Abstract
Telomerase reverse transcriptase (TERT) is the protein component of telomerase and combined with an RNA molecule, telomerase RNA component, forms the telomerase enzyme responsible for telomere elongation. Telomerase is essential for maintaining telomere length from replicative attrition and thus contributes to the preservation of genome integrity. Although diverse mouse models have been developed and studied to prove the physiological roles of telomerase as a telomere-elongating enzyme, recent studies have revealed non-canonical TERT activities beyond telomeres. To gain insights into the physiological impact of extra-telomeric roles, this review revisits the strategies and phenotypes of telomerase mouse models in terms of the extra-telomeric functions of telomerase.
During DNA replication, the linear ends of chromosomes are eroded at each cell division due to the end replication problem.1 Telomeres, the very ends of linear chromosomes, are predominantly composed of tandem repeats of short sequences; in vertebrates, the repeats consist of the TTAGGG hexanucleotide.2 Telomere lengths are also remarkably heterogeneous among individuals and vary according to the origin, age, and proliferative history of cells.3,4 Telomere length variations among individuals of the same age are, therefore, thought to be related to variations in ageing and longevity.5 As a ribonucleoprotein complex that is composed of telomerase reverse transcriptase (TERT) and telomerase RNA component (TERC),6 telomerase is responsible for elongation of the telomeres, and thus maintains genome stability.7,8 The enzymatic activity of telomerase is not detected in normal somatic cells, but is detected in embryonic and highly proliferative adult tissues.9 Furthermore, telomerase is re-activated in most cancers,10 thus suggesting the possibility that telomerase is a potential therapeutic target in cancers.
Recently, a number of reports have shown extra-telomeric functions of TERT. The following observations pose questions regarding the rationality of telomere length-dependent singular role of telomerase:
1) Although tissue stem and progenitor cells have sufficient telomere reserves, they highly express TERT. Notably, laboratory mice have significantly longer telomeres than humans (40-60 kb vs. 5-15 kb);11-14 however, no apparent role for long telomeres has been found in the survival of mice.
2) TERT overexpression promotes tumor development without further telomere elongation.15
3) The reconstitution ability of hematopoietic stem cells (HSCs) is essentially linked with TERT, although there is no evidence that HSC activities are fully dependent on the telomere-elongation function of TERT.16,17
4) Transgenic mice overexpressing murine TERT show significant resistance to ischemic brain injury and N-methyl-D-aspartic acid (NMDA) receptor-mediated excitotoxicity without any detectable change in telomere length.18 Interestingly, ischemic injury induces TERT expression in the wild-type brain.18
5) Suppression of TERT expression decreases cell growth rate and induces apoptosis prior to measurable telomere shortening,19 and the expression of specific TERT mutants lacking telomerase activity prevents apoptotic cell death.20
6) Ectopic expression of TERT in the hair follicle stem cells of mouse epidermis activates stem cell capacities.21 The phenotype appears to be independent of telomerase activity.
7) Cancers without functional telomerase (10-15% of all cases) maintain their telomere lengths by adopting an alternative lengthening of telomeres (ALT) pathway.22
Furthermore, indirect evidence also indicates extra-telomeric functions of telomerase: TERT may have additional functions because the reverse transcriptase (RT) domain of TERT is only 15 kDa, which is less than 10% of the total molecular weight. Thus, it is quite possible that other regions may mediate distinct activities other than telomerase activity.23 In support of this hypothesis, alternatively spliced forms of TERT devoid of the RT domain have been identified in humans.24 Although TERT is known as a nuclear protein, it is exported from the nucleus and delays replicative senescence in endothelial cells dependent on reactive oxygen species (ROS).25
Several lines of evidence obtained using mouse models have strengthened the idea that TERT contributes to preventing ageing and cell death, as well as promoting carcinogenesis, cell signaling, and transcriptional regulation, which are unrelated to its role in telomere lengthening. Here, we review the studies encompassing the extra-telomeric functions of telomerase conducted using transgenic or knockout mouse models.
Apoptosis induced by critically short telomeres has been extensively documented. Dysfunctional telomeres increase apoptosis in highly proliferative tissues including intestine,26,27 male germ cells,26,28 and splenocytes (B cells) from immunized fifth and sixth generation mice after mitogen treatment.29 These dysfunctional telomeres can be generated in early generations, and can increase apoptosis in these tissues as well. Protection of Telomeres 1 (POT1) is a single-stranded telomere binding protein that is essential for proper maintenance of telomere length. When Pot1 is deficient, abnormal apoptosis is induced in proliferative tissues as well as cells derived from Terc-/- mice, including male germ cells, hematopoietic cells, and intestinal cells.30 Critically short telomeres also affect highly proliferative developmental processes. Terc-/- embryos at embryonic day 10.5 (E10.5) with dysfunctional telomeres frequently exhibit neural tube closure defects, suggesting that this is one of the most sensitive developmental processes to telomere loss and chromosomal instability.31 Although cardiac tissues are not highly proliferative, the balance between cell growth and cell death is critical for maintaining normal heart function. Consistently, dysfunctional telomeres lead to abnormal apoptosis in cardiomyocytes, resulting in cardiac dilatation and heart failure in the late generation of Terc-/- mice.32 Additionally, cardiomyocyte survival is promoted in transgenic mice overexpressing wild-type TERT, but not expressing mutant TERT.33 Tert deficiency also induces apoptotic phenotypes; Tert-/- and Terc-/- mice show frequent apoptosis in intestinal crypt cells34-36 and male germ cells37 respectively in their late generations. Furthermore, the deleterious effects can be rescued by turning on telomerase activity in the late generation of homozygous ER-Tert knock-in mice by treating with tamoxifen.37 These results clearly demonstrate that telomerase deficiency elicits telomere erosion, resulting in abnormal apoptotic phenotypes in vivo.
Telomerase-deficient mouse models have provided opportunities for unraveling the mechanisms which induce these apoptotic phenotypes in late generations. Rajaraman, et al.36 showed using Tert-/- mice that apoptosis is dependent on S phase, and thus is primarily triggered by newly uncapped (or critically short) telomeres. It is not triggered by chromosome fusion-bridge breakage because mitotic blockade did not alter the apoptotic pattern.36 It is also dependent on p53 that is activated by genotoxic stresses, including critically short telomeres.36,38,39 In fact, growth arrest and/or apoptosis in late generations of Terc-/- mice are dependent on proper p53 activation.32,40 However, p53-mediated regulation of the phenotypic manifestations in telomerase knockout mice is complicated by its negative effect on TERT gene expression.41 Rahman, et al.20 evaluated the effect of human TERT (hTERT) overexpression on p53-dependent apoptosis. In HCT116 colon carcinoma cells carrying endogenous p53, genotoxic stress-induced apoptosis that is p53-dependent is suppressed by constitutive hTERT expression. Indeed, a telomerase-inactive hTERT mutant equally antagonizes p53-induced apoptosis.20 Similarly, ectopic mouse TERT expression in mouse embryonic stem cells that exhibit high levels of telomerase activity and maintain sufficiently long telomeres confers resistance to p53-dependent apoptosis.42 In addition to telomere-associated functions, these results indicate that TERT exerts antiapoptotic activity beyond telomeres.
In addition to the phenotypes induced by critically short telomeres, emerging evidence has indicated the existence of extra-telomeric functions of telomerase. The first clue for an extra-telomeric role of TERT was obtained by revealing the neuroprotective effect of TERT on neuronal cell death induced by the neurotoxic protein amyloid β-peptide, a protein believed to promote neuronal degeneration in Alzheimer's disease.43 Using transgenic mice ubiquitously overexpressing TERT, we also have provided evidence that TERT prevents NMDA neurotoxicity through the transfer of cytosolic free Ca2+ into the mitochondria, thereby playing a protective role in ameliorating ischemic neuronal cell death.18 Because TERT is induced in postmitotic neurons by ischemic brain injury and its overexpression confers resistance against NMDA neurotoxicity, these protective phenotypes are considered to be independent of telomerase activity. Similarly, first generation (G1) Tert-deficient mouse embryonic fibroblasts (MEFs) displayed increased sensitivity to staurosporine (STS), whereas Tert transgenic MEFs were more resistant to STS-induced apoptosis than wild-type.44 Consistent phenotypes were also observed upon NMDA treatment of Tert-deficient and Tert transgenic mice, respectively.44 Although extensive studies were conducted, it remains unclear whether the protective function is dependent on telomerase activity.44 In fact, Terc deficiency does not alter the sensitivity of Tert transgenic MEFs to STS treatment, and NMDA-induced excitotoxic cell death of primary neurons was suppressed by TERT, but not by Terc deficiency, in vitro and in vivo.44 Furthermore, although telomerase activity is evidently suppressed in transgenic mice overexpressing hTERT, hTERT transgenic MEFs still show resistance to STS-induced apoptosis.44 Based on these lines of evidence, telomerase activity must not be essential for the protective function of TERT. Therefore, independent of its roles in telomere maintenance, diverse telomerase mouse models have demonstrated that TERT-mediated antiapoptotic functions may contribute to tumorigenesis.
Telomerase knockout or transgenic mouse models have been extensively employed to elucidate the in vivo roles of telomerase and dysfunctional telomeres in tumorigenesis.
Telomeres are dedicated to the maintenance of linear chromosomes and thus prevent chromosomal abnormalities. In cultivated cells from late generations of Terc-/- mice, critically short or dysfunctional telomeres induce aneuploidy and chromosomal abnormalities, including end-to-end fusions.45 These phenomena are prevalent in cancer, and spontaneous tumors are more frequently induced in late generation Terc-/- mice,46 indicating that dysfunctional telomeres are genotoxic and possess mutagenic effects in mice. Additionally, it is plausible that p53 deficiency significantly attenuates genotoxic stresses triggered by telomere dysfunction. In fact, p53 deficiency contributes to the neoplastic transformation of cells with critically short telomeres from late generation Terc-/- mice40 and promotes non-reciprocal translocations and epithelial cancers.47 In contrast, these critically short telomeres also suppress tumor formation in cancer-prone Ink4a/Arf-deficient mice that still possess intact DNA damage responses.48 The phenotypes obtained from studies using Terc-/- mice support the tumor suppressive role of intact telomeres in maintaining genomic integrity, and prove the intimate genetic interaction between telomere regulation and p53-governed genomic surveillance.
Tert deficiency also results in overtly similar phenotypes to Terc deficiency in tumorigenesis, but the phenotypic manifestations are not completely identical, thus revealing the extra-telomeric role of TERT in tumorigenesis. The seminal observation was obtained from in vitro experiments employing human cell lines. Immortalized human cells are frequently transformed by introducing an oncogene such as ras; however, oncogenic ras cannot fully transform immortalized human cells that are TERT-deficient ALT cells.49 Interestingly, hTERT overexpression confers fully malignant traits to cells expressing oncogenic ras.49 A hemagglutinin (HA) epitope-tagged hTERT (hTERT-HA) that is defective in maintaining telomeres in vivo also exhibits comparable effects on cellular transformation.49 Similarly, hTERT overexpression in human mammary epithelial cells with epigenetically silenced p16INK4a resulted in increased resistance to growth arrest mediated by transforming growth factor β (TGF-β).50 Because resistance to TGF-β-induced growth inhibition is independent of telomere length,50 TERT possesses telomere-independent roles that cooperate with p16INK4a inactivation to promote tumor development. These results clearly demonstrate an oncogenic role of TERT beyond telomeres.
Extensive studies adopting diverse models have recently revealed the extra-telomeric roles of oncogenic TERT at an organismal level (Table 1). For example, telomere dysfunction in late generation Terc-/- mice enhances the initiation of hepatocellular carcinogenesis, but suppresses progression into fully malignant carcinomas.51 In contrast, enhanced tumor initiation does not occur in late generations of Tert-/- mice,35 indicating a possible oncogenic effect of TERT, other than telomeres, in tumorigenesis. Strong induction of TERT expression in hepatic neoplasms may also support its procarcinogenic effect on hepatic tumorigenesis.35 Consistently, transgenic overexpression of Tert promotes the development of spontaneous cancers in ageing mice.52 When TERT overexpression is targeted to basal keratinocytes using the bovine keratin 5 promoter, these transgenic mice show normal telomere length in their stratified epithelia even with high levels of telomerase activity.15 Interestingly, these mice are more susceptible to experimental skin carcinogenesis employing 7,12-dimethylbenz[a]anthracene and 12-o-tetradecanoylphorbol 13-acetate than wild-type mice.15 In addition, TERT overexpression actively promotes proliferation in epidermal tissues without telomere elongation.15 These results from mouse models suggest extra-telomeric roles of TERT, particularly in promoting tumor progression.
Telomerase mouse models have been also extensively used to validate telomerase as an important target for anticancer therapies. Since telomere dysfunction increases the chemo-sensitivity of Terc-deficient transformed MEFs, the combination of chemotherapy and telomerase inhibition may be an effective anticancer approach.53 Recently, Ding, et al.34 showed that telomerase reactivation by conditional rescue of Tert expression in mice with dysfunctional telomeres resulted in bone metastases of prostate tumors. Although the authors did not discuss the extra-telomeric roles of TERT in their study, this report is reminiscent of ALT cell transformation by hTERT overexpression.49 From this standpoint, anti-telomeric drugs are considered as an effective strategy for curing cancers. However, anti-telomerase therapy certainly provokes ALT and mitochondrial adaptive mechanisms in cancer,54 and with respect to the extra-telomeric functions of telomerase, anti-telomeric drugs may not be the best drug candidates.55
Taken together, telomerase exerts pleiotropic effects in cancer both dependent on and independent from its roles in telomeres. As described in Table 1, there are complex genetic interactions of telomerase with diverse genes. In conjunction with the currently emerging mechanisms of extra-telomeric roles, telomerase mouse models will expedite the invention of anti-telomerase strategies for cancer treatment.
Stem cells support tissue homeostasis and regeneration after certain types of damage. Because stem cells possess self-renewal potential and indefinitely propagate, high levels of telomerase activity should be essential for telomere maintenance. Therefore, extensive studies have been conducted to identify the patho-physiological consequences of telomerase deficiency or overexpression in stem cell function using diverse telomerase mouse models. However, telomere dysfunction is likely to affect stem cell functions in a context-dependent manner. Indeed, late-generation Terc-/- HSCs with short telomeres exhibit reduced proliferation capacity, but still possess long-term repopulating ability.56 Interestingly, when serially transplanted into recipient mice, the telomeres are considerably shortened even in wild-type HSCs, which is accelerated by approximately 2-fold in both Terc-/- and Tert-/- mice.16 Consistently, these telomerase-deficient HSCs exhibit considerably reduced replicative capacity compared to wild-type HSCs.16 However, although the telomere length of HSCs is constantly maintained by TERT overexpression in the transgenic mice, the long-term transplantation capacity of HSCs is not enhanced.57 Furthermore, Tert deficiency exacerbates senescence and the sensitivity of ataxia-telangiectasia mutated deficient murine HSCs against ROS-induced apoptosis, which does not accompany telomere shortening or dysfunction.17 These results suggest that telomerase may regulate the long-term replicative capacity of HSCs independently of telomere length.
Epidermal stem cells are also regulated by telomerase both dependent and independent of telomeres. In late generation Terc-/- mice, epidermal stem cell functions are significantly suppressed by critically short telomeres.21,58 However, epidermal overexpression of TERT under the control of the K5 promoter does not alter telomere length, but promotes stem cell mobilization, hair growth, and stem cell proliferation in vitro.18 Similarly, transgenic mice conditionally overexpressing TERT show robust hair growth via proliferation of quiescent, multipotent stem cells in the hair follicles.13 These phenotypes are also reproduced in a Terc-deficient genetic background without telomere dysfunction,13 thereby indicating the extra-telomeric activity of TERT.
In addition to the critical roles of p53 in mediating phenotypic manifestations against critically short telomeres,59 clues for the molecular mechanisms governing the extra-telomeric roles of telomerase have been obtained by identifying the positive effect of TERT on gene expression. Choi, et al.60 found that TERT triggers a rapid change in gene expression in the skin and hair follicles. This gene expression pattern significantly overlaps those controlling natural hair follicle cycling in wild-type mice. TERT affects the developmental program mediated by Myc and Wnt, which is intimately associated with stem cell function and cancer.60 Furthermore, TERT binds BRG1 (also called SMARCA4), a SWI/SNF-related chromatin remodeling protein, and directly modulates Wnt/β-catenin signaling as a cofactor in the β-catenin transcriptional complex.61 Therefore, independently of telomeres, TERT can act as a transcriptional regulator that is directly involved in stem cell functions, including in mouse epidermal tissues.
For more than a decade, diverse telomerase mouse models have provided us with precious opportunities for evaluating the patho-physiological significance of telomerase in genetically defined environments and at an organismal level. With an emphasis on defective telomeres, these mouse models have considerably contributed to understanding a broad spectrum of phenomena associated with cancer and ageing. Furthermore, growing evidence has indicated that defective telomerase functions are involved in distinct diseases other than human cancers including dyskeratosis congenita, atherosclerosis, and renal diseases.62-64 The list of disease-associated mutations has been expanding. To genetically define the pathological aspects and thus to establish animal models of these mutations, novel mouse models should be still generated and analyzed.
Despite the evident roles in telomeres, currently emerging extra-telomeric functions of telomerase are completely changing the scope of this enzyme. Notably, the direct roles of TERT in transcriptional regulation (e.g. Wnt/β-catenin and nuclear factor-κB or NFκB) provide good rationale for several phenotypes that cannot be explained by telomere dysfunction, and their physiological significance has been also confirmed using telomerase mouse models.62,64,65 As might be expected, these lines of evidence make us consider that diverse observations supporting extra-telomeric roles of telomerase should be scrutinized and validated in vivo by generating novel mouse models. For example, in addition to the effect of short telomeres on mitochondria,39 mitochondrial targeting of telomerase upon certain stressful conditions66 and the recently identified RNA-dependent RNA polymerase activity of TERT,67,68 indicates that telomerase has direct roles in mitochondria. Furthermore, considering the important roles of telomerase in cellular homeostasis, telomerase may be a critical factor for regulating the subcellular organelle homeostasis. Undoubtedly, we believe that these extra-telomeric functions of telomerase should be intimately associated with life span regulation, and that some regions of TERT, other than the RT domain, will be required for mediating protein-protein interactions with known functions in controlling the life span of an organism. In this context, we cannot rule out speculations for divergent mechanisms of telomerase function regulating survival, tumor progression, development/differentiation, and stress responses. These extra-telomeric functions have inevitably complicated the phenotypic manifestations elicited by dysfunctional telomeres and vice versa; thus, to separate these distinct functions of telomerase, more sophisticated genetic strategies should be developed in mice.
Figures and Tables
Table 1
Phenotypes of Telomerase Mouse Models
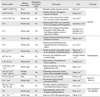
TERT, telomerase reverse transcriptase; HSC, hematopoietic stem cell.
*Mouse α-myosin heavy chain (MHC) promoter.
†Human cytomegalovirus immediate-early enhancer linked to the chicken β-actin promoter (CAG).
‡Bovine keratin 5 promoter (K5).
§Thymus-specific light-chain kinase (Lck) promoter.
∥Actin-rtTA+;tetop-TERT+ (termed as doxycycline-inducible Tert or i-Tert).
¶Lox-Stop-Lox cassette.
**Doxycycline-inducible expression of mutant TERT without telomerase activity under the regulation of K5-rtTA promoter.
ACKNOWLEDGEMENTS
This work was supported by National Research Foundation of Korea (NRF) grants funded by Ministry of Education, Science and Technology (MEST) of the Korean government (2009-0081177, 2010-0020878); a Korea Healthcare Technology R&D Project from the Ministry for Health & Welfare Affairs (A085136). M.A. was also supported by Higher Education Commission (HEC) of Pakistan government.
References
1. Bischoff C, Graakjaer J, Petersen HC, Hjelmborg Jv, Vaupel JW, Bohr V, et al. The heritability of telomere length among the elderly and oldest-old. Twin Res Hum Genet. 2005; 8:433–439.


4. Lansdorp PM. Telomere length and proliferation potential of hematopoietic stem cells. J Cell Sci. 1995; 108(Pt 1):1–6.


5. Heidinger BJ, Blount JD, Boner W, Griffiths K, Metcalfe NB, Monaghan P. Telomere length in early life predicts lifespan. Proc Natl Acad Sci U S A. 2012; 109:1743–1748.


6. Cohen SB, Graham ME, Lovrecz GO, Bache N, Robinson PJ, Reddel RR. Protein composition of catalytically active human telomerase from immortal cells. Science. 2007; 315:1850–1853.


7. Bianchi A, Shore D. Early replication of short telomeres in budding yeast. Cell. 2007; 128:1051–1062.


8. Laterreur N, Eschbach SH, Lafontaine DA, Wellinger RJ. A new telomerase RNA element that is critical for telomere elongation. Nucleic Acids Res. 2013; 41:7713–7724.


9. Wright WE, Piatyszek MA, Rainey WE, Byrd W, Shay JW. Telomerase activity in human germline and embryonic tissues and cells. Dev Genet. 1996; 18:173–179.


10. Kim NW, Piatyszek MA, Prowse KR, Harley CB, West MD, Ho PL, et al. Specific association of human telomerase activity with immortal cells and cancer. Science. 1994; 266:2011–2015.


11. Allshire RC, Dempster M, Hastie ND. Human telomeres contain at least three types of G-rich repeat distributed non-randomly. Nucleic Acids Res. 1989; 17:4611–4627.


12. Prowse KR, Greider CW. Developmental and tissue-specific regulation of mouse telomerase and telomere length. Proc Natl Acad Sci U S A. 1995; 92:4818–4822.


13. Sarin KY, Cheung P, Gilison D, Lee E, Tennen RI, Wang E, et al. Conditional telomerase induction causes proliferation of hair follicle stem cells. Nature. 2005; 436:1048–1052.


14. Starling JA, Maule J, Hastie ND, Allshire RC. Extensive telomere repeat arrays in mouse are hypervariable. Nucleic Acids Res. 1990; 18:6881–6888.


15. González-Suárez E, Samper E, Ramírez A, Flores JM, Martín-Caballero J, Jorcano JL, et al. Increased epidermal tumors and increased skin wound healing in transgenic mice overexpressing the catalytic subunit of telomerase, mTERT, in basal keratinocytes. EMBO J. 2001; 20:2619–2630.


16. Allsopp RC, Morin GB, DePinho R, Harley CB, Weissman IL. Telomerase is required to slow telomere shortening and extend replicative lifespan of HSCs during serial transplantation. Blood. 2003; 102:517–520.


17. Nitta E, Yamashita M, Hosokawa K, Xian M, Takubo K, Arai F, et al. Telomerase reverse transcriptase protects ATM-deficient hematopoietic stem cells from ROS-induced apoptosis through a telomere-independent mechanism. Blood. 2011; 117:4169–4180.


18. Kang HJ, Choi YS, Hong SB, Kim KW, Woo RS, Won SJ, et al. Ectopic expression of the catalytic subunit of telomerase protects against brain injury resulting from ischemia and NMDA-induced neurotoxicity. J Neurosci. 2004; 24:1280–1287.


19. Folini M, Brambilla C, Villa R, Gandellini P, Vignati S, Paduano F, et al. Antisense oligonucleotide-mediated inhibition of hTERT, but not hTERC, induces rapid cell growth decline and apoptosis in the absence of telomere shortening in human prostate cancer cells. Eur J Cancer. 2005; 41:624–634.


20. Rahman R, Latonen L, Wiman KG. hTERT antagonizes p53-induced apoptosis independently of telomerase activity. Oncogene. 2005; 24:1320–1327.


21. Flores I, Cayuela ML, Blasco MA. Effects of telomerase and telomere length on epidermal stem cell behavior. Science. 2005; 309:1253–1256.


22. Heaphy CM, Subhawong AP, Hong SM, Goggins MG, Montgomery EA, Gabrielson E, et al. Prevalence of the alternative lengthening of telomeres telomere maintenance mechanism in human cancer subtypes. Am J Pathol. 2011; 179:1608–1615.


23. Sýkorová E, Fajkus J. Structure-function relationships in telomerase genes. Biol Cell. 2009; 101:375–392.


24. Ulaner GA, Hu JF, Vu TH, Giudice LC, Hoffman AR. Telomerase activity in human development is regulated by human telomerase reverse transcriptase (hTERT) transcription and by alternate splicing of hTERT transcripts. Cancer Res. 1998; 58:4168–4172.
25. Haendeler J, Hoffmann J, Diehl JF, Vasa M, Spyridopoulos I, Zeiher AM, et al. Antioxidants inhibit nuclear export of telomerase reverse transcriptase and delay replicative senescence of endothelial cells. Circ Res. 2004; 94:768–775.


26. Khoo CM, Carrasco DR, Bosenberg MW, Paik JH, Depinho RA. Ink4a/Arf tumor suppressor does not modulate the degenerative conditions or tumor spectrum of the telomerase-deficient mouse. Proc Natl Acad Sci U S A. 2007; 104:3931–3936.


27. Siegl-Cachedenier I, Muñoz P, Flores JM, Klatt P, Blasco MA. Deficient mismatch repair improves organismal fitness and survival of mice with dysfunctional telomeres. Genes Dev. 2007; 21:2234–2247.


28. Liu L, Franco S, Spyropoulos B, Moens PB, Blasco MA, Keefe DL. Irregular telomeres impair meiotic synapsis and recombination in mice. Proc Natl Acad Sci U S A. 2004; 101:6496–6501.


29. Herrera E, Martínez-A C, Blasco MA. Impaired germinal center reaction in mice with short telomeres. EMBO J. 2000; 19:472–481.


30. He H, Wang Y, Guo X, Ramchandani S, Ma J, Shen MF, et al. Pot1b deletion and telomerase haploinsufficiency in mice initiate an ATR-dependent DNA damage response and elicit phenotypes resembling dyskeratosis congenita. Mol Cell Biol. 2009; 29:229–240.


31. Herrera E, Samper E, Blasco MA. Telomere shortening in mTR-/- embryos is associated with failure to close the neural tube. EMBO J. 1999; 18:1172–1181.


32. Leri A, Franco S, Zacheo A, Barlucchi L, Chimenti S, Limana F, et al. Ablation of telomerase and telomere loss leads to cardiac dilatation and heart failure associated with p53 upregulation. EMBO J. 2003; 22:131–139.


33. Oh H, Taffet GE, Youker KA, Entman ML, Overbeek PA, Michael LH, et al. Telomerase reverse transcriptase promotes cardiac muscle cell proliferation, hypertrophy, and survival. Proc Natl Acad Sci U S A. 2001; 98:10308–10313.


34. Ding Z, Wu CJ, Jaskelioff M, Ivanova E, Kost-Alimova M, Protopopov A, et al. Telomerase reactivation following telomere dysfunction yields murine prostate tumors with bone metastases. Cell. 2012; 148:896–907.


35. Farazi PA, Glickman J, Horner J, Depinho RA. Cooperative interactions of p53 mutation, telomere dysfunction, and chronic liver damage in hepatocellular carcinoma progression. Cancer Res. 2006; 66:4766–4773.


36. Rajaraman S, Choi J, Cheung P, Beaudry V, Moore H, Artandi SE. Telomere uncapping in progenitor cells with critical telomere shortening is coupled to S-phase progression in vivo. Proc Natl Acad Sci U S A. 2007; 104:17747–17752.


37. Jaskelioff M, Muller FL, Paik JH, Thomas E, Jiang S, Adams AC, et al. Telomerase reactivation reverses tissue degeneration in aged telomerase-deficient mice. Nature. 2011; 469:102–106.


38. Cosme-Blanco W, Shen MF, Lazar AJ, Pathak S, Lozano G, Multani AS, et al. Telomere dysfunction suppresses spontaneous tumorigenesis in vivo by initiating p53-dependent cellular senescence. EMBO Rep. 2007; 8:497–503.


39. Sahin E, Depinho RA. Linking functional decline of telomeres, mitochondria and stem cells during ageing. Nature. 2010; 464:520–528.


40. Chin L, Artandi SE, Shen Q, Tam A, Lee SL, Gottlieb GJ, et al. p53 deficiency rescues the adverse effects of telomere loss and cooperates with telomere dysfunction to accelerate carcinogenesis. Cell. 1999; 97:527–538.


41. Xu D, Wang Q, Gruber A, Björkholm M, Chen Z, Zaid A, et al. Downregulation of telomerase reverse transcriptase mRNA expression by wild type p53 in human tumor cells. Oncogene. 2000; 19:5123–5133.


42. Lee MK, Hande MP, Sabapathy K. Ectopic mTERT expression in mouse embryonic stem cells does not affect differentiation but confers resistance to differentiation- and stress-induced p53-dependent apoptosis. J Cell Sci. 2005; 118(Pt 4):819–829.


43. Zhu H, Fu W, Mattson MP. The catalytic subunit of telomerase protects neurons against amyloid beta-peptide-induced apoptosis. J Neurochem. 2000; 75:117–124.


44. Lee J, Sung YH, Cheong C, Choi YS, Jeon HK, Sun W, et al. TERT promotes cellular and organismal survival independently of telomerase activity. Oncogene. 2008; 27:3754–3760.


45. Blasco MA, Lee HW, Hande MP, Samper E, Lansdorp PM, DePinho RA, et al. Telomere shortening and tumor formation by mouse cells lacking telomerase RNA. Cell. 1997; 91:25–34.


46. Rudolph KL, Chang S, Lee HW, Blasco M, Gottlieb GJ, Greider C, et al. Longevity, stress response, and cancer in aging telomerase-deficient mice. Cell. 1999; 96:701–712.


47. Artandi SE, Chang S, Lee SL, Alson S, Gottlieb GJ, Chin L, et al. Telomere dysfunction promotes non-reciprocal translocations and epithelial cancers in mice. Nature. 2000; 406:641–645.


48. Greenberg RA, Chin L, Femino A, Lee KH, Gottlieb GJ, Singer RH, et al. Short dysfunctional telomeres impair tumorigenesis in the INK4a(delta2/3) cancer-prone mouse. Cell. 1999; 97:515–525.


49. Stewart SA, Hahn WC, O'Connor BF, Banner EN, Lundberg AS, Modha P, et al. Telomerase contributes to tumorigenesis by a telomere length-independent mechanism. Proc Natl Acad Sci U S A. 2002; 99:12606–12611.


50. Stampfer MR, Garbe J, Levine G, Lichtsteiner S, Vasserot AP, Yaswen P. Expression of the telomerase catalytic subunit, hTERT, induces resistance to transforming growth factor beta growth inhibition in p16INK4A(-) human mammary epithelial cells. Proc Natl Acad Sci U S A. 2001; 98:4498–4503.


51. Farazi PA, Glickman J, Jiang S, Yu A, Rudolph KL, DePinho RA. Differential impact of telomere dysfunction on initiation and progression of hepatocellular carcinoma. Cancer Res. 2003; 63:5021–5027.
52. Artandi SE, Alson S, Tietze MK, Sharpless NE, Ye S, Greenberg RA, et al. Constitutive telomerase expression promotes mammary carcinomas in aging mice. Proc Natl Acad Sci U S A. 2002; 99:8191–8196.


53. Lee KH, Rudolph KL, Ju YJ, Greenberg RA, Cannizzaro L, Chin L, et al. Telomere dysfunction alters the chemotherapeutic profile of transformed cells. Proc Natl Acad Sci U S A. 2001; 98:3381–3386.


54. Hu X, Li Y, Li C, Fu Y, Cai F, Chen Q, et al. Combination of fucoxanthin and conjugated linoleic acid attenuates body weight gain and improves lipid metabolism in high-fat diet-induced obese rats. Arch Biochem Biophys. 2012; 519:59–65.


55. Roh JI, Sung YH, Lee HW. Clinical implications of antitelomeric drugs with respect to the nontelomeric functions of telomerase in cancer. Onco Targets Ther. 2013; 6:1161–1166.
56. Samper E, Fernández P, Eguía R, Martín-Rivera L, Bernad A, Blasco MA, et al. Long-term repopulating ability of telomerase-deficient murine hematopoietic stem cells. Blood. 2002; 99:2767–2775.


57. Allsopp RC, Morin GB, Horner JW, DePinho R, Harley CB, Weissman IL. Effect of TERT over-expression on the long-term transplantation capacity of hematopoietic stem cells. Nat Med. 2003; 9:369–371.


58. Siegl-Cachedenier I, Flores I, Klatt P, Blasco MA. Telomerase reverses epidermal hair follicle stem cell defects and loss of long-term survival associated with critically short telomeres. J Cell Biol. 2007; 179:277–290.


59. Flores I, Blasco MA. A p53-dependent response limits epidermal stem cell functionality and organismal size in mice with short telomeres. PLoS One. 2009; 4:e4934.


60. Choi J, Southworth LK, Sarin KY, Venteicher AS, Ma W, Chang W, et al. TERT promotes epithelial proliferation through transcriptional control of a Myc- and Wnt-related developmental program. PLoS Genet. 2008; 4:e10.


61. Park JI, Venteicher AS, Hong JY, Choi J, Jun S, Shkreli M, et al. Telomerase modulates Wnt signalling by association with target gene chromatin. Nature. 2009; 460:66–72.


62. Gizard F, Heywood EB, Findeisen HM, Zhao Y, Jones KL, Cudejko C, et al. Telomerase activation in atherosclerosis and induction of telomerase reverse transcriptase expression by inflammatory stimuli in macrophages. Arterioscler Thromb Vasc Biol. 2011; 31:245–252.


63. Mitchell JR, Wood E, Collins K. A telomerase component is defective in the human disease dyskeratosis congenita. Nature. 1999; 402:551–555.


64. Shkreli M, Sarin KY, Pech MF, Papeta N, Chang W, Brockman SA, et al. Reversible cell-cycle entry in adult kidney podocytes through regulated control of telomerase and Wnt signaling. Nat Med. 2011; 18:111–119.


65. Ghosh A, Saginc G, Leow SC, Khattar E, Shin EM, Yan TD, et al. Telomerase directly regulates NF-κB-dependent transcription. Nat Cell Biol. 2012; 14:1270–1281.


66. Ahmed S, Passos JF, Birket MJ, Beckmann T, Brings S, Peters H, et al. Telomerase does not counteract telomere shortening but protects mitochondrial function under oxidative stress. J Cell Sci. 2008; 121(Pt 7):1046–1053.


67. Maida Y, Masutomi K. RNA-dependent RNA polymerases in RNA silencing. Biol Chem. 2011; 392:299–304.


68. Maida Y, Yasukawa M, Furuuchi M, Lassmann T, Possemato R, Okamoto N, et al. An RNA-dependent RNA polymerase formed by TERT and the RMRP RNA. Nature. 2009; 461:230–235.


69. Haendeler J, Dröse S, Büchner N, Jakob S, Altschmied J, Goy C, et al. Mitochondrial telomerase reverse transcriptase binds to and protects mitochondrial DNA and function from damage. Arterioscler Thromb Vasc Biol. 2009; 29:929–935.


70. Herrera E, Samper E, Martín-Caballero J, Flores JM, Lee HW, Blasco MA. Disease states associated with telomerase deficiency appear earlier in mice with short telomeres. EMBO J. 1999; 18:2950–2960.

