Abstract
Purpose
We hypothesized that parenteral nutrition associated cholestasis (PNAC) would be more severe in small for gestational age (SGA) compared with appropriate for gestational age (AGA) very low birth weight (VLBW) infants.
Materials and Methods
Sixty-one VLBW infants were diagnosed as PNAC with exposure to parenteral nutrition with elevation of direct bilirubin ≥2 mg/dL for ≥14 days. Twenty-one SGA infants and 40 AGA infants matched for gestation were compared.
Results
Compared with AGA infants, PNAC in SGA infants was diagnosed earlier (25±7 days vs. 35±14 days, p=0.002) and persisted longer (62±36 days vs. 46±27 days, p=0.048). Severe PNAC, defined as persistent elevation of direct bilirubin ≥4 mg/dL for more than 1 month with elevation of liver enzymes, was more frequent in SGA than in AGA infants (61% vs. 35%, p=0.018). The serum total bilirubin and direct bilirubin levels during the 13 weeks of life were significantly different in SGA compared with AGA infants. SGA infants had more frequent (76% vs. 50%, p=0.046), and persistent elevation of alanine aminotransferase.
Parenteral nutrition (PN) is essential in small premature infants during the critical early neonatal period to promote the growth and development. However, prolonged PN is associated with hepatobiliary dysfunction, particularly cholestasis. In very low birth weight (VLBW) infants, the rates of parenteral nutrition associated cholestasis (PNAC) have been reported to be as high as 50%.1
Although the mechanism is unclear, several risk factors for cholestasis have been identified, namely, immaturity of the biliary excretory system, absence of oral feeding, bacterial overgrowth or sepsis and cumulative high intake of amino acids and lipids.2-4
The infants who are small for gestational age (SGA) or growth restricted are known to have a number of metabolic abnormalities related to the liver as compared with those who are appropriate for gestational age (AGA).5,6 SGA infants exposed to PN have been reported to have more severe liver damage than AGA infants.7 Robinson and Ehrenkranz8 reported that SGA is an independent risk factor for PNAC, and suggested that SGA infants require less PN for cholestasis to develop. However, they did not focus on whether the clinical course and hepatic dysfunction would be different in SGA compared with AGA infants. In a recent report by Costa, et al.,9 SGA infants were not at higher risk of PNAC, and enteral intake during the first three weeks of life and oxygen therapy were the best predictors of PNAC.
In this study, we aim to identify whether SGA infants with PNAC would be different in clinical severity with hepatic dysfunction compared with AGA VLBW infants, asuming the altered hepatic metabolism in growth restricted condition.
Among VLBW infants admitted to the Severance Children's Hospital NICU from 2000 to 2007 and exposed to PN for more than 14 days, there were 21 SGA infants who fulfilled our preselection criteria of PN exposure. SGA was defined as a birth weight below the 10th percentile for gestational age on a fetal growth chart.10 Forty AGA infants who had the same gestation age as the SGA infants were selected for comparison as defined in blocks of one week. Exclusion criteria were cholestasis attributable to congenital infection, anatomic obstruction of the hepatobiliary tract, inherited metabolic disorders and congenital gastrointestinal disorders. Cholestasis was defined as the peak serum direct bilirubin greater than 2.0 mg/dL during the entire course of PN. All infants with cholestasis underwent liver ultrasound and testing for infectious hepatitis. In addition, severe PNAC was defined as the elevation of direct bilirubin greater than 4.0 mg/dL for 1 month and as elevation of aspartate aminotransferase (AST) and alanine aminotransterase (ALT) greater than 60 IU/L and 35 IU/L, respectively.
As for co-morbidities, bronchopulmonary dysplasia (BPD by NIH classification), late onset sepsis (diagnosed after 72 hours of life, culture proven in association with signs of systemic inflammatory response syndrome such as fever, low peripheral skin temperature, hypotension and unstable vital signs), necrotizing enterocolitis (NEC by the modified Bell's staging criteria), intraventricular hemorrhage (IVH included higher than grade 2 confirmed by brain ultrasonography or brain magnetic resonance imaging), periventricular leucomalacia (PVL confirmed by brain magnetic resonance imaging), retinopathy of prematurity (ROP classified according to the International Classification of Acute Retinopathy of Prematurity) were collected.
PN was started within the first 48 hours of life with 6% amino acid solution (6% Aminosteril Ped®, Boryung Pharma Corp, Seoul, Korea, or Trophamine®, Choongwae Pharma Corp, Seoul, Korea) and 20% lipid solution (Lipision®, Choongwae Pharma Corp, Seoul, Korea). The starting dose of amino acid or lipid was 0.5 g/kg/day, and doses were increased by 0.5 g/kg/day until reaching 3.0 g/kg/day. Dextrose was initiated on the first day, with glucose infusion rate of 6-8 mg/kg/min and advanced to a maximum of 12-14 mg/kg/min when needed. The volumes and durations of enteral feedings were dependent on the individual infants' ability to tolerate feedings. Liver function tests, total bilirubin and direct bilirubin were checked at weekly intervals to monitor for PN complications. With the diagnosis of PNAC, maximal daily infusion of amino acid was lowered to less than 2 g/kg/day. When hypertriglycemia developed (TG >150 mg/dL), the maximal daily infusion of lipid was reduced to less than 2 g/kg/day. All medication metabolized by liver were analyzed.
Statistical analysis was done with the Student t-test and chi-square analysis for means and frequencies. Numeric data are presented as mean and standard deviation because the data were normally distributed. Serum levels of bilirubin at each week were analyzed using repeated measures ANOVA. Calculations were performed with SPSS software version 17.0 (SPSS Inc., Chicago, IL, USA). p-values less than 0.05 were considered statistically significant.
Severe PNAC developed in 61% of SGA infants, significantly higher than 35% of AGA infants (OR=3.0, 95% CI, 1.03-9.02, p=0.018) (Table 1). Maternal pregnancy induced hypertension was more frequent in SGA than in AGA infants (67% vs. 25%, p=0.011). Common neonatal illnesses such as hyaline membrane disease (HMD), BPD, patent ductus arteriosus, sepsis, NEC, ROP, IVH and PVL were not significantly different between the groups (Table 2). There were no differences between groups for the days from birth to starting PN, days from birth to starting enteral feedings, and age at which enteral nutrition reached 60 or 120 kcal/kg/day. The duration of PN exposure, cumulative dose of parenteral amino acids and lipids, and average amounts of amino acid and lipid were not significantly different between the two groups (Table 3).
When the SGA infants were compared with the AGA infants, the mean age at diagnosis of cholestasis detected was significantly earlier (p=0.002), and the mean duration of cholestasis was significantly longer in SGA infants (p=0.048). In SGA infants, postconceptional age of cholestasis detected was 32.4±2.1 wks compared to 32.4±2.1 wks of AGA infants with no significant difference. The mean level of peak direct bilirubin tends to be high in SGA compared with AGA infants, but the group difference was not significant. In SGA infants, the days when serum direct bilirubin levels reached the maximum and were normalized were not significantly different. SGA infants had more frequent AST elevation (OR 4.0, 95% CI 1.02-15.81, p=0.048) and ALT elevation (OR 3.2, 95% CI 1.01-10.45, p=0.039), and longer duration of ALT elevation than AGA infants (p=0.046) (Table 4).
In the VLBW infants with severe PNAC, the serum total bilirubin and serum direct bilirubin levels were plotted over 13 weeks of the study period. Using the repeated measured ANOVA, the serum total bilirubin was shown to be significantly different between the SGA and AGA infants over the 13 weeks of life (p<0.01). Also, serum direct bilirubin was significantly different between SGA and AGA infants over the 13 weeks of life (p<0.01) (Fig. 1).
In the present study, we found that SGA infants have a different clinical course of PNAC compared with AGA infants, especially in the severity of PNAC and hepatic dysfunction. In SGA infants, PNAC is diagnosed earlier and persisted longer, and severe PNAC occurred more frequently compared with AGA infants. The serum total bilirubin and direct bilirubin levels during the 13 weeks of life were significantly different between SGA and AGA infants. This difference in clinical pattern would be important in caring for infants with PNAC.
In 1970's, PNAC was reported in 50% of the infants weighing less than 1000 g. After 2 to 4 weeks of PN exposure, PNAC occurred in 15% of the infants weighing between 1000 g and 2000 g.1 The rate is increased to 67% after 3 months of PN exposure. An overall associated mortality ranged from 3% to 14% due to hepatic failure and sepsis.11
Multifactorial risk factors for the development of PNAC include prematurity, lack of enteral feeding, prolonged duration of PN and bacterial overgrowth.12-14 The relation between the risk of PNAC and gestational age may be due to physiological immaturity of neonatal liver, and impaired hepatic transportation and metabolism of bile acids in prematurity.4 The decreased enterohepatic circulation, intestinal hormone and enzyme secretion by enteral starvation lead to further decreases in bile flow, causing cholestasis.4 Sepsis-related damage to hepatocytes is triggered by lipopolysaccharides of endotoxins, and cytokines induces hepatotoxicity and 30% increase in the bilirubin level.14,15 In addition, liver toxicity may result from PN components such as amino acid, lipid, and other materials.2,16 Phytosterols contained in lipid emulsions may also have a deleterious effect on biliary secretions, and intravenous infusions of amino acids result in decreased bile output because hepatocytes preferentially take up sodium-dependent amino acids to bile salts.17 Increased dextrose concentrations in the PN cause alterations in hepatic morphology and plasma levels of insulin and glucagon in adult rats.18 We did not find the difference in PN components between SGA and AGA infants in regard with liver injury. Both of them showed no difference in the use of medications which are metabolized by liver. VLBW Infants with SGA have higher mortality rates than AGA infants and are at significant risk for reduced postnatal growth and development as well as acute and chronic morbidities such as HMD, BPD and ROP.5,19-21 Some studies showed SGA as a risk factor for PNAC,7 whereas others not a risk factor for PNAC.8 In our study, severe PNAC developed three times greater in SGA infants than AGA infants. Furthermore, we noted the difference of clinical course with hepatobilliary dysfunction in SGA infants, and found that SGA infants had a higher risk for severe PNAC.
Because the mechanisms for the development of PNAC are not fully understood, investigations into the pathways in SGA infants may reveal important information about the pathophysiology and potential effective therapies of PNAC. Impaired perfusion of the liver in utero may affect the hepatocyte function and tolerance to feedings in the very preterm infant.7 SGA infants may have decreased capacity to utilize or metabolize protein and may be more sensitive to excessive protein load compared to AGA infants, possibly leading to hepatocellular dysfunction.6 Fetal rat with intrauterine growth restriction has altered hepatic fatty acid metabolism ratios.22,23 Increased level of glucose uptake may also alter glucose transporter expression and glucose homeostasis in liver.24 In addition, altered metabolism of copper and insufficient antioxidant activity in the liver were suggested as contributing factors in the development of cholestasis in SGA infants.25,26 A strong association between maternal hypertensive disorders and reduced fetal growth, possibly due to vasculopathy with resulting uteroplacental insufficiency, has been identified.27 However, there is no significant difference in the clinical course of PNAC between the babies with and without pregnancy induced hypertension mother.
In the typical course of liver toxicity in premature infants receiving PN, serum direct bilirubin levels reaches the peak levels at about 40 days of life, and serum ALT reaches the peak levels at about 40-60 days of life, and the level of direct bilirubin falls after increasing enteral feeding.4 In our study, clinical course of hepatic dysfunction showed that direct bilirubin reached the peak levels at about 40 days in both groups and normalized at about 80 days. Increase of direct bilirubin in SGA infants tended to be detected earlier, and prolonged longer, but without significance. AST/ALT elevation was noted for about 85 days and SGA infants had longer duration of elevation than AGA infants.
Although a resolution of the jaundice and a normalization of liver enzymes may occur after the withdrawal of PN and the initiation of enteral feeding, some infants may have persistent liver disease or hepatic failure. To date, several pharmacological agents have been marketted, but no conclusive benefits have been documented. Ursodeoxycholic acid (UDCA) can decrease the duration and extent of jaundice and shorten the clinical course of PNAC in VLBW nonsurgical patients while other biochemical parameters of liver function are not changed.28 We also treat all patients with UDCA. Recently, the potential benefits of providing fish oils for both treatment and prevention of PNAC are supported by experimental data.29 In the present study, fish oil-based lipid formulation was not adopted yet, further consideration will be needed.
Limitations of this study may be a small sample size, retrospective design, and multifactorial factors of prematurity in relation to PNAC.
In conclusion, SGA infants had earlier onset and longer duration of PNAC with hepatic dysfunction compared with AGA infants. Since clinical course of PNAC is more persistent and severe in SGA infants, careful monitoring and treatment are required to reduce clinical severity of PNAC and possibly to prevent hepatic failure.
Figures and Tables
Fig. 1
Comparison of serum total bilirubin (A) and direct bilirubin (B) levels from SGA and AGA infants with PNAC. There was a significant difference in the serum levels of total bilirubin (p<0.01) and direct bilirubin (p<0.01) levels of SGA infants compared with AGA infants by repeated measured ANOVA. SGA, small for gestational age; AGA, appropriate for gestational age; PNAC, parenteral nutrition associated cholestasis; ANOVA, analysis of variance.
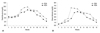
Table 2
Co-Morbid Factors of VLBW Infants with PNAC between SGA and AGA Infants
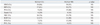
VLBW, very low birth weight; PNAC, parenteral nutrition associated cholestasis; SGA, small for gestational age; AGA, appropriate for gestational age; HMD, hyaline membrane disease; BPD, bronchopulmonary dysplasia; PDA, patent ductus arteriosus; NEC, necrotizing enterocolitis; ROP, retinopathy of prematurity; IVH, intraventricular hemorrhage; PVL, periventricular leukomalacia; NS, not significant.
Results are number of subject (%).
ACKNOWLEDGEMENTS
This research was supported by Basic Science Research Program through the National Research Foundation of Korea funded by the Ministry of Education, Science and Technology (No. 2011-0014207).
References
1. Beale EF, Nelson RM, Bucciarelli RL, Donnelly WH, Eitzman DV. Intrahepatic cholestasis associated with parenteral nutrition in premature infants. Pediatrics. 1979; 64:342–347.


2. Shin JI, Namgung R, Park MS, Lee C. Could lipid infusion be a risk for parenteral nutrition-associated cholestasis in low birth weight neonates? Eur J Pediatr. 2008; 167:197–202.


3. Steinbach M, Clark RH, Kelleher AS, Flores C, White R, Chace DH, et al. Demographic and nutritional factors associated with prolonged cholestatic jaundice in the premature infant. J Perinatol. 2008; 28:129–135.


4. Merritt RJ. Cholestasis associated with total parenteral nutrition. J Pediatr Gastroenterol Nutr. 1986; 5:9–22.


6. Boehm G, Müller DM, Teichmann B, Krumbiegel P. Influence of intrauterine growth retardation on parameters of liver function in low birth weight infants. Eur J Pediatr. 1990; 149:396–398.


7. Baserga MC, Sola A. Intrauterine growth restriction impacts tolerance to total parenteral nutrition in extremely low birth weight infants. J Perinatol. 2004; 24:476–481.


8. Robinson DT, Ehrenkranz RA. Parenteral nutrition-associated cholestasis in small for gestational age infants. J Pediatr. 2008; 152:59–62.


9. Costa S, Maggio L, Sindico P, Cota F, De Carolis MP, Romagnoli C. Preterm small for gestational age infants are not at higher risk for parenteral nutrition-associated cholestasis. J Pediatr. 2010; 156:575–579.


10. Lubchenco LO, Hansman C, Boyd E. Intrauterine growth in length and head circumference as estimated from live births at gestational ages from 26 to 42 weeks. Pediatrics. 1966; 37:403–408.


11. Kubota A, Yonekura T, Hoki M, Oyanagi H, Kawahara H, Yagi M, et al. Total parenteral nutrition-associated intrahepatic cholestasis in infants: 25 years' experience. J Pediatr Surg. 2000; 35:1049–1051.


12. Btaiche IF, Khalidi N. Parenteral nutrition-associated liver complications in children. Pharmacotherapy. 2002; 22:188–211.


13. Sondheimer JM, Asturias E, Cadnapaphornchai M. Infection and cholestasis in neonates with intestinal resection and long-term parenteral nutrition. J Pediatr Gastroenterol Nutr. 1998; 27:131–137.


14. Carter BA, Shulman RJ. Mechanisms of disease: update on the molecular etiology and fundamentals of parenteral nutrition associated cholestasis. Nat Clin Pract Gastroenterol Hepatol. 2007; 4:277–287.


15. Beath SV, Davies P, Papadopoulou A, Khan AR, Buick RG, Corkery JJ, et al. Parenteral nutrition-related cholestasis in postsurgical neonates: multivariate analysis of risk factors. J Pediatr Surg. 1996; 31:604–606.


16. Willis TC, Carter BA, Rogers SP, Hawthorne KM, Hicks PD, Abrams SA. High rates of mortality and morbidity occur in infants with parenteral nutrition-associated cholestasis. JPEN J Parenter Enteral Nutr. 2010; 34:32–37.


17. Bucuvalas JC, Goodrich AL, Blitzer BL, Suchy FJ. Amino acids are potent inhibitors of bile acid uptake by liver plasma membrane vesicles isolated from suckling rats. Pediatr Res. 1985; 19:1298–1304.


18. Li S, Nussbaum MS, Teague D, Gapen CL, Dayal R, Fischer JE. Increasing dextrose concentrations in total parenteral nutrition (TPN) causes alterations in hepatic morphology and plasma levels of insulin and glucagon in rats. J Surg Res. 1988; 44:639–648.


19. Aucott SW, Donohue PK, Northington FJ. Increased morbidity in severe early intrauterine growth restriction. J Perinatol. 2004; 24:435–440.


20. Bernstein IM, Horbar JD, Badger GJ, Ohlsson A, Golan A. Morbidity and mortality among very-low-birth-weight neonates with intrauterine growth restriction. The Vermont Oxford Network. Am J Obstet Gynecol. 2000; 182(1 Pt 1):198–206.


21. Regev RH, Lusky A, Dolfin T, Litmanovitz I, Arnon S, Reichman B. Israel Neonatal Network. Excess mortality and morbidity among small-for-gestational-age premature infants: a population-based study. J Pediatr. 2003; 143:186–191.


22. Lane RH, Flozak AS, Ogata ES, Bell GI, Simmons RA. Altered hepatic gene expression of enzymes involved in energy metabolism in the growth-retarded fetal rat. Pediatr Res. 1996; 39:390–394.


23. Lane RH, Tsirka AE, Gruetzmacher EM. Uteroplacental insufficiency alters cerebral mitochondrial gene expression and DNA in fetal and juvenile rats. Pediatr Res. 2000; 47:792–797.


24. Lane RH, Crawford SE, Flozak AS, Simmons RA. Localization and quantification of glucose transporters in liver of growth-retarded fetal and neonatal rats. Am J Physiol. 1999; 276(1 Pt 1):E135–E142.
25. Cordano A. Clinical manifestations of nutritional copper deficiency in infants and children. Am J Clin Nutr. 1998; 67:5 Suppl. 1012S–1016S.


26. Peña MM, Lee J, Thiele DJ. A delicate balance: homeostatic control of copper uptake and distribution. J Nutr. 1999; 129:1251–1260.


27. Piper JM, Langer O, Xenakis EM, McFarland M, Elliott BD, Berkus MD. Perinatal outcome in growth-restricted fetuses: do hypertensive and normotensive pregnancies differ? Obstet Gynecol. 1996; 88:194–199.

