Abstract
Purpose
Most chemical transfection reagents are ineffective for the transfection of cells in suspension, such as leukemic cell and stem cell lineages. We developed two different types of viroplexes, cationic Sendai F/HN viroplexes (CSVs) and protamine sulfate-condensed cationic Sendai F/HN viroplexes (PCSVs) for the efficient transfection of T-leukemic cells.
Materials and Methods
The viroplex systems were prepared by reconstitution of fusogenic Sendai F/HN proteins in DMKE (O,O'-dimyristyl-N-lysyl glutamate) cationic liposomes. The viroplexes were further optimized for plasmid DNA and siRNA delivery to suspension cells. The particle size and surface charge of the viroplexes were analyzed with a ζ-sizer. Transfection of plasmid DNA (pDNA) and small interfering RNA (siRNA) by CSVs or PCSV was evaluated by measurement of transgene expression, confocal microscopy, FACS, and RT-PCR.
Results
The optimized CSVs and PCSVs exhibited enhanced gene and siRNA delivery in the tested suspension cell lines (Jurkat cells and CEM cells), compared with conventional cationic liposomes. In the case of pDNA transfection, the CSVs and PCSVs show at least 10-fold and 100-fold higher transgene expression compared with DMKE lipoplexes (or lipofectamine 2000), respectively. The CSVs showed more effective siRNA delivery to the suspension cells than cationic liposomes, as assessed by confocal microscopy, FACS, and RT-PCR. The effective transfection by the CSVs and PCSVs is presumably due to fusogenic activity of F/HN proteins resulting in facilitated internalization of pDNA and siRNA.
A variety of chemical gene delivery systems have been utilized for large DNA molecules such as plasmid DNA (pDNA) and small RNAs such as ribozymes, small interfering RNA, and microRNA.1 Although the therapeutic effectiveness of viral vectors was proved by successful clinical trials for some genetic diseases, non-viral vectors have also become more attractive for the delivery of genetic material, especially small DNA molecules.2 However, most chemical delivery systems exhibit relatively low gene transfection to suspension cell lines such as blood cancer cells and stem cell lineages. There are very limited reports regarding chemical vectors-mediated transfection of suspension cells.3
Virosomes are chimeric gene transfer systems which combine viral and non-viral features due to the viral constituents of envelop proteins and artificial liposomal vesicles.4 Among non-viral delivery vehicles, virosomes are known to be one of the most effective transfection systems and many different types of virosomes have been formulated from several enveloped viruses including influenza, HSV, SV40, and Sendai virus.5-9 We previously developed Sendai virosomes containing two major glycoproteins, hemagglutinin-neuraminidase (HN) and fusion (F) proteins, anchored in the outer leaflet of the lipid bilayers and demonstrated their gene-transferring efficiency in several in vitro cancer models.10 In addition, a similar formulation of F/HN virosomes, called HVJ particles, also showed that F/HN proteins are functionally effective in the delivery of proteins, pDNA and siRNA into cells.5,11 The effective delivery of cargo molecules is a result of the active membrane fusion process mediated via F/HN proteins in the neutral pH of the extracellular medium.12
In this study, we formulated two different types of Sendai F/HN virosomes, cationic Sendai virosomes (CSVs) and protamine sulfate (PS)-condensed cationic Sendai virosomes (PCSVs) for the transfection of suspension cells which are difficult to be transfected by conventional chemical vectors. Plasmid DNA and siRNA oligonucleotides were effectively complexed with the optimized CSVs and PCSVs, forming viroplexes. The virosome formulations containing pDNA or siRNA were tested in Jurkat and CEM T-leukemia cells.
Jurkat cells (TIB-152, T-lymphocyte leukemia) and CEM cells (CRL-2265, T-lymphoblastic leukemia) were cultured in RPMI (Gibco, Calsbad, CA, USA) supplemented with 10% heat-inactivated fetal bovine serum (Gibco), 100 units/mL penicillin and 100 µg/mL streptomycin at 37℃ under 5% CO2.
The plasmid pAAV-CMV-Luc encoding luciferase and pEGFP-N1 encoding green fluorescence protein (Clontech Laboratories Inc., Mountain View, CA, USA) were propagated in the DH5α strain of E. coli in selective LB media with ampicillin and kanamycin.13 The plasmids were isolated and purified in a large scale preparation of plasmid DNA. Purity was confirmed by 1% agarose gel electrophoresis followed by ethidium bromide staining and the DNA concentration was measured by UV absorption at 260 nm. Fluorescein isothiocyanate (FITC)-label control siRNA (sense; 5'-CCUACGCCACC AAUUUCGUdTdT-3', antisense; 5'-ACGAAAUUGGU GGCGUAGGdTdT-3'), Vimentin siRNA (sense; 5'-UGA AGCUGCAACUACCAA-3', antisense; 5'-UUGGUA GUUAGCAGCUUCA-3') and glyceraldehyde 3-phosphate dehydrogenase (GAPDH) primers (sense; 5'-CGGGAAGCTTGTGATCAATGG-3', antisense; reverse 5'-CAGTCCATGCCATCACTGCC-3') were supplied by Bioneer Co. (Deajeon, Korea), Genolution Pharmaceuticals Inc. (Seoul, Korea), and Cosmogenetech (Seoul, Korea), respectively.
The Sendai virus (ATCC no.1698936, VR 907) was grown in an allantoic sac of 10-day-old embryonated chicken eggs as previously described.4 Briefly, the allantoic fluid collected from the infected eggs was centrifuged at 3000 g for 30 min at 4℃ and the clear supernatant was collected. The virus in the supernatant was pelleted by centrifugation at 100000 g for 1 h at 4℃ in an ultracentrifuge (Centrikon T-1180; Kontron Instruments, Milano, Italy). The virus pellet was resuspended in a small volume of phosphate-buffered saline (PBS) and aliquoted in batches of 10 mg of protein and stored at -70℃ until further use. Hemagglutination activity of the harvested Sendai virus was titrated in 96-well microplates by incubation of serially diluted virus and 1% human erythrocytes at 4℃ for 1 h. The hamagglutination assay (HA) titer was recorded as the reciprocal of the highest dilution showing positive HA and expressed as hamagglutinating unit (HAU) per mL.
For purification of Sendai F and HN-proteins, a pellet of 10 mg of Sendai virus was resuspended in 2 mL of PBS containing 1% Triton X-100. After incubation at 20℃ for 2 h, the suspension was centrifuged at 100000 g for 1 h at 4℃ to remove detergent-insoluble substances. The detergent was removed from the clear supernatant by stepwise addition of SM2 Bio-Beads (Bio-Rad Lab, Hercules, CA, USA) with constant rocking. The turbid suspension was separated from the Bio-Beads using a 26-gauge needle. The purified proteins were quantified by the Bio-Rad DC protein assay (Bio-Rad Lab, Hercules, CA, USA), analyzed by sodium dodecyl sulfate-polyacrylamide gel electrophoresis and stored at 4℃.
A mixture of DMKE (O,O'-dimyristyl-N-lysyl glutamate)/cholesterol (Chol) (10 mg, 5 : 5 molar ratio) was dissolved in 1 mL of chloroform/methanol (2 : 1, v/v).14 The lipid mixture was dried to a thin film under nitrogen gas and then vacuum-desiccated for 1 h to remove residual traces of organic solvent. The lipid film was then fully hydrated with 1 mL of distilled water. The resulting multilamellar vesicles were freeze-thawed 10 times and extruded 10 times through 100 nm pore diameter polycarbonate membranes using an Avanti Mini-Extruder (Avanti Polar Lipids, Alabaster, AL, USA). The resulting cationic liposomes were stored at 4℃. DNA (or siRNA)-liposome complexes were prepared by gentle mixing of plasmid DNA (or siRNA) at various N/P ratios. After incubation for 20 min at room temperature, Sendai viral F/HN proteins were added to the lipoplex solution (1 : 5, protein wt/lipid wt), which was further incubated for 30 min at room temperature with gentle mixing. The resulting complex is called cationic Sendai viroplexes (CSVs). Protamine sulfate (PS)-condensed DNA-encapsulating cationic Sendai viroplexes (PCSVs) were also prepared by pre-complexation of pDNA with PS (0.6 : 1, N/P ratio), followed by sequential addition of the DMKE/Chol cationic liposomes and purified F/HN proteins.
To determine whether complete complexation of pDNA (or siRNA) with the virosomes occurred, the prepared CSVs, and PCSVs (1 µg nucleic acid) analyzed on a 1% TAE agarose gel at 100 mV for 20 min. Bands of pDNA or siRNA were visualized with UV illuminator (Fluor-S MultiImage, Bio-Rad, Hercules, CA, USA).
The sizes and zeta-potentials of DMKE/Chol lipoplexes (6 : 1, N/P ratio of nucleic acid and lipid), CSVs (6 : 1, N/P ratio; 1 : 1, wt ratio of nucleic acid and F/HN proteins), and PCSVs (6 : 1, N/P ratio; 1 : 1 : 1, wt ratio of nucleic acid, F/HN and PS) were measured using a zetasizer (Zetasizer4, Malvern, UK). Viroplex suspensions in deionized water were loaded into the capillary cells mounted on the zetasizer, and their size and zeta-potentials were measured five times per sample at 25℃.
Suspension cells (Jurkat and CEM) were seeded into 6-well plates and grown for 24 h at 37℃. The CSVs or PCSVs containing pAAV-CMV-Luc prepared as described above were added to the cells (1×106) in a serum-free medium (1 µg DNA each well). The serum-free medium was replaced by cell culture medium 4 h later and the transfected cells were incubated for additional 24 h and 48 h.
For quantification of luciferase expression, the transfected cells were lysed with 200 µL of lysis buffer (0.05% Triton X-100, 2 mM EDTA, 0.1 M Tris, pH 7.8). The luciferase activities of the cell lysates (10 µL) were measured using a luciferase assay system (Promega, Madison, WI, USA) in a MultiLumat LB9506 luminometer (Berthold Tech., Bad Wildbad, Germany). The luciferase activity of each sample was expressed as relative light units per mg of total extracted proteins. The protein concentrations of the cell lysates were determined by the Bio-Rad DC protein assay (Bio-Rad Lab).
For flow cytometry analysis of GFP expression, transfected Jurkat and CEM cells were washed in PBS and then treated with 0.2% paraformaldehyde for 5 min at room temperature in the dark. The transfected cells expressing GFP were quantified by flow cytometry (BD FACScalibur, BD Bioscience, San Jose, CA, USA).
The CSVs containing FITC-labeled control siRNA (200 pmole) were prepared as described earlier and added to Jurkat or CEM cells (1×106) in a 5-mL culture tube. After incubation for varied time periods, the cells were washed in PBS and then treated with 0.2% paraformaldehyde for 5 min at room temperature in the dark. The cells transfected with FITC-siRNA were quantified by flow cytometry and the mean of fluorescence intensity (MFI) of the transfected cells was measured.
Cells transfected for 4 h were fixed with 0.2% paraformaldehyde for 5 min at room temperature in the dark. The fixed cells were stained using a diamidinophenylindole (DAPI) staining kit (Vector Laboratories Inc., Burlingame, CA, USA) according to the manufacture's protocol. The cells transfected with FITC-siRNA were examined by a confocal microcopy (FV-1000, Olympus, Center Valley, PA, USA).
Jurkat and CEM cells (1×106) grown in 6-well plates were transfected with CSVs containing vimentin siRNA (200 pmole) for 4 h and then further cultured for 24 h. For comparison, the same cells were electroporetically transfected under optimized conditions of 6 pulses for 10 msec at 320 V/cm. Total RNA was then purified from the transfected cells using Trizol (Invitrogen, Carlsbad, CA, USA). Reverse transcription was performed at 65℃ for 5 min. RNA (2 µg) from each sample was reversely transcribed using 200 units of reverse transcriptase (Invitrogen), oligo-dT random primers (Invitrogen), and forward and reverse primers for vimentin and GAPDH at 37℃ for 1 h. The resulting cDNAs were sequentially amplified with 2.5 units of a G-Taq kit (Cosmogenetech, Seoul) and 10 pmol of each primer in a PTC-200 thermocycler (Bio-RAD) with the following program: 25 cycles (22 cycles for β-actin) of denaturation (94℃ for 5 sec), annealing (55℃ for 20 sec), and extension (72℃ for 7 min). The amount of each mRNA transcript was normalized to that of GAPDH.
Cationic DMKE liposomes were complexed with varied amounts of pDNA and then analyzed on an agarose gel to find the optimal N/P ratio for stable lipoplex formation (Fig. 1A). The DMKE liposomes were able to complete complex transformation with pDNA at 3 : 1 N/P ratio. At this ratio, addition of Sendai F/HN proteins to the lipoplexes (1 : 1 wt ratio of pDNA and protein) to form CSVs or pDNA condensation by PS (1 : 0.6 N/P ratio or 1 : 1 wt ratio of PS and pDNA) to form PCSVs did not affect pDNA complexation with the cationic liposomes (Fig. 1B).
Meanwhile, siRNA molecules were stably complexed with DMKE liposomes at a 6 : 1 N/P ratio (Fig. 1C). Addition of Sendai F/HN proteins to DMKE lipoplexes with siRNA did not interfere with the charge interaction between cationic lipids and siRNA (Fig. 1D). Unlike pDNA, siRNA molecules were not effectively condensed by polycationic PS (data not shown). All CSVs and PCSVs for further transfection studies were prepared at 6 : 1 N/P ratio and 1 : 1 wt ratio of pDNA and F/HN protein. Under these conditions, all of the available cationic lipids are presumably involved in pDNA or siRNA binding.
In order to examine any biophysical changes induced by complexation of nucleic acids and incorporation of Sendai F/HN proteins, the particle sizes and zeta-potentials of cationic lipoplexes, CSVs, and PCSVs with plasmid DNA or siRNA were measured and compared (Table 1). The DMKE liposomes became larger after complexation with pDNA or siRNA while the cationic surface charge of the vesicles slightly decreased. Interestingly, addition of F/HN proteins caused the DMKE lipoplexes to become more compact and less cationic. In addition, pDNA condensation by PS before complexation with cationic lipids further reduced the size of viroplex vesicles, but had little effect on their surface charges.
The transfection efficiencies of CSVs and PCSVs were compared with two different types of conventional cationic liposomes: Lipofectamine 2000 and DMKE liposomes. According to the in vitro transfection data using Jurkat and CEM cells (Fig. 2A and B), the incorporation of Sendai F/HN proteins to DMKE liposomes was able to enhance the transfection efficiency of DMKE lipoplexes in Jurkat and CEM cell by 5-14 and 20-30 fold, respectively. Interestingly, condensation of pDNA in the preparation of PCSVs further enhanced their transfection capability by 8-100 fold in both cell lines. According to these results, PCSVs exhibited the most effective transfection and the luciferase expression mediated by PCSVs was at least 50-fold higher than that of conventional DMKE cationic lipoplexes.
Transfection efficiencies were also confirmed by FACS analysis of cells transfected with pDNA encoding GFP. PCSVs were able to transfect over 26% of Jurkat cells and 11% of CEM cells by 48 h post-transfection (Fig. 2C and D). Under the same transfection conditions, conventional DMKE and Lipofectamine 2000 lipoplexes exhibited far less efficient transfection to the same cells (>11% of Jurkat cells and >2% of CEM cells). The transfection efficiency of CSVs was lower than that of PCSVs in both cell lines.
Transfection mediated by CSVs or PCSVs was sustained for a longer period, and further enhanced with time after 48 h compared to Lipofectamine lipoplexes. Transfected cells expressing GFP were 11% of Jurkat cells and 5% of CEM cells at 24 h post-transfection with PCSVs. The number of transfected cells more than doubled 48 h later, to 26% and 11% (Fig. 2C and D). Sustained and enhanced transfection by PCSVs was confirmed by analysis of luciferase expression in the transfected cells (Fig. 2A and B). The levels of luciferase expression mediated by PCSVs in Jurkat and CEM cells at 48 h post-transfection were 15 and 10 fold higher than those at 24 h were, respectively.
Jurkat and CEM cells were treated with conventional cationic lipoplexes or CSVs of FITC-labeled siRNA, and transfected cell numbers and MFI were then measured by FACS. Generally, CSVs exhibited higher siRNA transfection than the two different types of conventional lipoplexes at 4 h and 24 h post-transfection (Fig. 3A and B). At 4 h post-transfection, 77% of Jurkat cells and 70% of CEM cells were transfected by CSVs, with 97027 and 82825 MFI, respectively. The DMKE lipoplexes exhibited relatively lower transfection, with 70% of Jurkat cells being transfected with 68029 MFI and 62% of CEM cells being transfected with 67010 MFI. This result suggests that incorporation of Sendai F/HN proteins enhances siRNA transfection efficiency of the DMKE lipoplexes. Even though chemically modified siRNA was adopted for transfection, the number of transfected cells and MFI were significantly reduced with time, to 45% of Jurkat cells with 27445 MFI and 34% of CEM cells with 30187 MFI. Efficient siRNA transfection by the viroplexes was further confirmed by analysis with confocal laser microscopy under which CSVs showed more efficient siRNA transfection into Jurkat and CEM cells than the conventional DMKE or Lipofectamine 2000 lipoplexes (Fig. 3C and D).
In order to demonstrate functional siRNA transfection mediated by the viroplexes, siRNA molecules of vimentin, a member of the intermediate filament structure, were utilized for transfection. Jurkat and CEM cells were transfected with vimentin siRNA by CSVs, DMKE lipoplexes or Lipofectamine 2000 lipoplexes. The same cell lines were electroporetically transfected under optimized conditions. Among the chemically transfected cells, only the cells transfected by CSVs exhibited a significant reduction of vimentin mRNA at 24 h post-transfection (Fig. 4). Transfection using CSVs reduced the level of vimentin mRNA in Jurkat and CEM cells by 36% and 35%, respectively. The electroporetic transfer of the same amount of vimentin siRNA reduced the level of vimentin mRNA in the cell lines by 47% and 14%, respectively. On the other hand, transfection mediated by DMKE or Lipofectamine 2000 lipoplexes resulted in less reduction in both cell lines.
Human leukemic T cell lineages such as Jurkat and CEM cells have been utilized as a popular cell model for a variety of studies in immunology and cancer biology.15 However, these cell types are also known to be extremely difficult to transfect non-virally for reasons that are still unclear.16 There have been a few reports on leukemic cell transfection mediated by chemical vectors, but transfection efficiencies using chemical vectors were unsatisfactory compared to those in adherent cancer cell lines.17,18 There have been no systematic or quantitative reports of transfection of suspension leukemic T cells mediated by chemical vector systems. Even a few related reports were only able to show qualitative data in leukemic cells. Hence, most transfection of leukemic cells in those studies was done by electroporation even though that method results in serious physical damages to the target cells.19 Therefore, it is necessary to develop a more effective and convenient chemical transfection system for research utilizing this type of cell.
In this study, we show that CSV or PCSV formulations of Sendai F/HN viroplexes are a useful transfection system for pDNA or siRNA transfection of Jurkat and CEM cells compared with conventional DMKE or Lipofectamine 2000 lipoplexes. Based on the results of luciferase transfection, the quantitative addition of Sendai F/HN to DMKE cationic liposomes was able to significantly enhance the transfection efficiency in both cell lines. As previously speculated by Kim, et al., the positively charged virosomal surface may facilitate the initial interaction with the cell surface, and the specific association of F/HN proteins with cell membranes may also facilitate internalization of cargo molecules, presumably due to their fusogenic activity.10 This result suggests that the Sendai F/HN proteins are functionally active components in the transfection process and that target cells have reactive receptor molecules on their cell surface, such as sialic acid-containing gangliosides and asialoglycoprotein receptors.4,8,20
In addition, pDNA condensation with PS before complexation with DMKE liposomes further enhanced the transfection efficiency of CSVs. The number of cells transfected with GFP by PCSVs was 2-fold higher than the number transfected by CSVs. Stable pDNA condensation by PS might be helpful in pDNA complexation and encapsulation into the cationic membranes, resulting in better protection of the DNA molecules.10,21 As suggested previously, the 21 basic arginine residues of protamine may also function as a nuclear localization signal after internalizations.10 We tried to formulate PCSVs containing siRNA molecules to prove this hypothesis. However, siRNA molecules do not require transport to the nucleus since they function in the cytoplasm. In addition, we did not observe a positive outcome using PS-condensed siRNA in terms of transfection and gene silencing in Jurkat and CEM cells.
Interestingly, the transgene expression mediated by Sendai viroplexes, both CSVs and PCSVs, was sustained for a relatively longer period of time compared to conventional lipoplexes. Generally, the extent of transfection by CSVs or PCSVs was much higher at 48 h post-transfection than at 24 h. This implies that Sendai F/HN proteins may function to protect the gene construct in the cell.
The transfection enhancement by Sendai F/HN proteins was also seen in siRNA-transfecting experiments. FACS analysis and confocal microscopy examination showed that CSVs are more effective in terms of siRNA transfection to Jurkat and CEM cells than the other formulations tested. However, the fluorescence intensity of the transfected cells decreased with time. Even though the sense and antisense siRNA was chemically modified at deoxygenated 3' endings for protection against enzymatic degradation,22 MFI of the transfected cells at 4 h post-transfection was reduced 2-3-fold in 24 h. Presumably, adoption of more stable siRNA structures recently suggested would yield more effective gene silencing for a longer period of time.23
Nevertheless, the down regulation of vimentin expression by CSVs containing vimentin siRNA demonstrated the effective delivery of functionally active siRNA to the cytoplasm of Jurkat and CEM cells. Vimentin is known to be a constitutive member of the intermediate filament family and plays a significant role in supporting and anchoring organelles in the cytosol.24 The reduction of vimentin mRNA levels by CSVs was comparable to that achieved by electroporation which is known to be one of the most efficient procedures for siRNA transfection.18 Among the tested siRNA-transferring systems, only transfection using CSVs showed a statistically meaningful reduction of vimentin mRNA in the transfected cells.
In recent years, poly(2-dimethylamino)ethyl methacrylate (PDMAEMA)-based star-shaped nanoparticles were introduced as a transfection agent for Jurkat T cells and primary T lymphocytes.25 According to the report, PDMAEMA-based nanoparticles formulated at 10 : 1 N/P ratio were able to transfect 31% of Jurkat cells and induce 40% silencing of target gene in primary T lymphocytes regardless their substantial cytotoxicity. Even though transfection conditions and formulations were different, the efficiencies of gene transfection (26%) and gene silencing (36%) in Jurkat cells in this study were comparable to the previous results.
In summary, we formulated two different types of cationic Sendai F/HN viroplexes, CSVs and PCSVs, for pDNA and siRNA transfection into Jurkat and CEM leukemic T cells. In general, the Sendai viroplex systems exhibited more effective transfection to the target cells compared to conventional DMKE cationic lipoplexes and commercially available Lipofectamine 2000 lipoplexes. These results suggest that viroplex systems can be widely utilized for the transfection of many types of cancer cells, including leukemic T cells.
Figures and Tables
Fig. 1
Gel retardation analysis of cationic Sendai F/HN viroplexes encapsulating pDNA or siRNA. DMKE cationic liposomes and Sendai F/HN virosomes were complexed with pDNA (A) or siRNA (C) at various N/P ratios. CSVs and PCSVs were prepared at a 3 : 1 N/P ratio with pDNA (B) and a 6 : 1 N/P ratio with siRNA (D). All samples were analyzed on a 1% TAE agarose gel at 100 mV for 20 min. M; 1 kb DNA ladder. CSVs, cationic Sendai F/HN viroplexes; PCSVs, protamine sulfate-condensed cationic Sendai F/HN viroplexes.
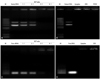
Fig. 2
Viroplex-mediated luciferase transfection of Jurkat and CEM cells. Lipofectamine 2000 lipoplexes, DMKE lipoplexes, CSVs, or PCSVs containing pAAV-CMV-Luc or pEGFP (1 µg pDNA per well, 3 : 1 N/P ratio, 1 µg F/HN proteins) were added to Jurkat and CEM cells in a 24-well plate (each 1×106/well). After 4 h of transfection, cells were incubated for 24 h or 48 h, and luciferase expression in Jurkat (A) and CEM (B) cells was assayed. GFP expression in Jurkat (C) and CEM (D) cells was also assayed. Statistical analysis was done by Student's t test; *p<0.001 versus expression by DMKE lipoplexes, †p<0.001 versus expression by CSVs. RLU, relative light units; CSV, cationic Sendai F/HN viroplex; PCSV, protamine sulfate-condensed cationic Sendai F/HN viroplex; GFP, green fluorescence protein; pEGFP, plasmid encoding GFP; pAAV-CMV-Luc, plasmid encoding luciferase.
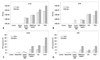
Fig. 3
Virosplex-mediated siRNA transfection of Jurkat and CEM cells. Jurkat (A) and CEM (B) cells were incubated with Lipofectamine 2000 lipoplexes, DMKE lipoplexes, or CSVs containing FITC-labeleled siRNA for 4 h or 24 h at 37℃. MFIs of transfected Jurkat (A) and CEM cells (B) were measured with flow cytometry. The same transfected Jurkat (C) and CEM (D) cells were stained with DAPI and then examined with a confocal laser microscope. Statistical analysis was done by Student's t test; *p<0.05 versus expression by DMKE lipoplexes. CSV, cationic Sendai F/HN viroplex; MFI, mean of fluorescence intensity.
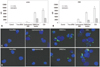
Fig. 4
Viroplex-mediated vimentin siRNA transfection of Jurkat and CEM cells. Jurkat and CEM cells were transfected with Lipofectamine 2000 lipoplexes, DMKE lipoplexes or CSVs of vimentin siRNA (200 pmole). For comparison, cells were transfected by electroporation with 6 pulses of 10 msec duration at 320 V/cm. At 24 h after transfection, the levels of vimentin mRNA (A) in the transfected cells were quantified by RT-PCR analysis and compared with that of GAPDH mRNA, a positive control (B). Statistical analysis was done by Student's t test; *p<0.05 versus expression by DMKE lipoplexes. CSV, cationic Sendai F/HN viroplex.
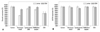
References
2. Gao Y, Liu XL, Li XR. Research progress on siRNA delivery with nonviral carriers. Int J Nanomedicine. 2011; 6:1017–1025.


3. Larsen HØ, Roug AS, Nielsen K, Søndergaard CS, Hokland P. Nonviral transfection of leukemic primary cells and cells lines by siRNA-a direct comparison between Nucleofection and Accell delivery. Exp Hematol. 2011; 39:1081–1089.


4. Bagai S, Puri A, Blumenthal R, Sarkar DP. Hemagglutinin-neuraminidase enhances F protein-mediated membrane fusion of reconstituted Sendai virus envelopes with cells. J Virol. 1993; 67:3312–3318.


5. Kaneda Y. Update on non-viral delivery methods for cancer therapy: possibilities of a drug delivery system with anticancer activities beyond delivery as a new therapeutic tool. Expert Opin Drug Deliv. 2010; 7:1079–1093.


6. Khoshnejad M, Young PR, Toth I, Minchin RF. Modified influenza virosomes: recent advances and potential in gene delivery. Curr Med Chem. 2007; 14:3152–3156.


7. de Jonge J, Holtrop M, Wilschut J, Huckriede A. Reconstituted influenza virus envelopes as an efficient carrier system for cellular delivery of small-interfering RNAs. Gene Ther. 2006; 13:400–411.


8. Lund PE, Hunt RC, Gottesman MM, Kimchi-Sarfaty C. Pseudovirions as vehicles for the delivery of siRNA. Pharm Res. 2010; 27:400–420.


9. Tu Y, Kim JS. A fusogenic segment of glycoprotein H from herpes simplex virus enhances transfection efficiency of cationic liposomes. J Gene Med. 2008; 10:646–654.


10. Kim HS, Kim JS, Lee YK, Koo KH, Park YS. An efficient liposomal gene delivery vehicle using Sendai F/HN proteins and protamine. Cancer Gene Ther. 2008; 15:214–224.


11. Zhang Q, Li Y, Shi Y, Zhang Y. HVJ envelope vector, a versatile delivery system: its development, application, and perspectives. Biochem Biophys Res Commun. 2008; 373:345–349.


12. Sanderson CM, Avalos R, Kundu A, Nayak DP. Interaction of Sendai viral F, HN, and M proteins with host cytoskeletal and lipid components in Sendai virus-infected BHK cells. Virology. 1995; 209:701–707.


13. Kim HS, Moon J, Kim KS, Choi MM, Lee JE, Heo Y, et al. Gene-transferring efficiencies of novel diamino cationic lipids with varied hydrocarbon chains. Bioconjug Chem. 2004; 15:1095–1101.


14. Kim HS, Song IH, Kim JC, Kim EJ, Jang DO, Park YS. In vitro and in vivo gene-transferring characteristics of novel cationic lipids, DMKD (O,O'-dimyristyl-N-lysyl aspartate) and DMKE (O,O'-dimyristyl-N-lysyl glutamate). J Control Release. 2006; 115:234–241.


15. Altenburg JD, Harvey KA, McCray S, Xu Z, Siddiqui RA. A novel 2,6-diisopropylphenyl-docosahexaenoamide conjugate induces apoptosis in T cell acute lymphoblastic leukemia cell lines. Biochem Biophys Res Commun. 2011; 411:427–432.


16. Cron RQ, Schubert LA, Lewis DB, Hughes CC. Consistent transient transfection of DNA into non-transformed human and murine T-lymphocytes. J Immunol Methods. 1997; 205:145–150.


17. Hawley-Nelson P, Ciccarone V, Moore ML. Transfection of cultured eukaryotic cells using cationic lipid reagents. Curr Protoc Mol Biol. 2008; Chapter 9:Unit 9.4.
18. Lee YK, Kim KS, Kim JS, Baek JE, Park SI, Jeong HY, et al. Leukemia-specific siRNA delivery by immunonanoplexes consisting of anti-JL1 minibody conjugated to oligo-9 Arg-peptides. Mol Cells. 2010; 29:457–462.


19. Fyrberg A, Lotfi K. Optimization and evaluation of electroporation delivery of siRNA in the human leukemic CEM cell line. Cytotechnology. 2010; 62:497–507.


20. Huckriede A, De Jonge J, Holtrop M, Wilschut J. Cellular delivery of siRNA mediated by fusion-active virosomes. J Liposome Res. 2007; 17:39–47.


21. Sorgi FL, Bhattacharya S, Huang L. Protamine sulfate enhances lipid-mediated gene transfer. Gene Ther. 1997; 4:961–968.


22. Hogrefe RI, Lebedev AV, Zon G, Pirollo KF, Rait A, Zhou Q, et al. Chemically modified short interfering hybrids (siHYBRIDS): nanoimmunoliposome delivery in vitro and in vivo for RNAi of HER-2. Nucleosides Nucleotides Nucleic Acids. 2006; 25:889–907.


23. Bramsen JB, Kjems J. Chemical modification of small interfering RNA. Methods Mol Biol. 2011; 721:77–103.

