Abstract
Purpose
This study was performed to evaluate the long-term effects and safety of intratracheal (IT) transplantation of human umbilical cord blood-derived mesenchymal stem cells (hUCB-MSCs) in neonatal hyperoxic lung injury at postnatal day (P)70 in a rat model.
Materials and Methods
Newborn Sprague Dawley rat pups were subjected to 14 days of hyperoxia (90% oxygen) within 10 hours after birth and allowed to recover at room air until sacrificed at P70. In the transplantation groups, hUCB-MSCs (5×105) were administered intratracheally at P5. At P70, various organs including the heart, lung, liver, and spleen were histologically examined, and the harvested lungs were assessed for morphometric analyses of alveolarization. ED-1, von Willebrand factor, and human-specific nuclear mitotic apparatus protein (NuMA) staining in the lungs and the hematologic profile of blood were evaluated.
Results
Impaired alveolar and vascular growth, which evidenced by an increased mean linear intercept and decreased amount of von Willebrand factor, respectively, and the hyperoxia-induced inflammatory responses, as evidenced by inflammatory foci and ED-1 positive alveolar macrophages, were attenuated in the P70 rat lungs by IT transplantation of hUCB-MSCs. Although rare, donor cells with human specific NuMA staining were persistently present in the P70 rat lungs. There were no gross or microscopic abnormal findings in the heart, liver, or spleen, related to the MSCs transplantation.
Bronchopulmonary dysplasia (BPD), a chronic lung disease that usually occurs in premature infants receiving prolonged ventilator support and oxygen supplementation, remains a major cause of mortality and long-term respiratory morbidity among premature infants despite recent improvements in neonatal intensive care medicine.1,2 As the treatment options for this common and serious disorder are limited, the development of a new therapeutic modality to improve the prognosis of this disease remains a major challenge in neonatal medicine.3,4
Recent advances in stem cell research show promising possibility for the prevention and treatment of incurable diseases, including BPD. Recently, we have shown that xeno transplantation of human umbilical cord blood (UCB)-derived mesenchymal stem cells (MSCs) attenuates hyperoxia-induced lung injuries, such as impaired alveolarization, inflammatory responses, oxidative stress, increased apoptosis and fibrosis, inimmuno competent neonatal rats.5,6 Furthermore, these protective effects were dose-dependent,5 and local intratracheal administration rather than systemic intraperitoneal transplantation was the preferred route of stem cell transplantation.6 Taken together, these findings suggest that human UCB-derived MSCs transplantation could be a novel therapeutic modality for the treatment of BPD. However, whether the protective effects of stem cell transplantation observed in the newborn period persist into adulthood is not known. Moreover, the long-term safety of human UCB-derived MSCs transplantation, which is essential for clinical translation, has not been determined yet. Therefore, in this pre-clinical translational study, we attempted to determine the long-term [postnatal day (P)70]outcome and safety of intratracheally delivered human UCB-derived MSCs in neonatal hyperoxic lung injury.
Newborn Sprague-Dawley rat pups were exposed to hyperoxia (90% oxygen) within 10 hours of birth until P14 and were allowed to recover in room air until sacrificed at P70. Mean linear intercept (MLI) was assessed for lung alveolarization, and angiogenesis was measured based on the expression of von Willebrand factor (vWF). Alveolar macrophages were measured by ED-1 quantification. The presence of transplanted human UCB-derived MSCs in the rat lung was detected by human specific nuclear mitotic apparatus protein (NuMA) expression. Hematologic analyses, including white blood cell (WBC) and lymphocyte counts, and subset analysis were also evaluated.
This study was approved by the Institutional Review Board of Samsung Medical Center and by Medipost, Co., Ltd., Seoul, Korea. UCB was collected from umbilical veins after neonatal delivery with informed consent from pregnant mothers. As previously reported, mesenchymal stem cells were isolated from human UCB, and cultivated.5,6 The cells expressed CD105 (99.6%) and CD73 (96.3%), but not CD34 (0.1%), CD45 (0.2%) or CD14 (0.1%), and they were positive for human leukocyte antigen (HLA)-AB (96.8%) but not HLA-DR (0.1%).5 They expressed pluripotency markers, such as octamer bindings transcription factor 4 (30.5%)7 and stage specific embryonic antigen 4 (67.7%).8 Human UCB-derived MSCs differentiated into various cell types, including respiratory epithelium, osteoblasts, chondrocytes and adipocytes, in response to specific induction stimuli.5,6 We have confirmed the differentiation potential and karyotypic stability of the human UCB-derived MSCs up to the 11th passage.5,6
The Animal Care and Use Committee of Samsung Biomedical Research Institute, Seoul, Korea reviewed and approved the described experimental protocol. This study was also done in accordance with the Institutional and National Institutes of Health guidelines for laboratory animal care. Timed pregnant Sprague Dawley rats (Orient Co., Seoul, Korea) were housed in individual cages with free access to water and laboratory chow. Rat pups were delivered spontaneously and reared with their dams. Newborn rat pups were randomly allocated to three groups: normoxia control group (NC, n=10), hyperoxia control group (HC, n=11), and hyperoxia with human UCB-derived MSCs transplantation group (HM, n=12). Rat pups from the NC were initially kept with a nursing mother rat, and after weaning, raised separately in a standard cage kept in room air throughout the experiment. Pups from the hyperoxia groups were maintained with a nursing mother in standard cages in 50 liter Plexiglas chambers, in which the hyperoxic condition (oxygen concentration of 90%) was maintained. Hyperoxia started within 10 hours after birth and continued up to P14. Following this treatment, the rat pups were kept in room air until they were sacrificed at P70. Humidity and environmental temperature was maintained at 50% and 24℃, respectively. Nursing mother rats were rotated daily between litters in the normoxia and hyperoxia groups until P14, to avoid oxygen toxicity. Survival and body weights of rat pups in each group were checked daily until P14, and then checked weekly from P21 to P70. Rats were sacrificed under deep pentobarbital anesthesia (60 mg/kg, intraperitoneal), blood was drawn from cardiac puncture, and organs, including the brain, heart, lung, liver, spleen, were obtained for weighing and histological analyses.
Human UCB-derived MSCs from the 5th passage from a single donor were transplanted at P5. For cell transplantation, 5×105 cells in 0.05 mL phosphate buffered saline (PBS, pH 7.4) were injected intratracheally with a 30-gauge needle syringe. For NC and HC, an equal volume of PBS was administrated intratracheally.5,6 There was no mortality associated with the transplantation procedure.
Organs, including the brain, heart, lung, liver and spleen, were harvested after transcardiac perfusion with ice-cold PBS. Harvested organs were grossly inspected, weighed, and fixed overnight in 10% buffered formalin at room temperature. For morphometric analysis, lungs were fixed in situ by tracheal instillation of 10% buffered formalin at a constant pressure of 20 cm H2O, and then fixed overnight at room temperature in the same fixative. After tissue processing, fixed organs were embedded in paraffin wax. Four micrometer thick sections were sliced from the paraffin blocks and stained with hematoxylin and eosin. Images of each section were captured with a magnifier digital camera through an Olympus BX40 microscope (Olympus optical Co. Ltd., Tokyo, Japan) and were saved as JPEG files.
The level of alveolarization was assessed by measuring mean linear index (MLI). MLI was defined as the value of the total length of lines drawn across lung section divided by the number of alveoli intercepts encountered, as described by Cooney and Thurlbeck.9 The inflammatory foci of the lung was defined as small pathologic legion which was evidenced by the infiltration of alveolar macrophages, red blood cells, and neutrophils and the thickening of the alveolar wall. Inflammatory foci were scored as normal (no change), minimal (one or two foci recognized under a ×2.5 objective), slight (intermediate between minimal and moderate), or moderate (more than 5, multiple, large inflammatory foci, recognized under a ×2.5 objective) based on modification of previously suggested methods.10-12 The severity of inflammatory foci was operationally defined as follows: normal=0, minimal=1, slight=2, and moderate=3. A minimum of four sections per rat and six fields per section were randomly examined by a pulmonary pathology specialist in a blinded manner.
Immunofluoroscence for angiogenesis (vWF), alveolar macrophages (ED-1) and donor cell localization (human-specific NuMA) was performed on the de-paraffinized 5 µm thick lung sections. To detect antigens, specimens were placed in a solution containing 0.1% (v/v) Triton X-100 and 0.5% (v/v) bovine serum albumin in PBS. The following primary antibody were incubated overnight at 4℃ with 1 : 100 for monocyte/macrophages (CD68, ED-1, mouse monoclonal, hemiproteincon, Millipore, MA, USA), 1 : 50 for NuMA (MERCK, Darmstadt, Germany), and 1 : 200 for vWF (Dako, Glostrup, Denmark) for angiogenesis. Dako polyclonal rabbit anti-mouse immunoglobulins/fluorescein isothiocyanate (FITC) (1 : 200, DakoUk Ltd., Cambridgeshire, UK) and polyclonal swine anti-rabbit immunoglobulins/FITC (1 : 200, DakoUk Ltd., Cambridgeshire, UK) were exposed for 2 hr at room temperature. Vectasheld mount medium with 4', 6-diamidino-2-phenylindole (Vector Laboratories, Inc., Burlingame, CA, USA) was used to preserve staining. Confocal microscopy was carried out at 400X or 800X magnification using Bio-Rad Radiance 2100 (Bio-Rad Laboratories, Inc., Hercules, CA, USA) with krypton/argon laser, and images were achieved using the Laser shop 2000 software (Bio-Rad Laboratories, Inc., Hercules, CA, USA). A minimum of three sections per rat and ten fields per each section were randomly selected and the optical density of immunofluroroscence was measured using the Image J (National Institutes of Health, USA) to assess extent of vWF, and ED-1 positive cells were counted to evaluate alveolar macrophage infiltration.
Withdrawn blood was analyzed for a total WBC count and leukocyte differential and absolute count using the ADVIA 120 Hematology System (Bayer Co., Tarrytown, NY, USA). Lymphocytes were analyzed for anti-CD3 (T cell marker), anti-CD4 (Th cell marker), anti-CD8 (Tc cell marker) using FACSort (Beckton-Dickson Co., San Jose, CA, USA).
Data are expressed as the mean±standard error of the mean. For continuous variables with a normal distribution, a statistical comparison between groups was performed by one-way analysis of variance test with Bonferroni's correction. For variables without a normal distribution, Wiloxon signed-rank tests with Bonferroni's correction were performed. A p-value of <0.05 was considered significant. Stata software (ver. 11.0, Stata Corp LP, College Station, TX, USA) was used for all analyses.
The survival rate during the first 2 weeks of hyperoxic exposure in HC and HM was 82% (9/11) and 83% (10/12), respectively, and no mortality was reported during the recovery period until sacrifice at P70. There was no mortality in the NC throughout the experiment. Birth weight was not different between the experimental groups. Although body weight in HC at P8 and P11 was significantly lower than NC, the final (P70) body weight (377.8±23.0 g, 373.8±21.7 g and 388.8±26.3 g, for the HC, NC and HM, respectively) was not different between groups. In addition, weight of various organs (brain/heart/lung/liver/spleen) including the lung (1.42+0.09 g, 1.45+0.11 g, and 1.55+0.15 g in NC, HC, and HM, respectively) at P70, were not significantly different between the experimental groups.
Fig. 1A presents typical photomicrographs showing histopathological differences of the P70 rat lung in each experimental group. Impaired alveolar growth, as evidenced by fewer and larger alveoli with heterogeneous sizes, was observed in HC as compared to NC, and these impairments in alveolar growth and morphological changes observed in HC were attenuated in HM. Based on morphometric analysis, the MLI, which is indicative of the alveolar size, was significantly higher in HC as compared to NC (p-value <0.05), and this hyperoxia-induced abnormality in morphometry was significantly attenuated in HM (p-value <0.01) (Fig. 1B). There were no grossly or histologically abnormal findings related to hyperoxia or stem cell transplantation, such as hypertrophy, tumor, hemorrhage, hematoma and cancerous pathologic findings in the organs, including the brain, heart, lung, liver and spleen.
Density of immunofluorescence staining for vWF, which indicate the extent of angiogenesis, were significantly reduced in HC as compared to NC in the P70 rat lung (p-value <0.05) (Fig. 2A and B). This hyperoxia-induced decrease in angiogenesis was significantly attenuated in HM (p-value <0.05).
The number of ED-1 positive cells, indicating alveolar macrophages in the lung of P70 rats per high-power field (Fig. 3A), was significantly higher in HC (p-value <0.05) only, not in HM (p-value >0.05), as compared to NC (Fig. 3B). The inflammatory foci in the lungs was graded as normal, minimal, slight and moderate in order to the severity (Fig. 3C). The average severity score of inflammation foci is significantly higher only in HC (p-value <0.05), not in HM, as compared to NC (Fig. 3D).
Donor stem cells were localized with NuMA, a human RNA-specific protein. Although they occur rarely, the expression of NuMA indicating the presence of human UCB-derived MSCs was observed only in HM of the P70 rat lung (Fig. 4).
Impaired alveolarization and vascular growth had adverse pulmonary consequences, as indicated by abnormal pulmonary function tests in premature infants suffered from BPD, and these can extend into and beyond childhood.13 Previous studies have reported that newborn rat pups exposed to hyperoxia showed markedly and persistently inhibited distal lung growth and had an increased distal air space size, which closely mimic the histology observed in human infants with new BPD.14,15 Furthermore, we have demonstrated that exposure of newborn rat pups to 90-95% hyperoxia for 2 weeks resulted in increased mortality, delayed growth, and lung injuries similar to those seen in premature human infants with BPD.5,6
In previous studies, we demonstrated that local intratracheal administration rather than systemic intraperitoneal transplantation was a more optimal route, when the MSCs were given at P5,6 and this short-term protective effects of UCB-MSCs were dose-dependent.5 As follow up-preclinical translational study, same optimal dose, timing and route for MSCs transplantation were chosen in the present study to further evaluate the long-term effects and safety of MSC transplantation in the same experimental settings with the previous studies.5,6
A recent study reported long-term (P65) failure of alveologenesis after an early short-term (P1-7) exposure to a PDGF-receptor antagonist.16 This implies that an insult in the early critical period can result in long-lasting changes in lung architecture, and suggests that permanent inhibition of alveolar formation could occur in BPD infants. These previous data are consistent well with the findings from the present study. In the present study, although somatic catch-up growth was observed during the recovery period, the alveolar and vascular growth measured by MLI and vWF, respectively, were still delayed in HC compared to NC, and these hyperoxia-induced lung injuries were attenuated in HM at P70. These findings suggest that hyperoxia-induced lung injuries persist even after a prolonged recovery period, and that the protective effects of human UCB-derived MSCs transplantation, as evidenced by improved alveolarization and angiogenesis, also are sustained up to P70.
Pulmonary inflammatory responses have been known to play a pivotal role in the development of BPD.1,14,17-19 In our previous studies,5,6 we have shown that the protective effects of human UCB-derived MSCs transplantation against hyperoxia-induced lung injuries are mediated mainly by their anti-inflammatory effects and not by their regeneration capacity.
In the current study, we found that the pulmonary inflammatory foci and ED-1 positive alveolar macrophages were persistently increased at P70 in HC than NC. The augmented number of alveolar macrophages, observed mainly in the inflammatory foci, was related to an increase in the inflammatory foci score. In a previous study,20 increased lymphocyte infiltration, a type of inflammation, was also observed in the chronic phase of BPD in the rabbit lungs, which is consistent with our data. These hyperoxia-induced inflammatory responses appeared to be attenuated in HM at P70. These findings suggest that hyperoxia-induced inflammatory responses persist even after a prolonged recovery period, and that the anti-inflammatory effects of stem cell transplantation are sustained up to P70.
It has been shown that MSCs do not elicit alloreactive lymphocyte proliferative responses,21 and the implantation of allogeneic, major-histocompatibility-mismatched MSCs have been well tolerated in baboons.22,23 This low immunogenic property of MSCs is thought to be related with the secretion of various factors that create an immunosuppressive environment.24,25 MSCs can be obtained from various sources, including bone marrow, UCB and adipose tissue.26 Among these sources, UCB is considered a promising source for human MSCs due to the low immunogenicity,27 easy availability and high proliferation capacity.28 The low expression of HLA major histocompatibility complex (MHC) class I and lack of MHC class II molecules in UCB-derived MSCs, indicates that these MSCs may evade the immune system, even in allogeneic transplantation.29 In our previous5,6 and present studies, despite xenotransplantation to the immunocompetent wild type rat, we found no apparent gross or microscopic findings consistent with abnormal immunologic reactions after local intratracheal transplantation of hUCB-MSCs. Furthermore, a hyperoxia-induced increase in WBC count (mainly neutrophil) in the blood was not significantly noticed in HM and the lymphocyte counts and its subset were not different between the experimental groups in the present study. However, further detailed studies are needed to determine whether these results are derived from the known low-immnunogenecity of MSCs or unknown poor immune responses in newborn rats.
We have previously shown the presence of human UCB-derived MSCs which were transplanted to the wild type rat pups at P14.5,6 In the present study, although they were rare, donor stem cells were persistently present in the P70 rat lungs. However, whether the donor cells observed in the lungs held stem cell properties is not clear; further investigation is needed.
The long-term safety of MSCs transplantation is not yet known. In the present study, no gross or histological abnormal findings, such as tumors, were observed in various organs in P70 rats. Rats raised up to P70 could be considered to be as old as human adults. Furthermore, the measured proportional weight (organ/body weight) of organs was not different between the NC, HC, and HM groups. These findings suggest that IT transplantation of human UCB-derived MSCs is safe without any long-term adverse effects up to P70.
Several clinical studies have demonstrated that the incidence of wheezing or asthma, or of bronchial hyper-responsiveness, is higher in BPD survivors30 and that persistent detrimental pulmonary function is correlated with the severity of BPD at diagnosis.31 Our present study demonstrated chronic ongoing lung impairment after an initial hyperoxic insult, which could be considered as supporting evidence for long-term respiratory morbidity of BPD infants. Furthermore, transplantation of UCB-derived MSCs in the acute critical period attenuated hyperoxic lung injury at P14, and this seemed to contribute to the long-term improvement evaluated at P70. These data support the possibility that transplantation of human UCB-derived MSCs may modify the long-term respiratory morbidities, if BPD infants are treated at an early critical time point.
In summary, the hyperoxia-induced retardation in alveolar and vascular growth and inflammatory responses in newborn rats were still persistent after a prolonged recovery period. The protective effects of intratracheal transplantation of human UCB-derived MSCs against neonatal hyperoxic lung injuries, such as improved alveolarization with angiogenesis and anti-inflammatory effects, were also sustained without any long-term adverse effects. Our data warrant the future translation of the transplantation of human UCB-derived MSCs into clinical studies for the treatment of BPD.
Figures and Tables
Fig. 1
Histology and morphometric analysis of the surviving P70 rat lung. (A) Representative optical microscopic photographs of the lungs stained with hematoxylin and eosin (original magnification; ×100, scale bars; 50 um). (B) Degree of alveolarization measured by mean linear intercept. Data are expressed as mean±SEM; *p<0.05 versus NC; †p<0.05 versus HC. NC, normoxia control group; HC, hyperoxia control group; HM, hyperoxia with human UCB-derived MSCs transplantation group; MSCs, mesenchymal stem cells; SEM, standard error of the mean.
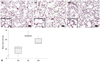
Fig. 2
(A) Representative immunofluorescence photomicrographs of von Willebrand factor (vWF) staining in the lungs of P70 rats. vWF was labeled with green fluorescent marker 5(6)-carboxyfluoresceindiacetate N-succinimidyl ester and the nuclei were labeled with 4',6-diamidino-2-phenylindole (blue) (scale bars; 25 um). (B) The immunofluorescence density of vWF per lung section. Data are expressed as mean±SEM; *p<0.05 versus NC; †p<0.05 versus HC. NC, normoxia control group; HC, hyperoxia control group; HM, hyperoxia with human UCB-derived MSCs transplantation group; MSCs, mesenchymal stem cells; SEM, standard error of the mean.
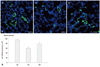
Fig. 3
(A) Representative photomicrographs of ED-1 positive cells in the lungs of P70 rats. ED-1 positive alveolar macrophages were labeled with CFSE (green), and the nuclei were labeled with DAPI (blue) (scale bar; 25 um). (B) Number of ED-1 positive cells per high power field in P70 rat lungs. (C) Representative optical microscopic photographs of the inflammatory foci grade in the P70 rat lungs stained with hematoxylin and eosin (scale bar; 100 um). (D) Severity scores of the inflammatory focis in the in P70 rat lungs. Data are expressed as mean±SEM; *p<0.05 versus NC. NC, normoxia control group; HC, hyperoxia control group; HM, hyperoxia with human UCB-derived MSCs transplantation group; CFSE, 5(6)-carboxyfluoresceindiacetate N-succinimidyl ester; DAPI, 4',6-diamidino-2-phenylindole; MSCs, mesenchymal stem cells; HPF, high power field; SEM, standard error of the mean.
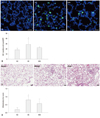
Fig. 4
Donor cell localization in the lung of the P70 rats. Human specific nuclear mitotic apparatus protein (NuMA) positive human UCB-derived MSCs were labeled with CFSE (green), and the nuclei were labeled with DAPI (blue) (scale bars; 25 um). UCB, umbilical cord blood; MSCs, mesenchymal stem cells; CFSE, 5(6)-carboxyfluoresceindiacetate N-succinimidyl ester; DAPI, 4',6-diamidino-2-phenylindole.
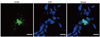
Fig. 5
The number of white blood cells (WBC) (A), neutrophil (B), monocyte (C), and the number of lymphocyte with T cell (CD4 positive and CD8 positive T cell) (D) in each experimental group at P70. Although WBC counts were significantly increased in HC compared to NC, there were no significant differences in lymphocyte counts and subset between the experimental groups. Data are expressed as mean±SEM; *p<0.05 versus NC. NC, normoxia control group; HC, hyperoxia control group; HM, hyperoxia with human UCB-derived MSCs transplantation group; MSCs, mesenchymal stem cells; SEM, standard error of the mean.
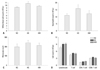
ACKNOWLEDGEMENTS
This work was supported by Basic Science Research Program through the National Research Foundation of Korea (NRF) funded by the Ministry of Education, Science and Technology (S-2011-0317-000), and by a Samsung Biomedical Research Institute Grant (#SBRI CB11271).
References
1. Bhandari A, Panitch HB. Pulmonary outcomes in bronchopulmonary dysplasia. Semin Perinatol. 2006. 30:219–226.


2. Bland RD. Neonatal chronic lung disease in the post-surfactant era. Biol Neonate. 2005. 88:181–191.


3. Avery ME, Tooley WH, Keller JB, Hurd SS, Bryan MH, Cotton RB, et al. Is chronic lung disease in low birth weight infants preventable? A survey of eight centers. Pediatrics. 1987. 79:26–30.


4. Bregman J, Farrell EE. Neurodevelopmental outcome in infants with bronchopulmonary dysplasia. Clin Perinatol. 1992. 19:673–694.


5. Chang YS, Choi SJ, Sung DK, Kim SY, Oh W, Yang YS, et al. Intratracheal transplantation of human umbilical cord blood-derived mesenchymal stem cells dose-dependently attenuates hyperoxia-induced lung injury in neonatal rats. Cell Transplant. 2011. 20:1843–1854.


6. Chang YS, Oh W, Choi SJ, Sung DK, Kim SY, Choi EY, et al. Human umbilical cord blood-derived mesenchymal stem cells attenuate hyperoxia-induced lung injury in neonatal rats. Cell Transplant. 2009. 18:869–886.


7. Boiani M, Schöler HR. Regulatory networks in embryo-derived pluripotent stem cells. Nat Rev Mol Cell Biol. 2005. 6:872–884.


8. Gang EJ, Bosnakovski D, Figueiredo CA, Visser JW, Perlingeiro RC. SSEA-4 identifies mesenchymal stem cells from bone marrow. Blood. 2007. 109:1743–1751.


9. Cooney TP, Thurlbeck WM. The radial alveolar count method of Emery and Mithal: a reappraisal 2--intrauterine and early postnatal lung growth. Thorax. 1982. 37:580–583.


10. Takada H, Kishimoto C, Hiraoka Y. Therapy with immunoglobulin suppresses myocarditis in a murine coxsackievirus B3 model. Antiviral and anti-inflammatory effects. Circulation. 1995. 92:1604–1611.


11. Smith S, Liggitt D, Jeromsky E, Tan X, Skerrett SJ, Wilson CB. Local role for tumor necrosis factor alpha in the pulmonary inflammatory response to Mycobacterium tuberculosis infection. Infect Immun. 2002. 70:2082–2089.


12. Skerrett SJ, Liggitt HD, Hajjar AM, Ernst RK, Miller SI, Wilson CB. Respiratory epithelial cells regulate lung inflammation in response to inhaled endotoxin. Am J Physiol Lung Cell Mol Physiol. 2004. 287:L143–L152.


13. Baraldi E, Filippone M, Trevisanuto D, Zanardo V, Zacchello F. Pulmonary function until two years of life in infants with bronchopulmonary dysplasia. Am J Respir Crit Care Med. 1997. 155:149–155.


14. Warner BB, Stuart LA, Papes RA, Wispé JR. Functional and pathological effects of prolonged hyperoxia in neonatal mice. Am J Physiol. 1998. 275(1 Pt 1):L110–L117.
15. Kunig AM, Balasubramaniam V, Markham NE, Morgan D, Montgomery G, Grover TR, et al. Recombinant human VEGF treatment enhances alveolarization after hyperoxic lung injury in neonatal rats. Am J Physiol Lung Cell Mol Physiol. 2005. 289:L529–L535.


16. Lau M, Masood A, Yi M, Belcastro R, Li J, Tanswell AK. Long-term failure of alveologenesis after an early short-term exposure to a PDGF-receptor antagonist. Am J Physiol Lung Cell Mol Physiol. 2011. 300:L534–L547.


17. Choo-Wing R, Nedrelow JH, Homer RJ, Elias JA, Bhandari V. Developmental differences in the responses of IL-6 and IL-13 transgenic mice exposed to hyperoxia. Am J Physiol Lung Cell Mol Physiol. 2007. 293:L142–L150.


18. Lindsay L, Oliver SJ, Freeman SL, Josien R, Krauss A, Kaplan G. Modulation of hyperoxia-induced TNF-alpha expression in the newborn rat lung by thalidomide and dexamethasone. Inflammation. 2000. 24:347–356.
19. Marcho Z, White JE, Higgins PJ, Tsan MF. Tumor necrosis factor enhances endothelial cell susceptibility to oxygen toxicity: role of glutathione. Am J Respir Cell Mol Biol. 1991. 5:556–562.


20. Ryan RM, Ahmed Q, Lakshminrusimha S. Inflammatory mediators in the immunobiology of bronchopulmonary dysplasia. Clin Rev Allergy Immunol. 2008. 34:174–190.


21. Le Blanc K, Tammik C, Rosendahl K, Zetterberg E, Ringdén O. HLA expression and immunologic properties of differentiated and undifferentiated mesenchymal stem cells. Exp Hematol. 2003. 31:890–896.


22. Bartholomew A, Patil S, Mackay A, Nelson M, Buyaner D, Hardy W, et al. Baboon mesenchymal stem cells can be genetically modified to secrete human erythropoietin in vivo. Hum Gene Ther. 2001. 12:1527–1541.


23. Devine SM, Bartholomew AM, Mahmud N, Nelson M, Patil S, Hardy W, et al. Mesenchymal stem cells are capable of homing to the bone marrow of non-human primates following systemic infusion. Exp Hematol. 2001. 29:244–255.


24. Aggarwal S, Pittenger MF. Human mesenchymal stem cells modulate allogeneic immune cell responses. Blood. 2005. 105:1815–1822.


25. Kode JA, Mukherjee S, Joglekar MV, Hardikar AA. Mesenchymal stem cells: immunobiology and role in immunomodulation and tissue regeneration. Cytotherapy. 2009. 11:377–391.


27. Rocha V, Wagner JE Jr, Sobocinski KA, Klein JP, Zhang MJ, Horowitz MM, et al. Graft-versus-host disease in children who have received a cord-blood or bone marrow transplant from an HLA-identical sibling. Eurocord and International Bone Marrow Transplant Registry Working Committee on Alternative Donor and Stem Cell Sources. N Engl J Med. 2000. 342:1846–1854.


28. Yang SE, Ha CW, Jung M, Jin HJ, Lee M, Song H, et al. Mesenchymal stem/progenitor cells developed in cultures from UC blood. Cytotherapy. 2004. 6:476–486.


29. Le Blanc K. Immunomodulatory effects of fetal and adult mesenchymal stem cells. Cytotherapy. 2003. 5:485–489.

