Abstract
Purpose
Lamotrigine, a novel anticonvulsant, is a sodium channel blocker that is efficacious in certain forms of neuropathic pain. Recently, microglial and astrocytic activation has been implicated in the development of nerve injury-induced neuropathic pain. We have assessed the effects of continuous intrathecal administration of lamotrigine on the development of neuropathic pain and glial activation induced by L5/6 spinal-nerve ligation in rats.
Materials and Methods
Following left L5/6 spinal nerve ligation (SNL), Sprague-Dawley male rats were intrathecally administered lamotrigine (24, 72, or 240 µg/day) or saline continuously for 7 days. Mechanical allodynia of the left hind paw to von Frey filament stimuli was determined before surgery (baseline) and once daily for 7 days postoperatively. On day 7, spinal activation of microglia and astrocytes was evaluated immunohistochemically, using antibodies to the microglial marker OX-42 and the astrocyte marker glial fibrillary acidic protein (GFAP).
Results
Spinal-nerve ligation induced mechanical allodynia in saline-treated rats, with OX-42 and GFAP immunoreactivity being significantly increased on the ipsilateral side of the spinal cord. Continuously administered intrathecal lamotrigine (240 µg/day) prevented the development of mechanical allodynia, and lower dose of lamotrigine (72 µg/day) ameliorated allodynia. Intrathecal lamotrigine (72 and 240 µg/day) inhibited nerve ligation-induced microglial and astrocytic activation, as evidenced by reduced numbers of cells positive for OX-42 and GFAP.
Conclusion
Continuously administered intrathecal lamotrigine blocked the development of mechanical allodynia induced by SNL with suppression of microglial and astrocytic activation. Continuous intrathecal administration of lamotrigine may be a promising therapeutic intervention to prevent neuropathy.
Peripheral nerve damage frequently results in neuropathic pain, which manifests primarily as allodynia (pain caused by a stimulus that does not normally provoke pain) and hyperalgesia (an increased response to a stimulus that is normally painful). The mechanisms responsible for the induction of nerve injury-induced neuropathic pain, however, are not entirely clear.
Activation of spinal glial cells (microglia and astrocytes) has been shown to contribute to the development and maintenance of nerve injury-induced neuropathic pain in the spinal nerve ligation (SNL) model in rats.1 Immunohistochemical investigations have demonstrated that, in animal models, spinal glial cells are activated by spinal nerve injury.2 Spinal glial cells may be activated by various pain-transmitting substances and prostaglandins, an activation related to pathologic pain states.3 Indeed, pharmacologic suppression of microglial and astrocytic activation attenuates the development of nerve injury-induced neuropathic pain.3
Lamotrigine was originally designed as an anticonvulsant but found to have analgesic activity, leading to its use in the treatment of different types of neuropathic pain. Its precise mechanism of action has not been determined, but the anticonvulsant activities of lamotrigine are thought to be related to its effects on sodium channels. In vitro pharmacologic studies have suggested that lamotrigine inhibits voltage sensitive sodium channels, stabilizing neuronal membranes and modulating the presynaptic transmitter release of excitatory amino acids, such as glutamate and aspartate.4 Sodium channel blockers have been shown to be effective in the treatment of neuropathic pain,5-7 and intrathecally administered lamotrigine was found to attenuate neuropathic pain.8 However, the effect of intrathecal lamotrigine on glial cell activation has not been determined. We, therefore, tested the possibility that lamotrigine attenuates microglial and astrocytic activation in the rat SNL model.
Sprague-Dawley male rats, weighing 200-250 g, were housed individually in plastic cages with soft bedding at room temperature and maintained on a 12-hour light/12-hour dark cycle, with free access to food and water. All animal experiments were conformed to the guidelines of and were approved by the Animal Use and Care Committee at Asan Institute for Life Science.
All surgical procedures were performed under inhalational anesthesia with sevoflurane in 100% oxygen, induced at 6% and maintained at 3%. Neuropathic pain was induced as described.9 Briefly, rats were anesthetized and placed under a microsurgical apparatus in a prone position. A midline incision was made on the back, and the left paraspinal muscles were separated from the spinous processes at the L4-S2 levels. The left L6 transverse process was carefully removed, and the L4/5 spinal nerves were identified. The left L5 nerve was tightly ligated with a 6-0 silk thread. The left L6 spinal nerve, located just caudal and medial to the sacroiliac junction, was tightly ligated with a silk thread. After suturing the left paraspinal muscles, intrathecal catheter was implanted according to a method used for lumbar catheterization.10 Briefly, a guide cannula (BD Angiocath Plus™, Becton Dickinson, Sandy, UT, USA, 20 ga, 1.1×30 mm) was inserted between the L5 and L6 vertebrae, and sterile saline-filled polyethylene tubing (PE-10, inner diameter 0.28 mm, outer diameter 0.61 mm, Becton Dickinson, Sparks, MD, USA) was inserted into the intrathecal space through the cannula. The internal tip of the polyethylene tubing was located at approximately the L1 level. The external end of the polyethylene tubing was connected to a mini-osmotic pump (Alzet Model 2001, pumping rate 1 µL/hour, fill volume 211 microliter, DURECT Corporation, Cupertino, CA, USA). The pump was inserted into a small subcutaneous pocket created in the posterior sacral area. The skin was closed with 4.0 silk sutures.
For sham-operated rats, the left L5 and L6 spinal nerves were exposed, but not ligated, and the intrathecal polyethylene tubing was not implanted. Animals that showed neurologic deficits after surgery were excluded from the study.
Lamotrigine (molecular weight, 256.09; Sigma, St. Louis, MO, USA) was dissolved in dimethyl sulfoxide (DMSO, minimum 99.5%; Sigma, St. Louis, MO, USA) and diluted with 0.9% saline. The final concentration of DMSO was 2%, 6% and 19%, respectively. The doses selected for intrathecal lamotrigine were based on previous results.8,11
Sixty rats were divided into five groups of 12 each: sham operated, control, LTG24, LTG72, and LTG240. In the control group, normal saline was continuously administered at a rate of 1 µL/hour for 7 days after SNL. In the lamotrigine groups (LTG24, LTG72, and LTG240), the osmotic pumps were filled with 210 µL of lamotrigine, at concentrations of 1, 3, and 10 µg/µL, and the rats were daily administered 24, 72, and 240 µg lamotrigine, respectively, continuously for 7 days after SNL. On day 8, the rats were subsequently sacrificed and their spinal cords were collected for evaluation of microglial and astrocytic activation. The doses of drugs were determined on the basis of our previous study.12 We investigated the antiallodynic effect of intrathecally administered lamotrigine (10, 30, 100, and 300 µg) in a rat model of neuropathic pain (spinal nerve ligation) and a dose-dependent antiallodynic action was observed (ED50=61.7 µg).
To quantify mechanical allodynia, the left hind paw withdrawal threshold (WT) was determined before surgery (baseline) and once daily for 7 days after surgery using von Frey filaments (Stoelting Co., Wood Dale, IL, USA) and the "up and down" method.13 All behavioral tests were performed between 9 AM and 2 PM by an examiner blinded to the treatment groups.
Each rat was placed in a transparent plastic dome with a metal mesh floor, allowing access to the plantar surface of the hind paw, and was habituated for 30 minutes. A von Frey filament was pressed perpendicular to the plantar surface of each left hind paw with sufficient force to cause slight bending, and was held for approximately 6 seconds. A positive response was defined as sharp withdrawal of the left hind paw or as flinching immediately upon removal of the filament. Each trial was repeated 3 times at approximately 3-minute intervals, and the mean force to produce withdrawal responses was determined.
Locomotor functional changes were evaluated by rotarod testing (Acceler Rota-Rod for Rats 7750; Ugo Basile, Italy). Rats were acclimated to revolving drums and habituated to handling to ameliorate stress during testing. Before spinal nerve ligation, rats were subjected to three training trials over 2 days on revolving drums of axis diameter 6.0 cm, with a corrugated surface. Rats were placed on the drum rotating at the lowest possible speed of 4 rpm and the speed was increased at a rate of 0.12 rpm/sec, to a maximum of 40 rpm. Rats able to remain on the revolving drum for a minimum of 120 sec were selected for drug testing. Baseline performance time was calculated as the mean of the three training runs. Following SNL, the rotarod performance of each rat was measured once daily for 7 days. Each test was performed three times at 5 min intervals, and mean values were calculated.
To evaluate microglial and astrocytic activation after surgery, tissue samples were stained with antibodies raised against the microglial marker OX-42 and the astrocyte marker glial fibrillary acidic protein (GFAP). After the last behavioral assessment on day 7 after surgery, rats were deeply anesthetized with sodium pentobarbital (120 mg/kg, intraperitoneal) and perfused intracardially with heparinized normal saline, followed by 4% paraformaldehyde in phosphate buffered saline (PBS). The spinal cord around L5 and L6 was removed and post-fixed in the same fixative at room temperature for 4 hours. All specimens were cryoprotected in 30% (w/v) sucrose. Spinal cords were cut transversely by cryostat into 20 µm floating sections, which were blocked with 5% donkey serum and 3% bovine serum albumin in 0.1 M PBS/0.3%/Triton X-100 for 1 hour at room temperature. The sections were incubated overnight at 4℃ with monoclonal antibodies to OX-42 (1 : 500; Chemicon, Temecula, CA, USA) and GFAP (1 : 500; Neomarkers, Fremont, CA, USA) and rabbit polyclonal antibodies to phospho-p38 mitogen-activated protein kinase (MAPK) (1 : 200; Cell Signaling Technology, Danvers, MA, USA) and phospho-p44/42 MAPK. The sections were washed and incubated for 2 hours at room temperature with Alexa Fluor 555 and 488 secondary antibodies (1 : 1000 each; Invitrogen, Carlsbad, CA, USA). The stained sections were examined under a microscope (Carl Zeiss Axio Observer Z1, Jena, Germany) and photographed. Nonspecific staining was determined by omitting the primary antibody.
Glial responses were assessed in 3 sections chosen at random from the L5 spinal cord segments. The number of immunoreactive cells was counted in the medial superficial dorsal horn (laminae I-III), where most of the positive cells were found after treatment. Observers were blind to the experimental groups. The same spinal area was captured by digital camera in the same box under a 100× magnification. The data were expressed as number of positive cells/area/spinal section±SEM.
All behavioral data were expressed as mean±SEM. Comparisons between the experimental and control groups at the same time point were assessed by one-way analysis of variance (ANOVA), followed by Tukey's test for multiple comparisons. Intragroup comparisons of post-treatment and baseline values were made using one-way repeated-measures ANOVA followed by Tukey's test for multiple comparisons. Statistical evaluation was performed with SigmaPlot® software version 11 (Systat Software, Inc., San Jose, CA, USA). p<0.05 was considered statistically significant.
All the rats maintained good health and continued to gain weight throughout the experimental period. No motor dysfunction was observed in rats that received saline or lamotrigine.
When we assessed temporal changes in left hind paw WT to von Frey filaments, we observed significant decreases relative to baseline in WT on postoperative days 1 to 7 in the control group (p<0.05), indicating the development of mechanical allodynia (Fig. 1). In addition, the postoperative WTs of the control group were significantly lower than those of the LTG72 and LTG240 groups (p<0.05), but not of the LTG24 group. Intrathecally administered vehicle (20% DMSO) showed no antiallodynic effects (data not shown). Continuous administration of lamotrigine did not significantly affect rotarod performance time (Fig. 2).
Seven days after nerve ligation, the expression of OX-42 and GFAP was significantly increased on the ipsilateral, but not on the contralateral, side of the L5 spinal cord in control, but not in sham-operated rats (Figs. 3 and 4). Increased OX-42 and GFAP immunoreactivity was prominent not only in the dorsal horn (laminae I-IV) but also in the ventral horn (laminae VIII, IX) (data not shown). The increases in OX-42 and GFAP expression were dose-dependently inhibited in the LTG72 and LTG 240 groups (Figs. 3 and 4). When we counted the numbers of OX-42 and GFAP positive cells in the left L5 dorsal horn under 100× magnification, we found that they were higher in the control (434.3±55.6 and 164.0±10.2) than in the sham-operated (29.0±1.6 and 49.3±5.7, respectively) rats, indicating that microglia and astrocytes had been activated. Intrathecal lamotrigine (LTG72 and LTG240 groups) dose-dependently attenuated the ligation-induced microglial activation in the left L5 dorsal horn, as indicated by the decreased number of OX-42 positive cells (287.0±18.7 and 162.0±25.1, respectively), and dose-dependently attenuated the astrocyte activation in the same area, as indicated by the decreased number of GFAP positive cells (92.3±7.1, and 74.0±5.7, respectively).
When the localization of OX-42 (microglia), DAPI (nuclei), p-p38 (phospho-p38) and p-p44/42 [phospho-extracellular signal-regulated kinase (ERK)] was assessed (Fig. 5A), we found that the localization of OX-42 and p-p38 and of OX-42 and DAPI were identical, whereas the localization of OX-42 and p-p44/42 was not (Fig. 5B).
We have shown here that continuous intrathecal administration of lamotrigine (240 µg/day) for 7 days after SNL prevented the development of mechanical allodynia, and lower dose of lamotrigine (72 µg/day) ameliorated the allodynia. Intrathecal lamotrigine also inhibited the microglial and astrocytic activation induced by nerve ligation. Our findings suggest that the activation of both microglia and astrocytes is important in the development of nerve injury-induced neuropathic pain. Therefore, continuous intrathecal administration of lamotrigine may be a promising therapeutic intervention to prevent nerve injury-induced neuropathy.
It has already been reported that lamotrigine inhibits the release of glutamate and neuronal sensitization in the spinal cord,4 and this inhibitory effect may contribute to the anti-hyperalgesic or anti-allodynic action of neuropathic pain therapies.14,15 Several studies have assessed the analgesic activity of lamotrigine. Intraperitoneally administered lamotrigine (10, 30 and 60 mg/kg) was found to significantly attenuate mechanical hyperalgesia in the spared nerve injury model.16 Intrathecal lamotrigine at doses higher than 50 µg showed an antihyperalgesic effect in the early phase of the inflammatory pain model and reversed tactile allodynia when given after induction of inflammation.11 In addition, intrathecally administered lamotrigine induced dose-dependent antihyperalgesic effects in a rat model of neuropathic pain.8
The systemic side effects of lamotrigine include skin rash, Stevens-Johnson syndrome, and toxic epidermal necrolysis.17 Although the reported frequency of serious rash in adults using lamotrigine for adjunct therapy is 0.3%, the mortality rates for Stevens-Johnson syndrome and toxic epidermal necrolysis are 5% or less and 25-30%, respectively.17 Intrathecally administered lamotrigine may avoid these systemic side effects. Indeed, we did not observe any obvious sensory or motor dysfunction in rats receiving continuous intrathecal administration of lamotrigine. This finding suggests that intrathecal lamotrigine may be used safely in clinical settings, but further formal neurotoxicity testing is necessary.
After peripheral-nerve injury, the central terminals of primary sensory neurons may release neurotransmitters, such as glutamate, substance P, and adenosine triphosphate, which may activate spinal microglia and astrocyte, resulting in the production and release of pronociceptive mediators, such as interleukin-1β, tumor necrosis factor-α, prostaglandin E2, and adenosine triphosphate, from activated glial cells.3 These mediators may facilitate pain processing by enhancing either presynaptic release of neurotransmitters or postsynaptic excitability.3 Several lines of evidence suggested that activated microglia and astrocytes play an important role in the initiation phase of nerve injury-induced neuropathic pain. The expression levels of phosphorylated p38 and extracellular signal-regulated kinase of microglia in the spinal dorsal horn are highly enhanced 1 to 3 days after SNL, with both molecules contributing to the development of nerve injury-induced neuropathic pain.18 Furthermore, a single intrathecal injection of activated microglia, but not astrocytes, has been found to induce thermal hyperalgesia or tactile allodynia in naive animals.19
In our model, microglial cells were more powerfully activated than astrocytes. Microglia are the early responding glial cells of the CNS after injury. Peripheral nerve injury activates these microglia, which may release bioactive agents and lead to anomalous astrocytic activation. Glial activation in turn maintains such long-term pathological states as persistent pain.20,21 Astrocytes may be further activated at times longer than 7 days. We found that intrathecal lamotrigine suppressed the microglial and astrocytic activation induced by L5/6 spinal-nerve ligation. Taken together, our findings suggest that activation of microglia and astrocytes contributes to the development of nerve injury-induced pain.
SNL has been found to activate p38 MAPK in spinal microglia soon after injury.22 In addition, recent studies have suggested that spinal astrocytes have a role in maintaining neuropathic pain.1 In particular, ERK MAPK is activated in spinal astrocytes late after nerve injury, an activation required for persistent allodynia in rats.18 We have shown here that OX-42 and p-p38 co-localized, as did GFAP and p-p44/42. This colocalization suggests that microglial p38 activation and astrocytic ERK activation are involved in nerve injury-induced pain, and that intrathecal lamotrigine blocked the development of mechanical allodynia induced by SNL by suppressing microglial and astrocytic activation.
In conclusion, we have shown here that continuous intrathecal administration of lamotrigine could prevent the development of L5/6 SNL-induced mechanical allodynia in rats by suppressing the activation of microglia and astrocytes. To our knowledge, this study is the first to examine the effects of continuous intrathecally administered lamotrigine on glial cells in the L5/6 SNL ligation pain model. These results suggest that activation of microglia and astrocytes contributes to the development of nerve injury-induced pain. Therefore, intrathecal administration of lamotrigine may provide a new strategy for the prevention of neuropathic pain in clinical settings. Nevertheless, further studies are required to determine whether continuous administration of intrathecal lamotrigine could be used safely in clinical settings.
Figures and Tables
Fig. 1
Changes in mechanical sensitivity to von Frey filaments after left L5/6 spinal-nerve ligation and the effect of continuous intrathecal administration of lamotrigine (24, 72, and 240 µg per day) for 7 days after spinal nerve ligation. *p<0.05, †p<0.01 compared with control by one-way analysis of variance followed by Tukey's test (n=6 per group), ‡p<0.01 compared with baseline by one-way repeated-measures analysis of variance followed by Tukey's test (n=6 per group).
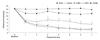
Fig. 2
The effect of continuous intrathecal administration of lamotrigine (24, 72, and 240 µg per day) for 7 days on rotarod performance time. Rotarod performance time was measured before (baseline) and after spinal nerve ligation. The results are expressed as mean±SEM. SEM, standard error of measurement.
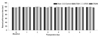
Fig. 3
Effect of lamotrigine on spinal immunoreactivity to OX-42 after left L5/6 spinal-nerve ligation. Rats were continuously administered 24, 72, and 240 µg per day lamotrigine or intrathecal saline for 7 days after spinal nerve ligation. Prominent microglial activation was observed in the ipsilateral side spinal cord of rats in the control group. No group showed significant changes in the contralateral spinal cord. Nerve ligation-induced microglial activation was markedly suppressed by intrathecal administration of 72 and 240 µg lamotrigine per day. Scale bar: 100 µm. *p<0.05 compared with the sham group, †p<0.05 compared with the control group, ‡p<0.05 compared with the LTG72 group, by one-way analysis of variance followed by Tukey's test (n=6 per group).
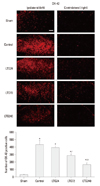
Fig. 4
Effect of lamotrigine on spinal immunoreactivity to GFAP after left L5/6 spinal-nerve ligation. Rats were continuously administered 24, 72, and 240 µg per day lamotrigine or intrathecal saline for 7 days after spinal nerve ligation. Prominent astrocytic activation was observed in the ipsilateral side spinal cord of rats in the control group. No group showed significant changes in the contralateral side spinal cord. Nerve ligation-induced astrocytic activation was markedly suppressed by 72 and 240 µg intrathecal lamotrigine. Scale bar: 100 µm. *p<0.05 compared with the sham group, †p<0.05 compared with the control group, by one-way analysis of variance followed by Tukey's test (n=6 per group). GFAP, glial fibrillary acidic protein.
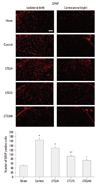
Fig. 5
(A) Immunohistochemical localization of microglia (OX-42; green), phospho-p38 (p-p38; red) and DAPI. Scale bars, 100 µm for the 100× and 200× images; 50 µm for the 400× images. p-p38: phosphorylated p38 mitogen-activated protein kinase. (B) Immunohistochemical localization of microglia (OX-42; green), phospho-p38 (p-p38; red) and phospho-p44/42 (p-ERK; red). Signals for microglia and p-p38 merged, whereas signals for microglia and p-p44/42 (p-ERK) did not. Scale bars, 100 µm for the 100× and 200× images, 50 µm for the 400× images. p-p38: phosphorylated p38 mitogen-activated protein kinase. p-ERK, phosphorylated extracellular signal regulated kinase; DAPI, 4',6-diamidino-2-phenylindole.
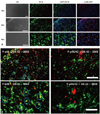
Fig. 6
(A) Immunohistochemical localization of astrocytes (GFAP; green), phospho-p38 (p-p38; red) and DAPI. Scale bars, 100 µm for the 100× and 200× images, 50 µm for the 400× images. (B) Immunohistochemical localization of astrocytes (GFAP; green), phospho-p38 (p-p38; red) and phospho-p44/42 (p-ERK; red). Signals for astrocytes and p-ERK merged, whereas signals for microglia and p-p38 did not. Scale bars, 100 µm for the 100× and 200× images, 50 µm for the 400× images. GFAP, glial fibrillary acidic protein; p-p38, phosphorylated p38 mitogen-activated protein kinase; p-ERK, phosphorylated extracellular signal regulated kinase; DAPI, 4',6-diamidino-2-phenylindole.
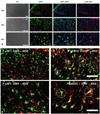
References
1. Zhuang ZY, Wen YR, Zhang DR, Borsello T, Bonny C, Strichartz GR, et al. A peptide c-Jun N-terminal kinase (JNK) inhibitor blocks mechanical allodynia after spinal nerve ligation: respective roles of JNK activation in primary sensory neurons and spinal astrocytes for neuropathic pain development and maintenance. J Neurosci. 2006. 26:3551–3560.
2. Colburn RW, Rickman AJ, DeLeo JA. The effect of site and type of nerve injury on spinal glial activation and neuropathic pain behavior. Exp Neurol. 1999. 157:289–304.


3. Marchand F, Perretti M, McMahon SB. Role of the immune system in chronic pain. Nat Rev Neurosci. 2005. 6:521–532.


4. Lees G, Leach MJ. Studies on the mechanism of action of the novel anticonvulsant lamotrigine (Lamictal) using primary neurological cultures from rat cortex. Brain Res. 1993. 612:190–199.


5. McCleane GJ. Lamotrigine in the management of neuropathic pain: a review of the literature. Clin J Pain. 2000. 16:321–326.


6. Datta S, Waghray T, Torres M, Glusman S. Amiodarone decreases heat, cold, and mechanical hyperalgesia in a rat model of neuropathic pain. Anesth Analg. 2004. 98:178–184.


7. Ma W, Du W, Eisenach JC. Intrathecal lidocaine reverses tactile allodynia caused by nerve injuries and potentiates the antiallodynic effect of the COX inhibitor ketorolac. Anesthesiology. 2003. 98:203–208.


8. Klamt JG. Effects of intrathecally administered lamotrigine, a glutamate release inhibitor, on short- and long-term models of hyperalgesia in rats. Anesthesiology. 1998. 88:487–494.


9. Kim SH, Chung JM. An experimental model for peripheral neuropathy produced by segmental spinal nerve ligation in the rat. Pain. 1992. 50:355–363.


10. Størkson RV, Kjørsvik A, Tjølsen A, Hole K. Lumbar catheterization of the spinal subarachnoid space in the rat. J Neurosci Methods. 1996. 65:167–172.


11. Lee TH, Wang CJ, Wu PC, Buerkle H, Lin SH, Yang LC. The thermal and mechanical anti-hyperalgesic effects of pre- versus post-intrathecal treatment with lamotrigine in a rat model of inflammatory pain. Life Sci. 2002. 70:3039–3047.


12. Song JG, Jun IG, Kwon MY, Park JY. The mechanical antiallodynic effect of intrathecal lamotrigine in rats with spinal nerve ligation. Korean J Pain. 2005. 18:118–124.


13. Chaplan SR, Bach FW, Pogrel JW, Chung JM, Yaksh TL. Quantitative assessment of tactile allodynia in the rat paw. J Neurosci Methods. 1994. 53:55–63.


14. Teoh H, Fowler LJ, Bowery NG. Effect of lamotrigine on the electrically-evoked release of endogenous amino acids from slices of dorsal horn of the rat spinal cord. Neuropharmacology. 1995. 34:1273–1278.


15. Woolf CJ, Mannion RJ. Neuropathic pain: aetiology, symptoms, mechanisms, and management. Lancet. 1999. 353:1959–1964.


16. Erichsen HK, Hao JX, Xu XJ, Blackburn-Munro G. A comparison of the antinociceptive effects of voltage-activated Na+ channel blockers in two rat models of neuropathic pain. Eur J Pharmacol. 2003. 458:275–282.


17. Hilas O, Charneski L. Lamotrigine-induced Stevens-Johnson syndrome. Am J Health Syst Pharm. 2007. 64:273–275.


18. Zhuang ZY, Gerner P, Woolf CJ, Ji RR. ERK is sequentially activated in neurons, microglia, and astrocytes by spinal nerve ligation and contributes to mechanical allodynia in this neuropathic pain model. Pain. 2005. 114:149–159.


19. Narita M, Yoshida T, Nakajima M, Narita M, Miyatake M, Takagi T, et al. Direct evidence for spinal cord microglia in the development of a neuropathic pain-like state in mice. J Neurochem. 2006. 97:1337–1348.


20. Kreutzberg GW. Microglia: a sensor for pathological events in the CNS. Trends Neurosci. 1996. 19:312–318.

