Abstract
Purpose
Hyperoxia has the chief biological effect of cell death. We have previously reported that cathepsin B (CB) is related to fetal alveolar type II cell (FATIIC) death and pretreatment of recombinant IL-10 (rIL-10) attenuates type II cell death during 65%-hyperoixa. In this study, we investigated what kinds of changes of CB expression are induced in FATIICs at different concentrations of hyperoxia (65%- and 85%-hyperoxia) and whether pretreatment with rIL-10 reduces the expression of CB in FATIICs during hyperoxia.
Materials and Methods
Isolated embryonic day 19 fetal rat alveolar type II cells were cultured and exposed to 65%- and 85%-hyperoxia for 12 h and 24 h. Cells in room air were used as controls. Cytotoxicity was assessed by lactate dehydrogenase (LDH) released into the supernatant. Expression of CB was analyzed by fluorescence-based assay upon cell lysis and western blotting, and LDH-release was re-analyzed after preincubation of cathepsin B-inhibitor (CBI). IL-10 production was analyzed by ELISA, and LDH-release was re-assessed after preincubation with rIL-10 and CB expression was re-analyzed by western blotting and real-time PCR.
Results
LDH-release and CB expression in FATIICs were enhanced significantly in an oxygen-concentration-dependent manner during hyperoxia, whereas caspase-3 was not activated. Preincubation of FATIICs with CBI significantly reduced LDH-release during hyperoxia. IL-10-release decreased in an oxygen-concentration-dependent fashion, and preincubation of the cells with rIL-10 significantly reduced cellular necrosis and expression of CB in FATIICs which were exposed to 65%- and 85%-hyperoxia.
Hyperoxia is a mainstay in the treatment of preterm infants with respiratory distress. However, hyperoixa has the major biological effects of inducing cell death and the inflammatory response, through which lung injury is generated. Hyperoxia-induced lung injury is a major contributing factor to the evolution of bronchopulmonary dysplasia in preterm infants.1
Excessive oxygen radicals generated by hyperoxia are stimuli for lysosomal permeabilization, which allows the release of lysosomal proteases.2 Recently, there have been growing concerns regarding lysosome-mediated cell death via non-caspase proteases released through lysosomal permeabilization,3-5 and cathepsins have gained the most attention among these proteases.6
Lung damage from increased intracellular oxygen radicals is accompanied by a secondary inflammatory response of the lungs.7 These effects are orchestrated by cytokines which amplify inflammatory cell influx into the lung.8 Currently, there is growing concern that an inability to regulate inflammation could be a factor in the susceptibility of preterm infants to bronchopulmonary dysplasia.9
Recently, "new" features of bronchopulmonary dysplasia (BPD) has gained the greatest concern in preterm newborns. "New""BPD" is characterized by marked alveolar and capillary hypoplasia,10 leading to developmental arrest of the lungs,11 differently from the patterns of atelectasis, overinflation and extensive fibroproliferation observed in "old" "BPD".10 And there is a remarkable difference in clinical features between "old" and "new""BPD" as well. "new" "BPD" is evoluted with a few days' supplementation of hyperoxia since birth, whereas "old""BPD" usually develops in infants exposed to long term of hyperoxia more than 28 days.11 These clinical natures indicate that lung injury may be generated from the acute stage of hyperoxia, which may play an important role in evoluting "new""BPD" in preterm newborns.
We have previously shown that fetal alveolar type II cell death is largely mediated by necrosis during the acute stage of 65%-hyperoxia, and fetal alveolar type II cell necrosis is mediated by cathepsin B, but not caspase-3, during the acute stage of 65%-hyperoxia.12 Also we have previously defined that an imbalance between the pro-inflammatory cytokine, IL-8 and the anti-inflammatory cytokine, IL-10 is generated in fetal alveolar type II cells exposed to 65%-hyperoxia, and that preincubation of fetal alveolar type II cells with recombinant IL-10 (rIL-10) prior to hyperoxia reduces cell necrosis and release of IL-8 in these cells during hyperoxia.13
Although there has been one report showing that IL-10 tends to decrease cathepsin B gene expression in adult lung epithelial cells,14 there have been no studies performed to investigate the relationship between IL-10 and cathepsin B in fetal alveolar type II cells with the stimulus of hyperoxia. Based on our previous studies and the findings of others', we hypothesized that IL-10 might inhibit cathepsin B expression in fetal alveolar type II cells exposed to hyperoxia. In this study, we investigated the expressions of cathepsin B at different concentrations of hyperoxia and the effect of preincubation with rIL-10 on cathepsin B expression in fetal alveolar type II cells exposed to different concentrations of hyperoxia.
Fetal rat lungs were achieved from time-pregnant Sprague-Dawley rats (Daehan Biolink, Eumseong, South Korea) on embryonic day 19 (E19) (term=22 days). Animal care and experimental procedures were carried out in accordance with the Guidelines for Animal Experimentation of Kangwon National University School of Medicine with the approval of the Institutional Animal Care. After extraction of fetal lungs and isolation of fetal type II cells as described previously,12,13,15,16 type II epithelial cells were harvested with 0.25% (wt/vol) trypsin in 0.4 mM EDTA and plated at a density of 10×105 cells/well on 6-well plates precoated with fibronectin (4 µg/well). Plates containing cells were incubated for an additional 24 h in serum-free DMEM and then incubated in a culture chamber with ProOx Oxygen Controller with Low profile right angle sensor (BioSpherix, Redfield, NY, USA). 65%- and 85%-hyperoxia was applied for 12 h and 24 h, and cells grown in room air (5% CO2) were treated in an identical manner and served as controls. For the study of preincubation of rIL-10, the cultured type II cells were treated with rIL-10 (R&D Systems, Minneapolis, MN, USA), at a concentration of 250 ng/mL,13 for 1 h before hyperoxia exposure. And for inhibition studies, the cells in an identical manner were preincubated with specific inhibitor of cathepsin B, CA074Me (Calbiochem, San Diego, CA, USA), at a concentration of 100 µM3,12 for 1 h prior to 65%-hyperoxia. The concentrations of 250 ng/mL of rIL-1013 and 100 µM cathepsin B-inhibitor3,12 were chosen based on previous studies, which showed that these concentrations greatly reduced cell death in fetal alveolar type II cells.12,13
LDH-release into the supernatant upon cell lysis was measured using a CytoTox 96® non-radioactive cytotoxicity assay (Promega, Madison, WI, USA) as descried previously.13
Cathepsin B activity was measured using Cathepsin B Assay Kit (BioVision, Mountain View, CA, USA) as described previously.13
Western blottings of cathepsin B and caspase-3 were processed as described previously.13
Total RNA was extracted from E19 FATIICs exposed to 65%- and 85%-hyperoxia for 12 h and 24 h or parallel normoxic samples by a single-step method and purified further with the Rneasy Mini Kit (Invitrogen, Carlsbad, CA, USA) as described previously.13
Results are expressed as means±SD from three experiments, using different litters for each experiment. Unpaired Student's t-test was used to analyze the intergroup comparisons, and analysis of variance was used to compare for LDH-release, IL-10 production and activity of cathepsin B between time points within each oxygen concentration. p-value <0.05 was considered statistically significant.
As shown in Fig. 1A, cellular necrosis increased in an oxygen-concentration-dependent manner during 65%- and 85%-hyperoxia (Fig. 1A). Cell lysis analyzed by LDH-release into the supernatant significantly increased 1.6-fold and 2.1-fold after 12 h of 65%- and 85%-hyperoxia, respectively (control=11.1±0.57 vs. 65%-hyperoxia=18.0±1.50 vs. 85%-hyperoxia=24.5±1.73; p<0.01), and increased 2.4-fold and 3.2-fold after 24 h of 65%- and 85%-hyperoxia, respectively compared to controls (control=13.8±0.25 vs. 65%-hyperoxia=32.7±2.95 vs. 85%-hyperoxia=42.7±1.87; p<0.01) (Fig. 1A). Activity of cathepsin B was analyzed by fluorescence-based assay upon cell lysis, and found to decrease in an oxygen-concentration-dependent manner (Fig. 1B). Fig. 1B shows that the activity of cathepsin B significantly increased: 1.8-fold and 2.8-fold after 12 h of 65%- and 85%-hyperoxia, respectively (control=2609.0±361.1 vs. 65%-hyperoxia=4703.3±252.7 vs. 85%-hyperoxia=7284.1±384.7; p<0.01), and increased 1.7-fold and 3.7-fold after 24 h of 65%- and 85%-hyperioxia, respectively compared to controls (control=5423.7±695.8 vs. 65%-hyperoxia=9371.3±1265.9 vs. 85%-hyperoxia=19952.3±165.9; p<0.01). IL-10 released into the supernatant was analyzed by ELISA. In contrast to the results of LDH-release and cathepsin B activity, IL-10 production significantly decreased in an oxygen-concentration-dependent manner (Fig. 1C). As shown in Fig. 1C, IL-10 decreased significantly by 24% and 40% after 12 h of 65%- and 85%-hyperoxia, respectively (control=100.3±8.50 vs. 65%-hyperoxia=77.7±3.79 vs. 85%-hyperoxia=61.7±4.73; p<0.05), and decreased by 46% and 75% after 24 h of 65%- and 85%-hyperoxia, respectively compared to controls (control=110.7±10.50 vs. 65%-hyperoxia=59.3±2.52 vs. 85%-hyperoxia=27.7±2.08; p<0.01) (Fig. 1C).
Based on the above findings showing that 65%- and 85%-hyperoxia increased cell necrosis and cathepsin B activity (Fig. 1B), we investigated whether preincubation of FATIICs with cathepsin B-inhibitor inhibits type II cell necrosis. Thus, E19 fetal type II cells were incubated with specific inhibitor of cathepsin B, CA074Me (Calbiochem, San Diego, CA, USA), at a concentration of 100 µM for 1 h13 prior to exposure to 65%- and 85%-hyperoxia. As shown in Fig. 2, addition of cathepsin B-inhibitor significantly reduced cell cytotoxicity (as measured by LDH-release) by 32% and 37% after 12 h of 65%- and 85%-hyperoxia, respectively (65%-hyperoxia=18.0±1.80 vs. inhibitor-treated=12.2±0.48; p<0.01; 85%-hyperoxia=24.5±1.73 vs. inhibitor-treated=16.7±0.56; p<0.01), and decreased by 44% and 37% after 24 h of 65%- and 85%-hyperoxia, respectively compared to untreated cells (65%-hyperoxia=32.7±2.95 vs. inhibitor-treated=18.3±0.45; p<0.01; 85%-hyperoxia=42.7±1.87 vs. inhibitor-treated=26.8±1.75; p<0.01) (Fig. 2).
Based on above-described observations showing that 65%- and 85%-hyperoxia decreased IL-10 production (Fig. 1C), we then evaluated whether preincubation of the cells with rIL-10 decreases type II cell necrosis. Thus, E19 fetal type II cells were incubated 250 ng/mL rIL-10 for 1 h13 prior to exposure to 65%- and 85%-hyperoxia. As shown in Fig. 2, preincubation of the cells with rIL-10 significantly reduced cell cytotoxicity (as measured by LDH-release) by 21% and 28% after 12 h of 65%- and 85%-hyperoxia, respectively (65%-hyperoxia=18.0±1.80 vs. rIL-10-treated=14.4±0.76; p<0.05; 85%-hyperoxia=24.5±1.73 vs. rIL-10-treated=17.7±0.53; p<0.05) and decreased by 37% and 30% after 24 h of 65%- and 85%-hyperoxia, respectively compared to untreated cells (65%-hyperoxia=32.7±2.95 vs. rIL10-treated=20.6±0.25; p<0.01; 85%-hyperoxia=42.7±2.29 vs. rIL10-treated=29.7±1.06; p<0.01) (Fig. 2).
Western blots were performed in three experimental sets and the result showed visibly increased expressions of cathepsin B in fetal alveolar type II cells exposed to 65%- and 85%-hyperoxia for 12 h and 24 h when compared to controls (Fig. 3A). And relative intensities of the blots significantly increased 4.4-fold and 6.0-fold after 12 h of 65%- and 85%-hyperoxia, respectively and increased 3.7-fold and 4.5-fold after 24 h of 65%- and 85%-hyperoxia, respectively (Fig. 3B).
Cleaved caspase-3 as a key executioner in apoptosis was assessed by western blotting. Western blots for two experimental sets showed that the level of caspase-3 was not enhanced during 65%- and 85%-hyperoxia compared to controls, and the abundance of full-length procaspase-3 did not concomitantly decrease in hyperoxic samples compared to controls (Fig. 4).
We evaluated whether preincubation of the cells with rIL-10 prior to hyperoxia would attenuate cathepsin B expression in fetal alveolar type II cells when subsequently exposed to 65%- and 85%-hyperoxia. Thus, E19 type II cells were preincubated with 250 ng/mL of rat rIL-10 for 1 h prior to hyperoxia.13 Fig. 5A shows that the expression of cathepsin B was decreased visibly in treated cells compared to untreated cells during 65%- and 85%-hyperoxia. The relative intensities decreased by 40% and 70% after 12 h and 24 h of 65%-hyperoxia, respectively, and decreased by 42% and 80% after 12 h and 24 h of 85%-hyperoxia, respectively in treated cells compared to untreated cells (Fig. 5B). As shown in Fig. 5C, the addition of IL-10 reduced gene expression of cathepsin B by 9% and 16% after 12 h and 24 h of 65%-hyperoxia, respectively, and reduced by 13% and 24% after 12 h and 24 h of 85%-hyperoxia, respectively compared to untreated cells (Fig. 5C).
The main findings of the present study are that cathespin B was enhanced in an oxygen-concentration-dependent manner in fetal alveolar type II cells during the acute stage of hyperoxia, and preincubation with rIL-10 prior to hyperoxia significantly reduced cathepsin-B expression induced in fetal alveolar type II cells during hyperoxia.
In the current study, we utilized a 65%- and 85%-hyperoxia regimen, which was based on previous evidences showing that exposure of newborn mice to 65%-hyperoxia led to impairment of lung architectures in adult mice,17 and that 85%-hyperoxia arrested alveolar and pulmonary development in neonatal rats.18
In the present study, we observed that cytotoxicity increased in an oxygen-concentration-dependent manner in fetal alveolar type II cells during the acute stage of sublethal and lethal hyperoxia. Similarly, cathepsin B was strongly activated in an oxygen-concentration-dependent fashion in fetal alveolar type II cells and increased expressions of cathespin B were in proportion to cell cytotoxicity during hyperoxia, whereas IL-10 production showed an opposite pattern. Furthermore, we found that preincubation of the cells with cathepsin B-inhibitor or rIL-10 significantly reduced cell cytotoxicity in fetal alveolar type II cells exposed to two different concentrations of hyperoxia. Taking together, these findings imply that overexpression of cathespin B is related to fetal type II cell death during hyperoxia, and failure of maintaining optimal level of IL-10 secondary to hyperoxia may affect fetal type II cell death during hyperoxia. In the present study, therefore, we evaluated whether IL-10 has any effect on inhibiting cathepsin B expression in fetal type II cells exposed to sublethal and lethal hyperoxia.
Currently, there has been growing concern regarding caspase-independent cell death via non-caspase protease released through lysosomal permeabilization. In particular, evidence is strongly accumulating for the involvement of an alternative protease, cathepsin B, in inducing cell death in other cell lines and conditions.3,5,19 Cathepsin B is ubiquitously expressed and most abundant in lysosomes and is released through lysosomal permeabilization.6 Our observation implies that oxygen radical species generated by hyperoxia should be a stimulus for lysososmal permeabilization as addressed in another report.6 However, the molecular identity of cathepsin B may vary depending on the type of cell and the applied death stimulus.20 Up to now, cathepsin B has been suggested to contribute to cell death in some systems via induction of mitochondrial membrane permeabilization, possibly via cleavage of Bid, thereby acting upsteam of the caspase cascade,20 whereas others have found that cathepsin B can act as an effector protease, downstream of caspases.20 Additionally, cathepsin B has been shown to be capable of executing cell death completely independent of the apoptotic machinery in fibrosarcoma cells.20 In the present study, caspase-3 was not activated at all in fetal alveolar type II cells exposed to 65%- and 85%-hyperoxia, which indicates that cathepsin B is a potential mediator of hyperoxia-induced cell death in fetal alveolar type II cells, with no requirement of the apoptotic cascade.
IL-10 is the most potent anti-inflammatory cytokine described to date21 with multifactorial anti-inflammatory properties including inhibition of pro-inflammatory transcription factor-κB, leading to suppressed cytokine production.22 We have previously reported that an imbalance between the pro-inflammatory chemokine, IL-8 and the anti-inflammatory cytokine, IL-10 is generated in fetal alveolar type II cells exposed to 65%-hyperoxia,13 and we observed in the current study that IL-10 failed to optimize its protective effect in an oxygen-concentration-dependent manner under hyperoxic conditions. In addition, we confirmed that pretreatment of the cells with rIL-10 had beneficial effects of augmenting cell cytotoxicity in fetal alveolar type II cells exposed to sublethal or lethal hyperoxia.
Although not statistically significant, there has been only one study so far published, showing that IL-10 at a concentration of 5 ng/mL tends to inhibit gene expression of cathepsin B in adult alveolar type II cells.14 The present study showed that IL-10 significantly attenuated the expression of cathepsin B in fetal alveolar type II cells exposed to 65%- and 85%-hyperoxia. With these results in mind, some potential mechanisms by which IL-10 leads to reduce cathepsin B expression induced by hyperoxia can be postulated. First, IL-10's inhibitory effect on nuclear factor-κB activated during hyperoxia may result in down-regulation of cathepsin B, since inhibition of nuclear factor-κB leads to a reduction of cathepsin B in human macrophages.23 Secondly, cathepsin B may be inhibited through IL-10's inhibitory effect on IL-8, because recombinant IL-8 increases activation of cathepsin B in synovial fluid in rabbits.24 Finally, inhibition of cathepsin B activity may partly be due to the fact that IL-10 may reduce the phospholipase A2 activated secondary to low doses of oxygen radical species generated by hyperoxia. As reported previously, low doses of oxygen radical species activate phospholipase A2 first and consequently lysosomal rupture indirectly through destabilization of lysosomes.6 Furthermore, in human tracheal smooth muscle cells, IL-1β-induced cytosolic phospholipase A2 expression is inhibited by pretreatment of the cells with nuclear factor κB-inhibitor.25 Consequently, it can be assumed that IL-10's inhibitory effect of nuclear factor κB might reduce phospholipase A2 expression.
In recent years, the features of "new""BPD" have been established, and "new""BPD" developes in preterm newborns exposed to low dose of high oxygen only for a few days after birth. Moreover, it has been demonstrated that failure of type II cells to proliferate during the first week of life may permanently alter postnatal lung growth,26 Hence, it is promising to define the mechanisms whereby hyperoxia on fetal type II cells contributes to the genesis of acute lung injury and to develop protective strategy to minimize acute lung injury induced from the early stage of hyperoxia.
Although the present study has the limitations of an in vitro experimental system in which E19 alveolar type II cells were isolated from their environment, the contribution of our study to the field is that the study showed that 65%- and 85%-hyperoxia could induce cell death by up-regulating cathepsin B expression in fetal alveolar type II cells from the early stage of hyperoxia, and that pretreatment with IL-10 prior to hyperoxia down-regulated the expression of cathepsin B induced in fetal alveolar type II cells exposed to 65%- and 85%-hyperoxia.
Collectively, our findings suggest that activation of cathespin B may contribute to fetal type II cell death from the early stage of sublethal and lethal hyperoxia, and cathepsin B-dependent cell death may be prevented by IL-10 in fetal alveolar type II cells from the acute stage of sublethal and lethal hyperoxia. These investigations imply that IL-10 may be a novel therapeutic strategy for preventing or limiting the extent of lung injury induced by short-term exposure of hyperoxia by inhibiting cathepsin B expression in preterm lungs susceptible to "new" bronchopulmonary dysplasia.
Figures and Tables
Fig. 1
Effect of hyperoxia on cytotoxicity, cathepsin B activity and IL-10 production. E19 type II cells exposed to 65%- and 85%-hyperoxia for the indicated periods of time. Cells in room air were used as controls. (A) Graphical depiction showing LDH-release expressed as experimental minus background LDH-release divided by maximum LDH-release in normoxia and 65%- and 85%-hyperoxic cells. The results are represented as mean±SD from 3 different experiments. (B) Graphical depiction showing activity of cathepsin B analyzed by fluorescence-based assay upon cell lysis. The results are represented as mean±SD from 3 different experiments. (C) Graphical depiction showing production of IL-10 in normoxia and 65%- and 85%-hyperoxic cells. The results are represented as mean±SD from 3 different experiments. LDH, lactate dehydrogenase; IL, interleukin; SD, standard deviation.
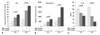
Fig. 2
Cytotoxicity of fetal type II cells after preincubation of cathepsin B-inhibitor or rIL-10. E19 type II cells exposed to 65%- and 85%-hyperoxia for the indicated periods of time. Cells in room air were used as controls. Graphical depiction showing LDH-release expressed as experimental minus background LDH-release divided by maximum LDH-release in cathepsin B-inhibitor- or rIL-10-untreated and treated cells. The results are represented as mean±SD from 3 different experiments. *p<0.01. CBI, cathepsin B-inhibitor; LDH, lactate dehydrogenase; rIL-10, recombinant IL-10; SD, standard deviation.
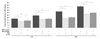
Fig. 3
Expression of cathepsin B in fetal type II cells exposed to hyperoxia. E19 type II cells exposed to 65%- and 85%-hyperoxia for the indicated periods of time. Cells in room air were used as controls. (A) Western blots showing that exposure to 65%- and 85%-hyperoxia for 12 h and 24 h increases the level of cathepsin B compared to the control samples. (B) Graphical depiction showing the relative intensities of cathepsin B from 3 independent experiments. Results are mean±SD from 3 different experiments. E19, embryonic day 19; SD, standard deviation.
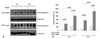
Fig. 4
Expression of caspase-3 in fetal type II cells exposed to 65%- and 85%-hyperoxia. E19 type II cells exposed to 65%- and 85%-hyperoxia for the indicated periods of time. Cells in room air were used as controls. Western blots demonstrating that exposure of E19 cells to 65%- and 85%-hyperoxia for 12 h and 24 h does not increase the level of cleaved caspase-3 and does not concomitantly decrease the abundance of full-length procaspase-3 compared to control samples. Membranes were immunoblotted with anti-cleaved caspase-3 antibody, stripped, and reprobed with full-length procaspase-3 antibody. With actin antibody to control for protein loading. E19, embryonic day 19.
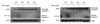
Fig. 5
IL-10 reduces cathepsin B expression in fetal type II cells exposed to hyperoxia. E19 type II cells exposed to 65%- and 85%-hyperoxia for the indicated periods of time. Cells in room air were used as controls. E19 cells were preincubated with 250 ng/mL rat recombinant IL-10 (rIL-10) before exposure to 65%- and 85%-hyperoxia. (A) Western blots showing that cathepsin B expression is reduced in rIL-10-treated cells compared to untreated cells. (B) Graphical depiction showing relative intensities of cathepsin B from 3 independent experiments in rIL-10-treated and untreated cells. (C) Graphical depiction showing gene expression of cathepsin B from 3 different experiments in rIL-10-treated and untreated cells. E19, embryonic day 19.
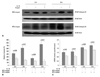
ACKNOWLEDGEMENTS
The author (HS Lee) thanks Dr. Chun-Ki Kim for his technical assistance. This work was supported by a grant from National Research Foundation (NRF) (grant No. C1007568-01-01), South Korea.
Notes
References
2. Li W, Yuan X, Nordgren G, Dalen H, Dubowchik GM, Firestone RA, et al. Induction of cell death by the lysosomotropic detergent MSDH. FEBS Lett. 2000. 470:35–39.


3. Bröker LE, Huisman C, Span SW, Rodriguez JA, Kruyt FA, Giaccone G. Cathepsin B mediates caspase-independent cell death induced by microtubule stabilizing agents in non-small cell lung cancer cells. Cancer Res. 2004. 64:27–30.


4. Layton GT, Harris SJ, Bland FA, Lee SR, Fearn S, Kaleta J, et al. Therapeutic effects of cysteine protease inhibition in allergic lung inflammation: inhibition of allergen-specific T lymphocyte migration. Inflamm Res. 2001. 50:400–408.


5. Tang PS, Tsang ME, Lodyga M, Bai XH, Miller A, Han B, et al. Lipopolysaccharide accelerates caspase-independent but cathepsin B-dependent death of human lung epithelial cells. J Cell Physiol. 2006. 209:457–467.


7. Mantell LL, Lee PJ. Signal transduction pathways in hyperoxia-induced lung cell death. Mol Genet Metab. 2000. 71:359–370.


8. Bhandari V, Elias JA. Cytokines in tolerance to hyperoxia-induced injury in the developing and adult lung. Free Radic Biol Med. 2006. 41:4–18.


9. Schultz C, Temming P, Bucsky P, Göpel W, Strunk T, Härtel C. Immature anti-inflammatory response in neonates. Clin Exp Immunol. 2004. 135:130–136.


12. Lee HS, Kim CK. Cathepsin B is activated as an executive protease in fetal rat alveolar type II cells exposed to hyperoxia. Exp Mol Med. 2011. 43:223–229.


13. Lee HS, Kim CK. Effect of recombinant IL-10 on cultured fetal rat alveolar type II cells exposed to 65%-hyperoxia. Respir Res. 2011. 12:68.


14. Gerber A, Welte T, Ansorge S, Bühling F. Expression of cathepsins B and L in human lung epithelial cells is regulated by cytokines. Adv Exp Med Biol. 2000. 477:287–292.


15. Sanchez-Esteban J, Wang Y, Gruppuso PA, Rubin LP. Mechanical stretch induces fetal type II cell differentiation via an epidermal growth factor receptor-extracellular-regulated protein kinase signaling pathway. Am J Respir Cell Mol Biol. 2004. 30:76–83.


16. Lee HS, Wang Y, Maciejewski BS, Esho K, Fulton C, Sharma S, et al. Interleukin-10 protects cultured fetal rat type II epithelial cells from injury induced by mechanical stretch. Am J Physiol Lung Cell Mol Physiol. 2008. 294:L225–L232.


17. Dauger S, Ferkdadji L, Saumon G, Vardon G, Peuchmaur M, Gaultier C, et al. Neonatal exposure to 65% oxygen durably impairs lung architecture and breathing pattern in adult mice. Chest. 2003. 123:530–538.


18. Zhu L, Li H, Tang J, Zhu J, Zhang Y. Hyperoxia arrests alveolar development through suppression of histone deacetylases in neonatal rats. Pediatr Pulmonol. 2012. 47:264–274.


19. Foghsgaard L, Wissing D, Mauch D, Lademann U, Bastholm L, Boes M, et al. Cathepsin B acts as a dominant execution protease in tumor cell apoptosis induced by tumor necrosis factor. J Cell Biol. 2001. 153:999–1010.


20. Herr I, Debatin KM. Cellular stress response and apoptosis in cancer therapy. Blood. 2001. 98:2603–2614.


21. Page TH, Smolinska M, Gillespie J, Urbaniak AM, Foxwell BM. Tyrosine kinases and inflammatory signalling. Curr Mol Med. 2009. 9:69–85.


22. Li JJ, Guo YL, Yang YJ. Enhancing anti-inflammatory cytokine IL-10 may be beneficial for acute coronary syndrome. Med Hypotheses. 2005. 65:103–106.


23. Geraghty P, Rogan MP, Greene CM, Boxio RM, Poiriert T, O'Mahony M, et al. Neutrophil elastase up-regulates cathepsin B and matrix metalloprotease-2 expression. J Immunol. 2007. 178:5871–5878.


24. Mishiro T, Nakano S, Takahara S, Miki M, Nakamura Y, Yasuoka S, et al. Relationship between cathepsin B and thrombin in rheumatoid arthritis. J Rheumatol. 2004. 31:1265–1273.
25. Lee CW, Lee IT, Lin CC, Lee HC, Lin WN, Yang CM. Activation and induction of cytosolic phospholipase A2 by IL-1beta in human tracheal smooth muscle cells: role of MAPKs/p300 and NF-kappaB. J Cell Biochem. 2010. 109:1045–1056.