. Glycoprotein 96 can chaperone both MHC class I- and class II-restricted epitopes for in vivo presentation, but selectively primes CD8+ T cell effector function. J Immunol. 2004; 172:6087–6092.


65. Wu Y, Wan T, Zhou X, Wang B, Yang F, Li N, et al. Hsp70-like protein 1 fusion protein enhances induction of carcinoembryonic antigen-specific CD8+ CTL response by dendritic cell vaccine. Cancer Res. 2005; 65:4947–4954.


66. Kurotaki T, Tamura Y, Ueda G, Oura J, Kutomi G, Hirohashi Y, et al. Efficient cross-presentation by heat shock protein 90-peptide complex-loaded dendritic cells via an endosomal pathway. J Immunol. 2007; 179:1803–1813.


67. Basu S, Binder RJ, Suto R, Anderson KM, Srivastava PK. Necrotic but not apoptotic cell death releases heat shock proteins, which deliver a partial maturation signal to dendritic cells and activate the NF-kappa B pathway. Int Immunol. 2000; 12:1539–1546.


68. Somersan S, Larsson M, Fonteneau JF, Basu S, Srivastava P, Bhardwaj N. Primary tumor tissue lysates are enriched in heat shock proteins and induce the maturation of human dendritic cells. J Immunol. 2001; 167:4844–4852.


69. Takakura Y, Takemoto S, Nishikawa M. Hsp-based tumor vaccines: state-of-the-art and future directions. Curr Opin Mol Ther. 2007; 9:385–391.
70. Feng H, Zeng Y, Whitesell L, Katsanis E. Stressed apoptotic tumor cells express heat shock proteins and elicit tumor-specific immunity. Blood. 2001; 97:3505–3512.


71. Hashemi SM, Hassan ZM, Soudi S, Ghazanfari T, Kheirandish M, Shahabi S. Evaluation of anti-tumor effects of tumor cell lysate enriched by HSP-70 against fibrosarcoma tumor in BALB/c mice. Int Immunopharmacol. 2007; 7:920–927.


72. Urban RG, Chicz RM, Lane WS, Strominger JL, Rehm A, Kenter MJ, et al. A subset of HLA-B27 molecules contains peptides much longer than nonamers. Proc Natl Acad Sci U S A. 1994; 91:1534–1538.


73. Stryhn A, Pedersen LO, Holm A, Buus S. Longer peptide can be accommodated in the MHC class I binding site by a protrusion mechanism. Eur J Immunol. 2000; 30:3089–3099.


74. Weinschenk T, Gouttefangeas C, Schirle M, Obermayr F, Walter S, Schoor O, et al. Integrated functional genomics approach for the design of patient-individual antitumor vaccines. Cancer Res. 2002; 62:5818–5827.
75. Hickman HD, Luis AD, Buchli R, Few SR, Sathiamurthy M, VanGundy RS, et al. Toward a definition of self: proteomic evaluation of the class I peptide repertoire. J Immunol. 2004; 172:2944–2952.


76. Alldinger I, Dittert D, Peiper M, Fusco A, Chiappetta G, Staub E, et al. Gene expression analysis of pancreatic cell lines reveals genes overexpressed in pancreatic cancer. Pancreatology. 2005; 5:370–379.


77. Grønborg M, Kristiansen TZ, Iwahori A, Chang R, Reddy R, Sato N, et al. Biomarker discovery from pancreatic cancer secretome using a differential proteomic approach. Mol Cell Proteomics. 2006; 5:157–171.


78. Uozumi N, Gao C, Yoshioka T, Nakano M, Moriwaki K, Nakagawa T, et al. Identification of a novel type of CA19-9 carrier in human bile and sera of cancer patients: an implication of the involvement in nonsecretory exocytosis. J Proteome Res. 2010; 9:6345–6353.


79. Storr SJ, Zaitoun AM, Arora A, Durrant LG, Lobo DN, Madhusudan S, et al. Calpain system protein expression in carcinomas of the pancreas, bile duct and ampulla. BMC Cancer. 2012; 12:511.


80. Ohnami S, Matsumoto N, Nakano M, Aoki K, Nagasaki K, Sugimura T, et al. Identification of genes showing differential expression in antisense K-ras-transduced pancreatic cancer cells with suppressed tumorigenicity. Cancer Res. 1999; 59:5565–5571.