Abstract
Purpose
Obesity has been suggested to be linked to asthma. However, it is not yet known whether obesity directly leads to airway hyperreactivity (AHR) or obesity-induced airway inflammation associated with asthma. We investigated obesity-related changes in adipokines, AHR, and lung inflammation in a murine model of asthma and obesity.
Materials and Methods
We developed mouse models of chronic asthma via ovalbumin (OVA)-challenge and of obesity by feeding a high-fat diet, and then performed the methacholine bronchial provocation test, and real-time PCR for leptin, leptin receptor, adiponectin, adiponectin receptor (adipor1 and 2), vascular endothelial growth factor (VEGF), transforming growth factor (TGF) β, and tumor necrosis factor (TNF) α in lung tissue. We also measured cell counts in bronchoalveolar lavage fluid.
Results
Both obese and lean mice chronically exposed to OVA developed eosinophilic lung inflammation and AHR to methacholine. However, obese mice without OVA challenge did not develop AHR or eosinophilic inflammation in lung tissue. In obese mice, lung mRNA expressions of leptin, leptin receptor, VEGF, TGF, and TNF were enhanced, and adipor1 and 2 expressions were decreased compared to mice in the control group. On the other hand, there were no differences between obese mice with or without OVA challenge.
The prevalence of obesity has nearly doubled during the past 20 years, and it is associated with chronic diseases such as metabolic syndrome, obstructive sleep apnea, obesity hypoventilation syndrome, chronic obstructive pulmonary disease, and asthma.1,2
Epidemiological studies have shown that the prevalence of asthma and obesity increase concomitantly.3 Obesity worsens asthma control and is associated with asthma severity.4 However, the mechanism underlying the associations between obesity and asthma has not yet been established. Dietary factors, systemic inflammation, changes in immune function, reduced chest wall compliance, insulin resistance, comorbidities, and common genetic predisposition have been suggested as potential links between the two conditions.5-7
Obesity leads to chronic low grade systemic inflammation.5 Adipose tissue is a source of inflammatory mediators and adipokines, which may lead to inflammatory activation in lung tissue or directly activate asthma-related inflammatory genes in lung tissue.8 Elevated leptin, IL-6, tumor necrosis factor (TNF) α, transforming growth factor (TGF) β, and vascular endothelial growth factor (VEGF), and decreased adiponectin in obesity could be implicated in asthma pathophysiology. Expansion of adipose tissue in obesity leads to angiogenesis, which requires VEGF. Adipose tissue can produce VEGF in response to hypoxia or proinflammatory cytokines in obesity,9 which in turn can be associated with airway remodeling in chronic asthma.
Airway hyperresponsiveness (AHR) is a characteristic feature of asthma. Decreased lung function and increased AHR have been observed in obesity and may be the mechanistic basis for a link between obesity and asthma. Although obesity may be a risk factor for aggravating asthma, little is known regarding whether obesity itself is related to AHR, and the relationships between obesity and adipokines are even less well understood.
The aim of this study was to find out whether obesity increases AHR and airway inflammation and to investigate whether lung mRNA levels of adipokines and cytokines are different in obese mice compared to control and asthma mice.
We used the same mouse model of chronic asthma with or without obesity as we previously described.10 Mice were divided into 4 groups: a control group, an asthma group, an obese group, and an obese asthma group. Each group comprised of 6 mice. The animal experimental protocol was approved by the Animal Subjects Committee at Kangbuk Samsung Hospital.
As previously described,10 six-week-old female C57BL/6J mice (Central Lab. Animal Inc., Seoul, Korea) were fed a high-fat diet in which 45% of the calories were derived from fat (Feedlab, Guri, Korea) for a period of 16 weeks. Nonobese mice were given a standard diet.
We developed chronic asthma model in the previous report.10 Briefly, six-week-old female C57BL/6J mice were immunized by subcutaneous injection on days 0, 7, 14, and 21 with 25 µg of ovalbumin (OVA, grade V; Sigma-Aldrich, St. Louis, MO, USA) adsorbed to 1 mg of aluminum hydroxide (Aldrich, Milwaukee, WI, USA) in 200 µL of phosphate-buffered saline (PBS). Intranasal OVA challenges (20 µg/50 µL in PBS) were administered on days 27, 29, and 31 and repeated twice per week until week 18 of the study period. Age- and gender-matched control mice were treated the same way with PBS. Mice were sacrificed 24 h after the final OVA challenge, and bronchoalveolar lavage (BAL) and lung tissues were obtained.
AHR was assessed by a single-chamber whole-body plethysmograph (Allmedicus, Anyang, Korea), as previously described.10 The enhanced pause (Penh) was used to monitor airway responsiveness. Briefly, mice were exposed to nebulized PBS for 3 min to establish baseline Penh values, and to increasing concentrations of nebulized methacholine (3-50 mg/mL; Sigma-Aldrich) using an Aerosonic ultrasonic nebulizer (DeVilbiss, Somerset, PA, USA). Following each nebulization, recordings were taken for 3 min. The Penh values measured during each 3-min sequence were averaged and are expressed for each methacholine concentration.
Mice were sacrificed after measuring AHR. The trachea was cannulated and 1 mL of sterile PBS was instillated into the lung to withdraw BAL fluid. The BAL fluid was stained with a hematology analyzer, XE-2100 (Sysmex, Kobe, Japan) after cytospinning (7 min at 2000 rpm). Total cell count was performed using a hemacytometer. After counting 400 leukocytes under light microscopy, the percentage of eosinophils, neutrophils, lymphocytes, and macrophages were calculated. Supernatants were stored at -70℃, and VEGF, TNFα, and TGFβ concentrations were measured using ELISA kit (R&D, Minneapolis, MN, USA) according to the manufacturer's instructions.
After sacrifice of mice, lung tissues were removed from animals and fixed in 10% neutral buffered formalin and embedded in paraffin. Then, the paraffin blocks were cut on a microtome into 5 µm thick sections. After deparaffinization and dehydration, 5-µm thick sections were stained with hematoxylin and eosin (H&E, catalog no. GHS 132, HT110116, respectively; Sigma-Aldrich Corp.). Histological slides were examined under a light microscopy (magnification, ×200). Lung specimens were evaluated on the distribution of inflammatory cells by two pathologists.
Lung tissues were analyzed by real-time PCR for leptin, leptin receptor, adiponectin, adiponectin receptor (adipor1 & adipor2), VEGF, TGFβ, and TNFα in the same method as we had done before.10 Thus, total RNA was isolated from lung tissue using Trizol reagent (Invitrogen, Carlsbad, CA, USA). Total RNA, at a concentration of 2 µg, was reverse-transcribed with Moloney murine leukemia virus reverse transcriptase (MMLV-RT) and oligo11 12-18 primer (Invitrogen), to generate cDNA. Briefly, RNA was denatured for 10 minutes at 72℃, then immediately placed on ice for 5 minutes. Denatured RNA was mixed with MMLV-RT, MMLV-RT buffer, and dNTP mixture, and incubated for 1 h at 42℃. The reagent was inactivated by heating at 95℃ for 2 minutes. Synthesized cDNA was amplified by real-time PCR (Light-Cycler 480; Roche, Lewis, UK) using SYBR green (Invitrogen) and specific primers (Bioneer Co., Daejeon, Korea). The following primers were used: leptin, forward: 5'-TGGCTTTGGTCCTATCTGTC-3', reverse: 5'-TCCTGGTGACAATGGTCTTG-3' (GenBank accession number: BC125245); leptin receptor, forward: 5'-TGTCTCAGCTACATCTCTGC-3', reverse: 5'-CTGAACCATCCAGTCTCTTG-3' (GenBank accession number: BC082551); adiponectin, forward: 5'-AGAGAAAGGAGATGCAGGTC-3', reverse: 5'-TGAACGCTGAGCGATACACA-3' (GenBank accession number: NM009605); adipor1, forward: 5'-AGATGGGCTGGTTCTTCCTC-3', reverse: 5'-CAGACAACTCAGACTCTTCC-3' (GenBank accession number: NM1283203); adipor2, forward: 5'-ATGTTTGCCACCCCTCAGTA-3', reverse: 5'-CAGATGTCACATTTGCCAGG-3' (GenBank accession number: NM197985); VEGF, forward: 5'-GTGGACATCTTCCAGGAGTA-3', reverse: 5'-TTCATCGTTACAGCAGCCTG-3' (GenBank accession number: BC 061468); TGFβ, forward: 5'-AGTGTGGAGCAACATGTGGA-3', reverse: 5'-GTACAACTCCAGTGACGTCA-3' (GenBank accession number: BC013738); TNFα: 5'-CGCTCTTCTGTCTACTGAAC-3', reverse: 5'-CTACAGGCTTGTCACTCGAA-3' (GenBank accession number: NM013693). Each cycle consisted of denaturation at 94℃ for 15 seconds, annealing at 55℃ for 10 seconds, and extension at 72℃ for 20 seconds. Quantification was performed using the comparative 2-(delta delta Ct) method; expression levels for the target genes were normalized to the β-actin of each sample.
Animals in the obese groups fed a 45% fat diet with or without OVA challenge weighed significantly more than animals fed a normal diet from the 12 weeks of study period. At study week 18, the final body weight of the obese group and the obese asthma group was 27.3±0.9 g and 26.3±2.2 g, respectively, which were significantly higher than that of the control group (22.5±0.2 g) (Fig. 1).
Asthma mice and obese asthma mice showed significantly increased airway resistance compared to control mice. However, obese mice did not show AHR compared to control mice (Fig. 2).
Total cell numbers in BAL fluid increased significantly in the asthma, obese, and obese asthma groups compared to the control group. Asthma mice and obese asthma mice had significantly increased number of eosinophils in the BAL fluid compared to those in the control group, whereas obese mice did not show increased eosinophils in BAL fluid. Mice in the obese and obese asthma groups showed significantly increased neutrophil numbers in BAL fluid compared to control mice, whereas there was no difference in neutrophil counts between control and asthma mice (Fig. 3).
Asthma and obese asthma mice showed eosinophilic inflammation compared to control mice in H&E stain. However, diet-induced obese mice without OVA challenge did not show eosinophilic inflammation (Fig. 4).
To assess the effect of obesity on lung inflammation and expression of adipokines in mice, we measured mRNA levels of leptin, leptin receptor, adiponectin, and adiponectin receptor in lung tissue of mice by real-time PCR. Leptin and leptin receptor mRNA levels increased in obese mice and obese asthma mice compared to control mice, whereas there were no differences in leptin and leptin receptor mRNA levels between obese and obese asthma mice. Adiponectin, adipor1, and adipor2 mRNA levels decreased in obese mice compared to control mice, whereas there were no differences in adiponectin, adipor1 and adipor2 between obese and obese asthma mice (Fig. 5).
VEGF mRNA levels were higher in asthma, obese, and obese asthma mice than those in control mice. There were no differences in VEGF mRNA levels among asthma, obese, and obese asthma mice. TGFβ mRNA levels increased in obese mice compared to control mice, while TGFβ mRNA levels in obese mice showed no differences compared to asthma or obese asthma mice. TNFα mRNA levels increased in obese mice and obese asthma mice compared to control mice, whereas there was no difference between obese mice and obese asthma mice (Fig. 6A).
VEGF levels in BAL fluid also increased significantly in asthma, obese, and obese asthma mice compared to control mice. TNFα levels in BAL fluid were higher in asthma, obese, and obese asthma mice than those in control mice. Concentration of TGFβ in BAL fluid augmented in obese mice and obese asthma mice compared to control mice. (Fig. 6B).
In this study, we observed that obesity alone did not increase either AHR or eosinophilic airway inflammation in mice without asthma. When obese mice were stimulated with OVA challenge, they showed both AHR and eosinophilic lung inflammation. However, AHR or eosinophilic inflammation in lung tissue was not augmented by obesity per se. This finding is in contrast with the results of previous studies showing that AHR is a common feature of murine obesity.12,13 In the present study, we used a diet-induced obesity model with C57BL/6J mice. Until recently, genetically engineered mice such as ob/ob, db/db or Cpefat mice or obesity-susceptible mice have been used for obesity studies.14-16 Ob/ob and db/db mice have increased fat mass at 2 week of age and weigh nearly 150% more than lean, wild-type mice at the same age.14 In contrast to the genetic obesity model or obesity-susceptible mice, the diet-induced obesity model of wild type mice has only mild obesity, not extreme obesity, requiring a longer duration to develop obesity, therefore, both the magnitude and duration of obesity can affect AHR. In one study, mice fed a high fat diet did not develop AHR until 30 weeks of age.12,14 In this study, we fed mice a high-fat diet for 18 weeks; the average body weight of obese mice was 20% more than that of control mice. It is possible that if we were to develop this diet-induced obese model for a longer period of time, AHR may develop. However, we do not know whether AHR is caused by obesity itself or the aging process, as old age can increase airway resistance. Since airway eosinophil accumulation is characteristic feature of allergic lung inflammation, we examined lung tissues of mice with or without ovalbumin challenge. We found that OVA challenge in both obese and lean mice induced an eosinophil accumulation in lung tissue as well as increased eosinophil numbers in BAL fluid, however, we found no eosinophilic inflammation in obese mice without OVA challenge as well as no difference in eosinophilic lung inflammation between obese and lean asthma mice. This is in contrast to other studies. One murine study demonstrated that OVA challenge in obese mice augmented marked eosinophil accumulation in lung tissue surrounding the bronchi and bronchiol,17 but not in BAL fluid. Recently, in another murine study with diet-induced obese mice, eosinophilic lung inflammation was found to be augmented in high fat diet group with OVA challenge compared to low fat diet group with OVA challenge.16 Even though eosinophilic lung inflammation was observed in high fat diet mice, it failed to enhance AHR compared to low fat diet group, which is similar to our study. This implies that eosinophilic lung inflammation in obesity is not related to AHR. We detected an increase in neutrophils in the BAL fluid of obese mice, suggesting that obesity is associated with neutrophilic inflammation rather than eosinophilic inflammation.
Leptin and adiponectins are hormones that are closely associated with obesity. In this study, we showed that leptin and leptin receptor were markedly increased in the lung tissues of obese mice compared to both control and asthma mice, however, we found no distinct differences between obese mice with or without OVA challenge. These results suggest that leptin or leptin receptor may not be associated with either eosinophilic lung inflammation or AHR. In a previous study using a mouse model, it was found that leptin augments AHR only in OVA-challenged mice but not in PBS-challenged mice, implying that leptin itself may not increase AHR.18
In contrast to leptin, adiponectin declines with obesity and has both pro- and anti-inflammatory effects.19 Three adiponectin receptors have been identified; adipor1, adipor2 and T-cadherin.20 We found that there was a negative correlation between adiponectin expression in lung tissue and obesity. Lung mRNA expressions of adiponectin and adipor1, 2 in obese mice decreased significantly compared to control mice, but lung expression of adiponectin and adiponectin receptors were not influenced by OVA challenge. There were no significant differences between obese mice and obese asthma mice or between asthma mice and control mice. Considering the decline in adiponectin and adipor1, and 2 in obese mice, but not in asthma mice, the adiponectin-related immunomodulatory pathway is not likely to be associated with AHR or eosinophilic inflammation. However, a previous study showed that exogenous adiponectin administration in mice leads to a suppression of allergen-induced AHR, airway inflammation, and TH2 cytokine expression in the lung.21
VEGF is one of the key mediators related to airway remodeling in asthma, and VEGF serum levels are increased in obesity.22 In a recent study, leptin was shown to stimulate the release of VEGF by airway smooth muscle cells.23 Our results indicated that mRNA expression of VEGF as well as VEGF levels in BAL fluid was elevated in asthma, obese, and obese asthma mice compared to control mice. Therefore, our results indicate that leptin is less likely to stimulate VEGF release in lung tissue since the VEGF mRNA level was elevated in asthma mice compared to control mice. Thus, even though VEGF is related to both asthma and obesity, VEGF itself may not be the main factor modulating the pathophysiology of obese asthmatics.
TNFα is increased in the obese state, and increased TNFα is related to induce AHR.24,25 TGFβ is an important factor of airway remodeling and increased in asthmatic patients,26,27 and TGFβ may also contribute to airway inflammation by enhancing VEGF release through the phosphatidylinositol 3-kinase pathway.28 In our study, lung mRNA expression of TNFα was increased in obese and obese asthma mice compared to control mice. However, there were no significant differences between obese mice and obese asthma mice. Our study also showed that lung mRNA expression of TGFβ in obese mice was distinctly elevated compared to control mice. Thus, TNFα and TGFβ are more likely to be associated with obesity than with asthma.
The main limitation of our study was that we used a diet-induced mildly obese mouse model. Therefore, we cannot exclude the possibility that the obese mice may have developed increased airway resistance to methacholine inhalation if the model had been developed for a longer period of time. However, genetically engineered mice or obesity-susceptible mice can be extreme obese, which is not physiologic, and may have lung phenotypes different from wild type mice. Therefore, extreme obese mice of those strains may not represent the effect of obesity per se on airway inflammation in asthma. We used wild type mice to examine the effect of obesity on AHR and airway inflammation, and found that diet-induced, mildly obese wild type mice did not develop AHR or eosinophilic lung inflammation until the age of 24 weeks. Furthermore, there are only a few studies on lung inflammation and AHR in mice with both obesity and asthma.
Our results demonstrated that diet-induced, mild obesity did not augment AHR or eosinophilic airway inflammation. In addition, lung expression of leptin, leptin receptor, TGFβ, VEGF, and TNFα was increased in obese mice, but there were no distinct differences according to OVA challenge in obese mice. These findings suggest that these obesity-related adipokines and proinflammatory cytokines may not be associated directly with asthma phenotypes including AHR or eosinophilic inflammation. It is needed to study the relationship between obesity and asthma in severe obese model fed high fat diet for longer period of time.
Figures and Tables
Fig. 1
Effect of high-fat diet on body mass. Obese mice and obese asthma mice weighed significantly more than control mice from the 12 weeks of study period to the end of the study period. There was no significant difference in weight between asthma and control mice. *p<0.01 compared to control mice.
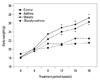
Fig. 2
Airway hyperresponsiveness to methacholine. Asthma mice and obese asthma mice showed increased airway resistance to methacholine. However, obese mice did not show airway hyperresponsiveness compared to control mice. *p<0.05 compared to control mice. PBS, phosphate-buffered saline.
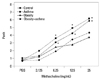
Fig. 3
Effect of obesity and ovalbumin challenge on total and differential cell counts in bronchoalveolar lavage fluid. Total cell numbers indicated a significant increase in asthma mice and obese asthma mice. Obese mice showed increases in neutrophil counts, but not in eosinophil counts compared to control mice. Eosinophils were markedly increased in both asthma mice and obese asthma mice. *p<0.01 compared to control and obese mice. **p<0.01 compared to control and asthma mice. BAL, bronchoalveolar lavage.
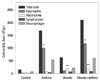
Fig. 4
Airway inflammation induced by obesity and ovalbumin challenge. Compared to control mice (A), mice with OVA challenge showed eosinophilic inflammation (B). In comparison with obese mice without OVA challenge (C), obese mice with OVA challenge (D) demonstrated eosinophilic lung inflammation. Hematoxylin and eosin staining, ×200.
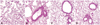
Fig. 5
Adipokines mRNA expression in lung tissue of mice. Total RNA was extracted from lung tissue. (A) Leptin and leptin receptor mRNA levels were higher in obese and obese asthma mice than those in asthma or control mice. (B) Adiponectin, adiporeceptor1, and adiporeceptor2 mRNA expressions decreased in obese mice compared to control mice. *p<0.05 compared to control mice.
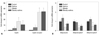
Fig. 6
VEGF, TNFα and TGFβ mRNA lung expressions and levels in bronchoalveolar lavage fluid. (A) VEGF expression increased significantly in asthma, obese, and obese asthma mice compared to control mice. TGFβ mRNA expression was higher in obese mice than that in control mice and TNFα mRNA expression augmented in obese and obese asthma mice compared to control mice. (B) VEGF levels in bronchoalveolar fluid were also higher in asthma, obese and obese asthma mice than those in control mice. TGFβ concentration increased in obese and obese asthma mice compared to control mice and TNFα concentration increased in asthma, obese and obese asthma mice compared to control mice. Data were normalized to the β-actin of each sample. All values are expressed as mean±SEM for 6 mice. *p<0.05 compared to control mice. VEGF, vascular endothelial growth factor; TNF, tumor necrosis factora; TGF, transforming growth factorb; BAL, bronchoalveolar lavage.
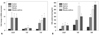
ACKNOWLEDGEMENTS
Jung Yeon Shim received grant support from the IN-SUNG Foundation for Medical Research.
References
4. Taylor B, Mannino D, Brown C, Crocker D, Twum-Baah N, Holguin F. Body mass index and asthma severity in the National Asthma Survey. Thorax. 2008; 63:14–20.


6. Litonjua AA, Gold DR. Asthma and obesity: common early-life influences in the inception of disease. J Allergy Clin Immunol. 2008; 121:1075–1084.


7. Husemoen LL, Glümer C, Lau C, Pisinger C, Mørch LS, Linneberg A. Association of obesity and insulin resistance with asthma and aeroallergen sensitization. Allergy. 2008; 63:575–582.


8. Wajchenberg BL. Subcutaneous and visceral adipose tissue: their relation to the metabolic syndrome. Endocr Rev. 2000; 21:697–738.


9. Hausman GJ, Richardson RL. Adipose tissue angiogenesis. J Anim Sci. 2004; 82:925–934.
10. Ryu SL, Shim JW, Kim DS, Jung HL, Park MS, Park SH, et al. Expression of peroxisome proliferator-activated receptor (PPAR)-α and PPAR-γ in the lung tissue of obese mice and the effect of rosiglitazone on proinflammatory cytokine expressions in the lung tissue. Korean J Pediatr. 2013; 56:151–158.


11. Zhao AZ, Shinohara MM, Huang D, Shimizu M, Eldar-Finkelman H, Krebs EG, et al. Leptin induces insulin-like signaling that antagonizes cAMP elevation by glucagon in hepatocytes. J Biol Chem. 2000; 275:11348–11354.


12. Johnston RA, Theman TA, Lu FL, Terry RD, Williams ES, Shore SA. Diet-induced obesity causes innate airway hyperresponsiveness to methacholine and enhances ozone-induced pulmonary inflammation. J Appl Physiol. 2008; 104:1727–1735.


13. Lu FL, Johnston RA, Flynt L, Theman TA, Terry RD, Schwartzman IN, et al. Increased pulmonary responses to acute ozone exposure in obese db/db mice. Am J Physiol Lung Cell Mol Physiol. 2006; 290:L856–L865.
14. Shore SA. Obesity, airway hyperresponsiveness, and inflammation. J Appl Physiol. 2010; 108:735–743.


15. Johnston RA, Theman TA, Shore SA. Augmented responses to ozone in obese carboxypeptidase E-deficient mice. Am J Physiol Regul Integr Comp Physiol. 2006; 290:R126–R133.


16. Dietze J, Böcking C, Heverhagen JT, Voelker MN, Renz H. Obesity lowers the threshold of allergic sensitization and augments airway eosinophilia in a mouse model of asthma. Allergy. 2012; 67:1519–1529.


17. Calixto MC, Lintomen L, Schenka A, Saad MJ, Zanesco A, Antunes E. Obesity enhances eosinophilic inflammation in a murine model of allergic asthma. Br J Pharmacol. 2010; 159:617–625.


18. Shore SA, Schwartzman IN, Mellema MS, Flynt L, Imrich A, Johnston RA. Effect of leptin on allergic airway responses in mice. J Allergy Clin Immunol. 2005; 115:103–109.


19. Fantuzzi G. Adiponectin and inflammation: consensus and controversy. J Allergy Clin Immunol. 2008; 121:326–330.


20. Yamauchi T, Kamon J, Ito Y, Tsuchida A, Yokomizo T, Kita S, et al. Cloning of adiponectin receptors that mediate antidiabetic metabolic effects. Nature. 2003; 423:762–769.


21. Shore SA, Terry RD, Flynt L, Xu A, Hug C. Adiponectin attenuates allergen-induced airway inflammation and hyperresponsiveness in mice. J Allergy Clin Immunol. 2006; 118:389–395.


22. Silha JV, Krsek M, Sucharda P, Murphy LJ. Angiogenic factors are elevated in overweight and obese individuals. Int J Obes (Lond). 2005; 29:1308–1314.


23. Shin JH, Kim JH, Lee WY, Shim JY. The expression of adiponec tin receptors and the effects of adiponectin and leptin on airway smooth muscle cells. Yonsei Med J. 2008; 49:804–810.


24. Hotamisligil GS, Shargill NS, Spiegelman BM. Adipose expression of tumor necrosis factor-alpha: direct role in obesity-linked insulin resistance. Science. 1993; 259:87–91.


25. Thomas PS, Yates DH, Barnes PJ. Tumor necrosis factor-alpha increases airway responsiveness and sputum neutrophilia in normal human subjects. Am J Respir Crit Care Med. 1995; 152:76–80.


26. Vignola AM, Mirabella F, Costanzo G, Di Giorgi R, Gjomarkaj M, Bellia V, et al. Airway remodeling in asthma. Chest. 2003; 123:3 Suppl. 417S–422S.


27. Redington AE, Madden J, Frew AJ, Djukanovic R, Roche WR, Holgate ST, et al. Transforming growth factor-beta 1 in asthma. Measurement in bronchoalveolar lavage fluid. Am J Respir Crit Care Med. 1997; 156(2 Pt 1):642–647.