Abstract
Purpose
The present study was designed to determine whether rapamycin could inhibit transforming growth factor β1 (TGF-β1)-induced fibrogenesis in primary lung fibroblasts, and whether the effect of inhibition would occur through the mammalian target of rapamycin (mTOR) and its downstream p70S6K pathway.
Materials and Methods
Primary normal human lung fibroblasts were obtained from histological normal lung tissue of 3 patients with primary spontaneous pneumothorax. Growth arrested, synchronized fibroblasts were treated with TGF-β1 (10 ng/mL) and different concentrations of rapamycin (0.01, 0.1, 1, 10 ng/mL) for 24 h. We assessed m-TOR, p-mTOR, S6K1, p-S6K1 by Western blot analysis, detected type III collagen and fibronectin secreting by ELISA assay, and determined type III collagen and fibronectin mRNA levels by real-time PCR assay.
Results
Rapamycin significantly reduced TGF-β1-induced type III collagen and fibronectin levels, as well as type III collagen and fibronectin mRNA levels. Furthermore, we also found that TGF-β1-induced mTOR and p70S6K phosphorylation were significantly down-regulated by rapamycin. The mTOR/p70S6K pathway was activated through the TGF-β1-mediated fibrogenic response in primary human lung fibroblasts.
Pulmonary fibrosis results from an exaggerated repair response to repeated lung injury. Lung injuries trigger a complex interaction of epithelial, stromal, endothelial, and inflammatory cells, resulting in abnormal accumulation of fibroblasts/myofibroblasts and excessive extracellular matrix (ECM) deposition in the pulmonary interstitium, eventually causing the loss of lung structure and function.1-5 Therefore, direct inhibition of fibroblast accumulation and extracellular matrix level may be a useful antifibrotic therapeutic target.
Rapamycin, an inhibitor of mammalian target of rapamycin (mTOR) and a potent antiproliferative drug, was introduced initially into clinical practice as a maintenance immunosuppressant to prevent transplant rejection.6 Rapamycin has also been used in the treatment of tumors and restenosis of coronary artery stents.7,8 In addition, rapamycin is attractive because its potent antifibrogenic action has been demonstrated in animal models of hepatic, renal, and pulmonary fibrosis.9-12 Rapamycin inhibits collagen production of human lung fibroblasts (WI-26) in a time- and dose-dependent manner, and decreases COL1A1, COL1A2, and COL3A1 mRNA levels.13 It plays a role by inhibiting mTOR pathway. Downstream effectors of mTOR include ribosomal protein S6 kinases (S6K) and 4 eukaryote-binding protein 1 (4E-BP1). Activation of S6K increases protein synthesis and cell growth. Alternatively, hyperphosphorylation of mTOR induces dissociation of 4E-BP1 from eukaryotic translation initiation factor (eIF4E), which facilitates mRNA translation.
Transforming growth factor β1 (TGF-β1) is a profibrotic cytokine that plays a critical role in the development of pulmonary fibrosis.14-16 Elevated levels of TGF-β1 in the lungs have been found in animal models of lung fibrosis and also in patients with idiopathic pulmonary fibrosis (IPF).17-20 TGF-β1 promotes fibroblast activation and epithelial-mesenchymal transition (EMT), followed by enhancement of collagen synthesis.21-23 TGF-β1 induces EMT through Smad signaling and non-Smad pathways.24-26 It also initiates Akt signaling through phosphoinositide 3 kinase during EMT, activating mTOR pathway, and finally controlling the translation of specific mRNA and protein synthesis involved in cell cycle regulation.11,27
The aims of this study were to find out whether ECM level in primary human lung fibroblasts was inhibited by rapamycin and to identify whether the effect of inhibition would be through the mTOR and its downstream p70S6K pathway, and confirm the activation of mTOR pathway during lung fibroblast responses to TGF-β1. To accomplish this, ECM level (includes collagen and fibronectin) and expression of mTOR pathway kinases were investigated in primary human lung fibroblasts after stimulation with TGF-β1. Then, the action of rapamycin on TGF-β1-induced ECM level and expression of mTOR pathway kinases were examined.
Adult human lung fibroblasts were mechanically dissociated from histologically normal lung tissue samples of 3 patients with primary spontaneous pneumothorax (3 male nonsmokers, mean ages 35±5 years) at the time of thoracoscopy with stapling of any air leak, and cultured. They had no evidence of other acute or chronic lung disease. The harvested lung tissue samples were maintained in sterile phosphate-buffered saline (Hyclone, Logan, UT, USA), cut into small pieces (1-5 mm3), and placed into 25-cm2 flasks (Corning, NY, USA) with Dulbecco's Modified Eagle Medium (DMEM) supplemented with 10% fetal calf serum, penicillin (100 U/mL), and streptomycin (100 µg/mL) (all from Hyclone, Logan, UT, USA). Primary fibroblasts were cultured at 37℃ in a humidified atmosphere of 5% CO2. Spindle-like fibroblasts started to growout from tissue samples on day 2 to 3. Outgrowth of fibroblasts took 1 to 2 weeks. Tissue samples were then removed by aspiration, and cells were allowed to reach confluence. Fibroblasts at confluence were expanded by trypsinization and passaged every 4 to 5 days at a 1 : 4 ratio. After 4 passages, pulmonary fibroblasts were identified by morphology and immunohistochemistry. The fibroblasts used in these experiments were obtained between passages 5 and 8.
This study was approved by the ethics committee of Beijing Chao-Yang Hospital, Capital Medical University, and informed consent was obtained from the subjects.
Cell viability was evaluated using a colorimetric assay with 3-[4,5-dimethylthiazol-2-yl]-2,5-diphenyltetrazolium bromide (MTT). Normal human lung fibroblasts were seeded onto a 96-well plate at a density of 5×104 cells/well. When the cells were nearly confluent (70%), the medium was replaced with DMEM containing 2% fetal bovine serum. TGF-β1 and rapamycin were purchased from Sigma, St. Louis, MO, USA. Rapamycin was first dissolved in dimethyl sulfoxide (DMSO), and then added to culture medium to reach the final concentrations. In the control and TGF-β1 groups, vehicle (DMSO) was added at the highest concentration (0.0004%) needed. The cells were treated with TGF-β1 (10 ng/mL) and various concentrations
of rapamycin (0.01, 0.1, 1.0, and 10 ng/mL) according to the method described previously,13 and then incubated for 24 h. Then, 20 µL of MTT (5 mg/mL) were added per well and incubated for 4 h. Finally, the medium was removed and formazan crystals were solubilized with 150 µL DMSO. The optical density of each well was measured at 490 nm. The results are based on the cleavage of tetrazolium salt by viable cells that are proportional to the number of living cells in the wells.
Fibroblasts were seeded in 100-mm2 cell culture dishes (Corning, NY, USA) and allowed to reach 70% confluence. After 24 h of serum starvation, the cultures were treated with TGF-β1 (10 ng/mL) and rapamycin (0.01, 0.1, 1.0, and 10 ng/mL) for another 24 h. Concentrations of secreted type III collagen and fibronectin were determined by ELISA in culture supernatants of fibroblasts. Type III collagen and fibronectin were measured according to manufacturer's instructions (Blue Gene, BG, Beijing, China).
Fibroblasts were grown in 25-cm2 tissue culture flasks and treated with rapamycin as described above. RNA was extracted using an RNA extraction kit (Beijing Huanya Biomedicine Technology Co., Ltd., Beijing, China) according to manufacturer's instructions. The extracted mRNA was reverse-transcribed to cDNA using oligodT and M-MLV reverse transcriptase (Beijing Tiangen Biochemical Technology Co., Ltd., Beijing, China). Quantitative real-time PCR was performed using a fluorescence quantitative PCR detection system (Hangzhou Bori Science and Technology Co., Ltd., Hangzhou, China). Annealing temperatures was 60℃ for all reactions. BioEasy SYBR Green I Real Time PCR Kit Manual (Beijing Huanya Biomedicine Technology Co., Ltd., Beijing, China) was used according to manufacturer's instructions. Relative quantification of cDNA was carried out by the method previously described.28 The primers of type III collagen and fibronectin used for the PCR reactions are shown in Table 1.
For Western blot analysis, the human lung fibroblasts were seeded in 100-mm2 cell culture dishes (Corning, NY, USA) and treated with rapamycin as described above. Total cell lysates were obtained using RIPA buffer (25 mM Tris-HCl, 150 mM NaCl, 1% NP-40, 1% sodium deoxycholate, 0.1% SDS, Thermo Scientific, Waltham, MA, USA) containing 1 : 100 phenylmethylsulfonyl fluoride and phosphatase inhibitors (Beijing Puli Lai Gene Technology Co., Ltd., Beijing, China). The supernatants obtained by centrifugation of the lysates at 12000 r/min for 10 min at 4℃ were used as cytoplasmic extracts. Protein concentrations were determined using a BCA assay kit (Pierce, ID, USA). Twenty µg of each protein were subjected to sodium dodecyl sulfate polyacrylamide gel electrophoresis and transferred to PVDF membranes (Millipore, MA, USA). The membranes were blocked for 1 h in Tris-buffered saline (10 mM Tris-HCl pH7.5 and 0.15 M NaCl) containing 0.1% (v/v) Tween 20 and 5% (w/v) nonfat dry milk, and then probed at 4℃ overnight with primary antibodies against human m-TOR (diluted 1 : 1000; Cell Signaling Technology, CST, Boston, MA, USA), p-mTOR (diluted 1 : 500; CST, Boston, MA, USA), S6K1 (diluted 1 : 1000; CST, Boston, MA, USA), p-S6K1 (diluted 1 : 250; CST, Boston, MA, USA) and β-actin (diluted 1 : 5000; California Bioscience, Coachella, CA, USA). Immunoreactive bands were detected with horseradish peroxidase-conjugated secondary antibodies (diluted 1 : 2000; Protein Tech Group, Inc., Chicago, IL, USA) and visualized by enhanced chemiluminescence detection reagents on ChemiDoc XRS (Bio-Rad, Hercules, CA, USA).
All experiments were conducted in duplicate and repeated three times. The results are representative of three independent experiments. Data are expressed as means±SD. Statistical analysis was conducted by analysis of variance followed by appropriate post hoc tests including multiple comparison tests (LSD). All analyses were performed using SPSS 11.5 statistical software package (SPSS Inc., Chicago, IL, USA) and prism software (GraphPad Software, Inc., CA, USA). Probability values <0.05 were considered statistically significant.
All primary human lung fibroblasts cells displayed typical spindle-shaped morphology under a light microscopy (Fig. 1A) and stained positive for fibronectin, vimentin, and type III collagen, but were negative for immunostaining with monoclonal antibodies raised against von Willebrand factor, pro-surfactant protein C, and α-smooth muscle actin (Fig. 1B), indicating no contamination with cells such as pulmonary artery endothelial cells, alveolar type II cells, or pulmonary artery smooth muscle cells. Fig. 1C shows positive control of pulmonary artery endothelial cells, alveolar type II cells, or pulmonary artery smooth muscle cells. All subsequent experiments were performed using subconfluent quiescent cultures of fibroblast cells between passages 5 and 8 to maintain comparability.
In this study, primary human lung fibroblasts were used instead of transformed cell lines. To ensure that the inhibition of collagen level was not due to cytotoxicity of rapamycin, primary human lung fibroblasts were incubated for 24 h with rapamycin concentrations ranging from 0.01 to 10 ng/mL, and cell viability was determined using MTT assay. No difference was found in viability between cells treated with rapamycin and TGF-β1 for 24 h and untreated cells (Fig. 2). TGF-β1 did not have any significant effect on primary fibroblast proliferation, consistent with the results of Eickelberg.29 These results indicate that rapamycin is not cytotoxic toward pulmonary fibroblasts.
To determine the effects of rapamycin on ECM level in human pulmonary fibroblasts TGF-β1-induced type III collagen and fibronectin levels were examined by ELISA. As shown in Fig. 3, TGF-β1 (10 ng/mL) increased type III collagen and fibronectin by 66% and 39%, respectively (p<0.05), while rapamycin decreased TGF-β1-induced type III collagen and fibronectin level up to 53% and 40%, respectively (p<0.05). No significant difference was found among different concentrations of rapamycin.
The effects of rapamycin on TGF-β1-induced mRNA levels of type III collagen and fibronectin in human lung fibroblasts were investigated by real-time PCR (Fig. 4). TGF-β1 increased type III collagen and fibronectin mRNA levels by 27% and 14%, respectively (p<0.05), while rapamycin decreased TGF-β1-induced type III collagen mRNA levels by up to 37% (p<0.05) and TGF-β1-induced fibronectin mRNA level by up to 47% (p<0.05). Furthermore, rapamycin (especially at higher doses) was found to significantly reduce fibronectin mRNA to a much lower than no-TGF-β1 baseline level (p<0.05), suggesting that rapamycin inhibits basal levels of fibronectin also (Fig. 4B).
To determine whether the inhibition of rapamycin on TGF-β1-induced matrix proteins in human pulmonary fibroblasts was through the mTOR-p70S6K pathway, phosphorylation levels of mTOR-Ser2448 and p70S6K-Thr389 in human lung fibroblasts were determined by Western blot. As shown in Fig. 5, treatment of the cells with 10 ng/mL TGF-β1 increased mTOR-Ser2448 and p70S6K-Thr389 phosphorylation levels by 50% and 300%, respectively. Rapamycin significantly down-regulated TGF-β1-induced mTOR-Ser2448 and p70S6K-Thr389 phosphorylation (at most 83% and 67%, respectively, p<0.05). The inhibition occurred in a dose-dependent manner. In addition, rapamycin (especially at higher doses) was found to significantly reduce mTOR mRNA to a much lower than no-TGF-β1 baseline level (Fig. 5A), suggesting that rapamycin inhibits basal levels of mTOR also. These data suggest that the mTOR pathway is activated in human pulmonary fibroblasts in response to TGF-β1 stimulation, and that rapamycin can inhibit TGF-β1-dependent mTOR signaling.
We demonstrated in the present study that rapamycin directly reduced the TGF-β1-enhanced extracellular matrix (type III collagen and fibronectin) levels and mRNA levels in primary human lung fibroblasts.
Furthermore, we showed that rapamycin exerted a clear effect on the TGF-β1-induced fibrosis model, demonstrating antifibrotic effect of rapamycin on primary human lung fibroblasts. Previous in vitro studies showed that rapamycin could inhibit extracellular matrix level in normal mouse fibroblasts or cell lines: rapamycin (0.01 ng/mL) inhibited mesangial cell type IV collagen level, a major component of mesangial matrix.30 In addition, rapamycin has been shown to reduce total type I and III collagen production of transformed fibroblast cell lines in a time- and dose-dependent manner, and to decrease COL1A1, COL1A2, and COL3A1 mRNA steady-state levels by decreasing mRNA stability, and exerting direct antifibrotic activities that affect both matrix production and degradation by fibroblasts.13
Activation of the Akt-mTOR-p70S6K signaling pathway occurs in epidermal growth factor receptor-mediated pulmonary fibrosis.11 Effects of rapamycin on different phosphorylation sites in the mTOR/p70S6K pathway have been investigated, specifically to explore the action of the drug in TGF-β1-induced fibrosis. In the present study, we showed that rapamycin reduced the mTOR-Ser2448 and p70S6K-Thr389 phosphorylation levels that occurred in parallel with the effects of rapamycin on collagen type III and fibronectin protein and mRNA levels of primary human lung fibroblasts. However, no effects were observed on S6K phosphorylation at 0.01 ng/mL (Fig. 5B), a dose at which collagen III and fibronectin secretion in culture medium was inhibited (Fig. 3). The finding shown in Fig. 5B was confirmed by the gene expression of COL3A1 mRNA (Fig. 4A). Similar results were reported by Lock, et al.;30 no effects were seen on S6K phosphorylation at 0.01 ng/mL, a dose at which collagen IV secretion was inhibited (in mesangial cells). Different results can be due to different sensitivitiy of the assays. Rapamycin inhibited the mTOR/p70S6K pathway in a dose-dependent manner. However, the effect on collagen type III and fibronectin secretion did not occur in a dose-dependent manner, and the reason remains unclear. At this point, a question arises whether downstream proteins of the mTOR pathway, such as protein phosphatase 2A or protein kinase C δ affect collagen type III and fibronectin synthesis or not? Further experiments are needed to clarify this. Nevertheless, our results suggest that rapamycin works partly through the mTOR-Ser2448/p70S6K-Thr389 pathway in TGF-β1-induced fibrosis. Taken together, possible mechanisms of rapamycin-inhibition of TGF-β1-induced collagen secretion is suggested as below. First, TGF-β1 increases collagen gene and protein expression through the mTOR/p70S6K pathways. Second, rapamycin-induced activation of the c-Jun N-terminal kinase antagonizes TGF-β1-induced collagen gene expression by preventing Smad/DNA interaction and related gene expression.13,31,32
TGF-β1-induced human pulmonary fibroblast activation was used as a model of pulmonary fibrosis in vitro, because TGF-β1 is recognized as a key cytokine in the formation and development of IPF.19 TGF-β1 influences collagen at the level of transcription and translation.33 Thus, TGF-β1 increases collagen mRNA, resulting in the increase of collagen synthesis.34 In addition, TGF-β1 decreased collagen degradation by selectively inhibiting collagenase synthesis. Although TGF-β1 had no effect on human lung fibroblast proliferation in this study, TGF-β1 increased the secretion of collagen III and fibronectin in lung fibroblasts. These results are consistent with a previous report and confirm the role of TGF-β1 in promoting fibrosis.29 In our study, TGF-β1 increased pmTOR-Ser2448 and p70S6K-389Thr expression, suggesting that TGF-β1 increases collagen gene and protein expression through mTOR/p70S6K pathways. In addition to the inhibition of exogenous TGF-β1 response, rapamycin (especially at higher doses) reduced fibronectin mRNA and mTOR-Ser2448 phosphorylation to a much lower than no-TGF-β1 baseline level, suggesting that there may be endogenous TGF-β1 activity which maintains the expression of fibronectin mRNA and mTOR-Ser2448 phosphorylation at certain levels.
In conclusion, the mTOR/p70S6K pathway is activated in TGF-β1-mediated fibrogenic response of primary human lung fibroblasts. Thus, rapamycin may effectively inhibit TGF-β1-induced type III collagen and fibronectin levels partly through mTOR/p70S6K pathway. These results indicate potential value of rapamycin in the treatment of pulmonary fibrosis.
Figures and Tables
Fig. 1
(A) Characterization of primary human lung fibroblasts. All primary human lung fibroblasts cells displayed typical spindle-shaped morphology under a light microscopy (×40). (B) Characterization of primary human lung fibroblasts. Indirect immunofluorescence staining of primary human pulmonary fibroblasts for vimentin, fibronectin, type III collagen, von Willebrand factor (vWF), pro-surfactant protein C (pro-SPC) and α-smooth muscle actin (α-SMA). Original magnification was ×100. (C) Characterization of primary human lung fibroblasts. Indirect immunofluorescence staining of pulmonary artery endothelial cells for vWF, alveolar type II cells for pro-SPC, and pulmonary artery smooth muscle cells for α-SMA. Original magnification was ×100.
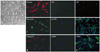
Fig. 2
Assessment of cell viability by MTT. Primary human lung fibroblasts were treated with TGF-β1 (10 ng/mL) and various concentrations of rapamycin (0.01, 0.1, 1.0 and 10 ng/mL). No difference was found in viability between cells treated with rapamycin and TGF-β1 for 24 h and untreated cells (p>0.05). There was also no differernce between TGF-β1 group and the untreated group (p>0.05). Data are representatives of 3 independent experiments. TGF-β1, transforming growth factor β1.
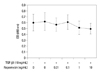
Fig. 3
(A) Rapamycin inhibits TGF-β1-induced type III collagen level in the culture medium of human lung fibroblast, evidenced by ELISA. Type III collagen level after 24-h incubation with medium alone (control), 0.01, 0.1, 1.0, and 10 ng/mL rapamycin and 10 ng/mL TGF-β1. No significant difference was found between different concentration groups of rapamycin. (B) Rapamycin inhibits TGF-β1-induced fibronectin level in the culture medium of human lung fibroblast, shown by ELISA. Fibronectin level after 24-h incubation with medium alone (control), 0.01, 0.1, 1.0, and 10 ng/mL rapamycin and 10 ng/mL TGF-β1. Values are mean of three independent experiments. No significant difference was found between different concentration groups of rapamycin. *p<0.05 vs. control; †p<0.05 vs. TGF-β1 group. TGF-β1, transforming growth factor β1.
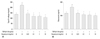
Fig. 4
(A) Effects of rapamycin on TGF-β1-induced gene expression of type III collagen in human lung fibroblasts, shown by real-time PCR. Type III collagen mRNA expression after 24-h incubation with medium alone (control), 0.01, 0.1, 1.0, and 10 ng/mL rapamycin and 10 ng/mL TGF-β1. (B) Effects of rapamycin on TGF-β1-induced gene expression of fibronectin in human lung fibroblasts, shown by real-time PCR. Fibronectin mRNA expression after 24-h incubation with medium alone (control), 0.01, 0.1, 1.0, and 10 ng/mL rapamycin and 10 ng/mL TGF-β1. Values are mean of three independent experiments. *p<0.05 vs. control; †p<0.05 vs. TGF-β1 group; ‡p<0.05 vs. 0.01 ng/mL rapamycin group. TGF-β1, transforming growth factor β1.
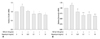
Fig. 5
(A) Effects of rapamycin on TGF-β1-induced mTOR-Ser2448 phosphorylation in human lung fibroblasts, shown by Western blotting. mTOR-Ser2448 phosphorylation after 24-h incubation with medium alone (control), 0.01, 0.1, 1.0, and 10 ng/mL rapamycin and 10 ng/mL TGF-β1. Differences are apparent between the different rapamycin concentration groups (p<0.05). The densities of protein bands were normalized against β-actin and expressed as a ratio. Values are expressed as mean±SEM of three independent experiments. (B) Effects of rapamycin on TGF-β1-induced p70S6K-Thr389 protein production in human lung fibroblasts, examined by Western blotting. p70S6K-Thr389 protein production after 24-h incubation with medium alone (control), 0.01, 0.1, 1.0, and 10 ng/mL rapamycin and 10 ng/mL TGF-β1. The densities of the protein bands were normalized against β-actin and expressed as a ratio. Values are expressed as mean±SEM of three independent experiments. *p<0.05 vs. control; †p<0.05 vs. TGF-β1 group; ‡p<0.05 between two groups. TGF-β1, transforming growth factor β1; mTOR, mammalian target of rapamycin.
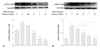
ACKNOWLEDGEMENTS
This work was supported by grants from National Natural Science Foundation of China (No. 30971312) and Specialized Research Fund for the Doctoral Program of Higher Education of China (No. 200800250002).
The authors thank Dr. Bin You and Dr. Qi-Rui Chen for harvesting lung tissue sample, and Xin Chen and Ran Miao for technical assistance.
References
1. Gharaee-Kermani M, Gyetko MR, Hu B, Phan SH. New insights into the pathogenesis and treatment of idiopathic pulmonary fibrosis: a potential role for stem cells in the lung parenchyma and implications for therapy. Pharm Res. 2007. 24:819–841.


2. Andersson-Sjöland A, de Alba CG, Nihlberg K, Becerril C, Ramírez R, Pardo A, et al. Fibrocytes are a potential source of lung fibroblasts in idiopathic pulmonary fibrosis. Int J Biochem Cell Biol. 2008. 40:2129–2140.


3. Phillips RJ, Burdick MD, Hong K, Lutz MA, Murray LA, Xue YY, et al. Circulating fibrocytes traffic to the lungs in response to CXCL12 and mediate fibrosis. J Clin Invest. 2004. 114:438–446.


4. Scotton CJ, Chambers RC. Molecular targets in pulmonary fibrosis: the myofibroblast in focus. Chest. 2007. 132:1311–1321.
5. Willis BC, duBois RM, Borok Z. Epithelial origin of myofibroblasts during fibrosis in the lung. Proc Am Thorac Soc. 2006. 3:377–382.


6. Webster AC, Lee VW, Chapman JR, Craig JC. Target of rapamycin inhibitors (sirolimus and everolimus) for primary immunosuppression of kidney transplant recipients: a systematic review and meta-analysis of randomized trials. Transplantation. 2006. 81:1234–1248.


7. Moses JW, Leon MB, Popma JJ, Fitzgerald PJ, Holmes DR, O'Shaughnessy C, et al. Sirolimus-eluting stents versus standard stents in patients with stenosis in a native coronary artery. N Engl J Med. 2003. 349:1315–1323.


8. Price KA, Azzoli CG, Krug LM, Pietanza MC, Rizvi NA, Pao W, et al. Phase II trial of gefitinib and everolimus in advanced non-small cell lung cancer. J Thorac Oncol. 2010. 5:1623–1629.


9. Simler NR, Howell DC, Marshall RP, Goldsack NR, Hasleton PS, Laurent GJ, et al. The rapamycin analogue SDZ RAD attenuates bleomycin-induced pulmonary fibrosis in rats. Eur Respir J. 2002. 19:1124–1127.


10. Bridle KR, Popa C, Morgan ML, Sobbe AL, Clouston AD, Fletcher LM, et al. Rapamycin inhibits hepatic fibrosis in rats by attenuating multiple profibrogenic pathways. Liver Transpl. 2009. 15:1315–1324.


11. Korfhagen TR, Le Cras TD, Davidson CR, Schmidt SM, Ikegami M, Whitsett JA, et al. Rapamycin prevents transforming growth factor-alpha-induced pulmonary fibrosis. Am J Respir Cell Mol Biol. 2009. 41:562–572.


12. Swaminathan S, Arbiser JL, Hiatt KM, High W, Abul-Ezz S, Horn TD, et al. Rapid improvement of nephrogenic systemic fibrosis with rapamycin therapy: possible role of phospho-70-ribosomal-S6 kinase. J Am Acad Dermatol. 2010. 62:343–345.


13. Poulalhon N, Farge D, Roos N, Tacheau C, Neuzillet C, Michel L, et al. Modulation of collagen and MMP-1 gene expression in fibroblasts by the immunosuppressive drug rapamycin. A direct role as an antifibrotic agent? J Biol Chem. 2006. 281:33045–33052.


14. Sime PJ, Xing Z, Graham FL, Csaky KG, Gauldie J. Adenovector-mediated gene transfer of active transforming growth factor-beta1 induces prolonged severe fibrosis in rat lung. J Clin Invest. 1997. 100:768–776.


15. Bartram U, Speer CP. The role of transforming growth factor beta in lung development and disease. Chest. 2004. 125:754–765.


16. Goodwin A, Jenkins G. Role of integrin-mediated TGFbeta activation in the pathogenesis of pulmonary fibrosis. Biochem Soc Trans. 2009. 37(Pt 4):849–854.


17. Khalil N, O'Connor RN, Unruh HW, Warren PW, Flanders KC, Kemp A, et al. Increased production and immunohistochemical localization of transforming growth factor-beta in idiopathic pulmonary fibrosis. Am J Respir Cell Mol Biol. 1991. 5:155–162.


18. Broekelmann TJ, Limper AH, Colby TV, McDonald JA. Transforming growth factor beta 1 is present at sites of extracellular matrix gene expression in human pulmonary fibrosis. Proc Natl Acad Sci U S A. 1991. 88:6642–6646.


19. Coker RK, Laurent GJ, Shahzeidi S, Lympany PA, du Bois RM, Jeffery PK, et al. Transforming growth factors-beta 1, -beta 2, and -beta 3 stimulate fibroblast procollagen production in vitro but are differentially expressed during bleomycin-induced lung fibrosis. Am J Pathol. 1997. 150:981–991.
20. Coker RK, Laurent GJ, Jeffery PK, du Bois RM, Black CM, McAnulty RJ. Localisation of transforming growth factor beta1 and beta3 mRNA transcripts in normal and fibrotic human lung. Thorax. 2001. 56:549–556.


21. Willis BC, Liebler JM, Luby-Phelps K, Nicholson AG, Crandall ED, du Bois RM, et al. Induction of epithelial-mesenchymal transition in alveolar epithelial cells by transforming growth factor-beta1: potential role in idiopathic pulmonary fibrosis. Am J Pathol. 2005. 166:1321–1332.


22. Willis BC, Borok Z. TGF-beta-induced EMT: mechanisms and implications for fibrotic lung disease. Am J Physiol Lung Cell Mol Physiol. 2007. 293:L525–L534.
23. Yoshioka S, Mukae H, Ishii H, Kakugawa T, Ishimoto H, Sakamoto N, et al. Alpha-defensin enhances expression of HSP47 and collagen-1 in human lung fibroblasts. Life Sci. 2007. 80:1839–1845.


24. Derynck R, Zhang YE. Smad-dependent and Smad-independent pathways in TGF-beta family signalling. Nature. 2003. 425:577–584.


25. Moustakas A, Heldin CH. Non-Smad TGF-beta signals. J Cell Sci. 2005. 118(Pt 16):3573–3584.
26. Lamouille S, Derynck R. Emergence of the phosphoinositide 3-kinase-Akt-mammalian target of rapamycin axis in transforming growth factor-β-induced epithelial-mesenchymal transition. Cells Tissues Organs. 2011. 193:8–22.


27. Hartford CM, Ratain MJ. Rapamycin: something old, something new, sometimes borrowed and now renewed. Clin Pharmacol Ther. 2007. 82:381–388.


28. Pfaffl MW. A new mathematical model for relative quantification in real-time RT-PCR. Nucleic Acids Res. 2001. 29:e45.


29. Eickelberg O, Köhler E, Reichenberger F, Bertschin S, Woodtli T, Erne P, et al. Extracellular matrix deposition by primary human lung fibroblasts in response to TGF-beta1 and TGF-beta3. Am J Physiol. 1999. 276(5 Pt 1):L814–L824.
30. Lock HR, Sacks SH, Robson MG. Rapamycin at subimmunosuppressive levels inhibits mesangial cell proliferation and extracellular matrix production. Am J Physiol Renal Physiol. 2007. 292:F76–F81.


31. Verrecchia F, Mauviel A. Control of connective tissue gene expression by TGF beta: role of Smad proteins in fibrosis. Curr Rheumatol Rep. 2002. 4:143–149.


32. Verrecchia F, Mauviel A. Transforming growth factor-beta signaling through the Smad pathway: role in extracellular matrix gene expression and regulation. J Invest Dermatol. 2002. 118:211–215.


33. Mariani TJ, Roby JD, Mecham RP, Parks WC, Crouch E, Pierce RA. Localization of type I procollagen gene expression in silica-induced granulomatous lung disease and implication of transforming growth factor-beta as a mediator of fibrosis. Am J Pathol. 1996. 148:151–164.