Abstract
Purpose
Diabetes is the leading cause of end-stage renal failure. The present study was undertaken to characterize the effects of Corni Fructus on diabetic nephropathy in streptozotocin-induced diabetic rats and their mechanisms.
Materials and Methods
Streptozotocin-diabetic rats were orally administrated with Corni Fructus at a dose of 100, 200 or 400 mg/kg body mass for 40 days.
Results
Corni Fructus-treated diabetic rats showed significant decreases of blood glucose, urinary protein levels and water consumption. Corni Fructus also reduced serum total cholesterol, total triglyceride and low-density lipoprotein cholesterol levels, and showed a tendency of enhancing high-density lipoprotein cholesterol level. Levels of serum albumin and creatinine in diabetic rats were also significantly reduced by Corni Fructus administration at a dose of 200 and 400 mg/kg body mass compared with non-treated diabetic rats. Corni Fructus increased catalase (CAT), superoxide dismutase (SOD) and glutathione peroxidose (GSH-px) activities in the kidneys of diabetic rats. Furthermore, Corni Fructus treatment enhanced renal peroxisome proliferator-activated receptor-γ (PPARγ) expression in diabetic rats.
Diabetes mellitus, a common metabolic disease characterized by elevated blood glucose, has been linked to diabetic complications such as hyperlipidemia and retinopathy. In particular, there is an increased risk of diabetes in several types of kidney disease, and the predominant cause of end-stage renal disease in this disorder is diabetic nephropathy.1 Furthermore, there is considerable evidence to indicate that oxidative stress plays a role in the pathogenesis of diabetic nephropathy.2 Oxygen free radicals exert their cytotoxic effects on membrane phospholipids resulting in the formation of malondialdehyde (MDA). In addition, diabetes is usually accompanied with an increase in lipid peroxidation, alteration of the glutathione redox state, a decrease in the content of individual natural antioxidants, and decreased induction of antioxidant enzymes.3
Peroxisome proliferator-activated receptor-γ (PPARγ) is a member of the nuclear hormone receptor superfamily of ligand-activated transcription factors. It plays important roles in maintaining glucose, insulin sensitivity, lipid homeostasis and cell cycle regulation.4 PPARγ agonist ligands can attenuate the expression or activity of nicotinamide adenine dinucleotide oxidase, which is now considered to be a key pathogenic mediator of injury in the diabetic kidney. Therefore, PPARγ activators are used widely in the treatment of type 2 diabetes, and they improve the sensitivity of insulin receptors. Stimulation of PPARγ may trigger its association with the corepressor NCoR, thereby preventing dissociation of NCoR from the iNOS promoter and subsequent gene action.5 Troglitazone was the first PPARγ agonist to be used in the clinic. However, this compound was withdrawn because marked hepatotoxicity adverse effects were observed. Rosiglitazone, another PPARγ agonist, increases the risk of bone fractures, weight gain and edema.6 Modern drugs, including insulin and other oral hypoglycemic agents such as biguanides, sulphonylureas and alpha-glucosidase inhibitors, can control blood glucose level as long as they are administered regularly, however, they may also produce a number of undesirable side-effects.7
All of these observations have raised an enthusiasm in the development of new PPARγ agonists devoid of side-effects. At present, medical plants play a major role in the introduction of new therapeutic agents and have received much attention as the sources of biologically active substances because of its nontoxic and non side-effect properties.8,9 Corni Fructus (Cornus officinalis SIEB. et Zucc.) has attracted much interest due to its various biological activities, including immunological regulation, blood glucose reduction and antimicrobial activity.10-12 We earlier optimized the extraction method for Corni Fructus and evaluated its antidiabetic properties in alloxan-induced diabetic mice.13 In the present study, we investigated the effects of Corni Fructus on antioxidation, PPARγ expression and renal function in streptozotocin-induced diabetic rats in order to study its renal protective effects and hypoglycemic mechanisms.
Macroporous resin was purchased from Nan Kai University Chemical Co., ursolic acid standards from Yousi Biotechnology Inc. (Shanghai, China), streptozotocin from Sigma Chemicals Co. (St. Louis, MO, USA), glucose analyzer (GT-1640) and strips from Arkray Inc. (Kyoto, Japan), and Corni Fructus from Chang Hai Traditional Chinese Medicine Ltd. (Qinhuangdao, China). The identity of Corni Fructus was verified by a botanist.
Powdered Corni Fructus was initially soaked in 10 volumes of 70% ethanol for 2 hours and then extracted with ultrasound for 30 minutes. The supernatant was concentrated by a rotary evaporator and vacuum dried. The powdered extract was resuspended in water and resolves by a column chromatography, using macroporous resin (AB-8) column successively eluted with dH2O, 50%, 75% and anhydrous ethanol. The anhydrous ethanol phase was collected, dried, and then examined by thin-layer chromatogram (TLC) analysis.
Male Wistar rats, weighing 220-240 g, were provided by the Zoology Department of the Beijing Institute of Traditional Medical and Pharmaceutical Sciences. The rats were fed a standard rat chow diet and had free access to water. Experimental diabetes was induced by a single intraperitoneal injection of streptozotocin at a dose of 60 mg/kg body mass (using a freshly prepared solution in 0.1 M citrate buffer, pH 4.5) to the animals who fasted overnight while control rats were injected with citrate buffer alone. Blood samples were obtained from the tail vein after an overnight fast at 72 hour post-injection. Rats with fasting blood glucose levels in the range of 16.7 to 25 mM were defined as diabetic model rats.14 All animal procedures were performed according to the National Institutes of Health Guide for the Care and Use of Laboratory Animals.
Group I consisted of 8 healthy rats and received 0.5% carboxymethylcellulose (CMC) solution. Streptozotocin-induced diabetic rats were divided into four groups of 8 animals each (Groups II-V). Group II served as a diabetic control and received 0.5% CMC solution, while Groups III, IV, and V received 100, 200 and 400 mg/kg body mass of Corni Fructus extract dissolved in 0.5% CMC solution: the rats were administered intragastrically once daily for 40 days. Water intake and body weight were determined every 2 days, while blood glucose levels were measured at 10 day intervals using the glucose oxidase method to ascertain diabetic status of each group.15
On day 1 after overnight fasting with free access to water, the rats were administered with Corni Fructus extract dissolved in a solution of 0.5% CMC by oral gavage at the following doses: 100 mg/kg body mass (Group III), 200 mg/kg body mass (Group IV), and 400 mg/kg body mass (Group V), or with the same volume of 0.5% CMC solution alone (Groups I and II). Tail blood samples were drawn from each rat, and a glucose (1.0 g glucose/kg body mass) solution was given by oral gavage to all experimental rats 30 minutes after Corni Fructus administration. A baseline (0 minute) blood sample was taken from the tail vein in normal control and experimental rats, and the blood samples were then collected at 30, 60, 90, and 120 minute intervals following glucose administration. Blood plasma glucose levels were measured at various time points using the glucose oxidase method.
On day 40, blood samples were collected from the eyes of fasted experimental rats under ether anaesthesia, left at room temperature for 2 hours and then centrifuged at 1500×g for 15 minutes to prepare serum. Serum was used to determine the levels of total cholesterol (TC), total triglyceride (TG), low-density lipoprotein cholesterol (LDL-C) and high-density lipoprotein cholesterol (HDL-C) levels following the instructions of the manufacturer (Nanjing Jiancheng Bioengineering Co., Nanjing, China). Serum samples were also used for measuring creatinine and blood urea nitrogen (BUN). Twenty-four hour urinary protein concentrations were determined by the Bradford method, by using commercial kits (Nanjing Jiancheng biomedical engineering Co. Ltd.).
All animals were sacrificed on day 40 by cervical dislocation under ether anesthesia and kidney tissues of each animal were rapidly excised, weighed and washed twice with cold phosphate buffer solution (PBS). One quarter of the kidney tissue from each rat was weighed, homogenized with Tris-HCl (pH 7.4), and centrifuged (1500×g, 10 minutes, 4℃). Supernatants were used immediately to assay MDA, glutathione peroxidase (GSH-px), catalase (CAT) and superoxide dismutase (SOD) using commercial kits according to the manufacturer's instructions (Nanjing Jiancheng Bioengineering Co., Nanjing, China).
One quarter of the kidney tissues from each rat was homogenized in ice-cold lysis buffer containing 2% sodium dodecylsulfate (SDS), 100 mM proteinase cocktail inhibitor, 1 mM phenylmethylsulfonylfluoride, 1 mM dithiothreitol, and 5 mM ethylenediaminetetraacetic acid in 50 mM Tris-buffered saline (TBS, 50 mM Tris-HCl, pH 7.4). After centrifugation (12500×g, 5 minutes, 4℃), the supernatants were collected. Samples (100 µg protein) were boiled for 3 minutes and subjected to 10% SDS-PAGE. After electrophoresis, proteins were transferred to polyvinylidene difluoride (PVDF) membranes (invitrogen). The blots were incubated in blocking buffer (TBST, 5% skim milk in TBS containing 0.1% Tween-20) for 1 hour at room temperature and then probed overnight with a 1:1000 dilution of goat polyclonal anti-PPARγ (Santa Cruz, CA, USA) at 4℃. The blots were washed three times with TBST for 10 minutes and reacted with goat anti-rat horseradish peroxidase (HRP)-conjugated antibody (1:5000 dilution; Sigma, St. Louis, MO, USA) for 1 hour at room temperature. The immunoreactive bands were visualized using enhanced chemiluminescence (ECL Kit; Millipore, Billerica, MA, USA). The membranes were exposed to X-ray films, and band intensities were quantified using Scion Image.
Total mRNA from one quarter of the kidney tissues from each rat was extracted with Trizol reagent (invitrogen). Relative levels of specific mRNAs were assessed by reverse transcriptase polymerase chain reaction (RT-PCR). Single-stranded cDNA was synthesized from 1 µg of total RNA using avian myeloblastosis virus (AMV) reverse transcriptase in a total reaction volume of 20 µL (Promega, Madison, WI, USA). The reverse transcription program was 25℃ for 10 minutes, 42℃ for 50 minutes, and then 70℃ for 15 minutes. cDNA was synthesized with Taq DNA Polymerase (Promega, Madison, WI, USA). The genes examined were PPARγ (GenBank Accession No. NM 013124: 265 bp; forward primer: 5'-ACTCCCATTCCTTTGACATC-3', reverse primer: 5'-TCCCCACAGACTCGGCACTC-3')16, and β-actin (228 bp; sense primer: 5'-AGCCATGTACGTAGCCATCC-3' and antisense primer: 5'-CTCTCAGCTGTGGTGGTGAA-3'). The PCR cycle was 94℃ for 5 minutes, then 35 cycles at 94℃ for 50 seconds, 60℃ for 1 minute, and 72℃ for 1 minute, and finally 72℃ for 10 minutes. The PCR samples were electrophoresed on 1% agarose gels and gel images were analysed after staining with ethidium bromide. RT-PCR values are presented as the ratio of the specified gene signal in the selected linear amplification cycle divided by the β-actin signal.
One quarter of the kidney tissues from each rat were immersed in neutral buffered formalin solution, fixed with 10% neutral formalin phosphate buffer, dehydrated through a graded alcohol series, embedded in paraffin, cut into 4 µm sections and stained with periodic acid-schiff (PAS) stain. The sections were viewed with a Nikon light microscope by an experienced pathologist. Slides were evaluated under 400×magnification. After five slides of each specimen were photographed, the cross-sectional area and glomerular radius of each section were assessed. Glomerular volume (Gv) was calculated by the formula: Gv=4πr3/3.17 The mesangial expansion index was scored by quantitatively estimating the width of the mesangial zones in each glomerulus, and was expressed as a function of the total glomerular area18: 0, normal glomeruli; 1, matrix expansion occurred in up to 50% of the glomeruli; 2, matrix expansion occurred in 50-75% of the glomeruli; 3, matrix expansion occurred in 75-100% of the glomeroli. Each slide was scored by a pathologist who was unaware of the experimental details.
Statistical analyses were performed using the SPSS 13.0 software. The effects of Corni Fructus on OGTT and hypoglycemia were determined using two-way ANOVA with repeated measures, followed by the Turkey test. Differences in antioxidant enzymes and renal function parameters among diabetic controls and groups treated with different doses of Corni Fructus were analyzed using one-way ANOVA, followed by the Scheffe test. Results were considered significantly different at the level of p<0.05.
The extract was analyzed using TLC by comparing the color and retardation factor (Rf) value with ursolic acid standards. The sample turned purple and had the same Rf value as the ursolic acid standards, showing that the main active ingredient in the Corni Fructus extract prepared above was ursolic acid. The sample also contained a little organic acid and glycoside.
As shown in Table 1, the body weight of Group I increased consistently from 233.38 g to 325.63 g during the forty-day experiment, while the diabetic group showed a significant decrease in body weight from 233.38 g to 207.88 g. However, the body weight of Corni Fructus-treated groups increased significantly, especially in Groups IV and V, compared with that of the diabetic control group (p<0.01).
Oral glucose tolerance tests were used to determine the acute effect of Corni Fructus on diabetic rats. Corni Fructus supplementation improved glucose tolerance (Fig. 1). Plasma glucose concentration peaked at 30 minutes after oral glucose loading and then decreased to baseline by 60 minutes in normal rats. However, plasma glucose concentrations were still higher in diabetic control rats at 120 minutes after glucose ingestion. In Corni Fructus-treated groups, blood glucose levels peaked at 30 minutes and promptly decreased to the fasting level by 60 minutes, and blood glucose levels were lower than that of the fasting level at 2 hours (Group IV and V). It is obvious that Corni Fructus administration prevented drastic increases of blood glucose after glucose loading, and also decreased the glucose levels significantly in a concentration-dependent manner. The differences between Corni Fructus-treated groups and the diabetic control group at 120 minutes were statistically significant.
In the forty-day glucose test, all rats were treated as above for 40 days, and blood glucose levels were monitored at 10 days intervals (Fig. 2). They consistently remained at similar levels in both the normal and diabetic control groups during the experiment, i.e., from day 0 to day 40. However, significantly lower blood glucose levels were observed in Corni Fructus-treated groups (p<0.05 or 0.01). Blood glucose levels, however, did not return to normal levels in Corni Fructus-treated groups. Blood glucose decreased in Groups III, IV, and V by 20.98, 48.39 and 58.34%, respectively. These results indicate that Corni Fructus induced a more pronounced hypoglycemic effect in the streptocotocin-induced diabetic rats in a dose-dependent manner.
The effect of Corni Fructus treatment on serum lipids of tested groups is given in Table 2. The levels of TG, TC and LDL-C in Group II were 1.228±0.025, 2.033±0.033 and 0.749±0.023 mM, respectively, and were significantly higher (p<0.05, 0.01) than those of normal rats. When diabetic rats were treated with Corni Fructus for 40 days, TG, TC and LDL-C serum levels were reduced (p<0.05, 0.01) compared to diabetic control rats. The Corni Fructus-treated groups also had a significantly higher HDL-C level than diabetic control rats (p<0.05), which had HDL-C levels close to that of normal rats.
During the study period, the levels of serum creatinine, urine protein and BUN in STZ-diabetic rats were significantly higher than those of normal rats (Table 3), while those in STZ-diabetic rats receiving Corni Fructus treatment (40 days) tended to be reduced; at the termination of 40-day treatment, the values for serum creatinine, urine protein and BUN in Groups IV and V were significantly lower than those of diabetic rats (p<0.01). In addition, the water consumption of normal rats was stable during the experimental period, but was significantly increased in diabetic control rats than in normal rats. Water consumption in Corni Fructus-treated groups (Groups III, IV, and V) decreased during the experimental period. On the fortieth day, the water consumption of these Corni Fructus-treated groups was approximately half of that of the diabetic control group.
The mean ratio of kidney weight to body weight in STZ-induced diabetic rats increased significantly compared to that of the normal group (p<0.01). Corni Fructus-treated diabetic rats (Group III) had slightly reduced renal hypertrophy at the termination of the 40-day treatment, and there was a marked reduction in the above parameters in STZ-diabetic rats after Corni Fructus treatment (Groups IV and V) (Table 3).
In PAS-stained images of glomeruli at the end of the 40-day treatment period (Fig. 3), there was significant glomerular hypertrophy and expansion of the mesangial area in STZ-diabetic rats compared with normal rats. The glomerular hypertrophy and mesangial matrix accumulation induced by STZ were significantly suppressed by 40 days of Corni Fructus treatment (Group IV and V) compared to the diabetic control group. Furthermore, morphometric analysis indicated that the Corni Fructus treatment (Group V) significantly inhibited the increase of glomerular volume (Fig. 3F) and mesangial expansion index compared with vehicle treatment (Fig. 3G), indicating that the intake of Corni Fructus slowed down functional and pathological deterioration of diabetic nephropathy in these rats.
The levels of SOD, GSH-px, CAT and MDA in normal, diabetic and Corni Fructus-treated rats are shown in Table 4. In STZ-induced diabetic rats, kidney SOD, GSH-px and CAT levels decreased significantly by 13%, 25%, and 33%, respectively. MDA increased by 31% in the kidney of diabetic rats. Corni Fructus at 100 mg/kg body mass did not have a significant effect on SOD, CAT and GSH-px activities in the kidney of STZ-diabetic rats (p>0.05). However, activities of SOD, CAT and GSH-px in Corni Fructus-treated diabetic rats (200 and 400 mg/kg body mass) were significantly increased (p<0.05, 0.01). Furthermore, Corni Fructus at a dose of 100 mg/kg body mass did not significantly decrease STZ-induced increases in MDA level in the rat kidney. MDA levels in the kidneys of Groups IV and V (200 and 400 mg/kg body mass) were decreased significantly by 14% and 22%, respectively. Corni Fructus treatment, therefore, significantly blocked the increase of MDA and was associated with a partial elevation of kidney total antioxidant ability, including SOD, CAT and GSH-px activities.
The protein was isolated from one quarter of the kidney tissues of treated rats and expression levels of PPARγ were determined by Western blotting. PPARγ expression levels in normal, diabetic and Corni Fructus-treated rats are shown in Fig. 4. STZ-induced diabetic rats, PPARγ levels decreased significantly by 43.94%. Expression levels of PPARγ in Corni Fructus-treated diabetic rats (100, 200 and 400 mg/kg body mass) were higher compared with diabetic control rats (p<0.01), indicating that Corni Fructus treatment significantly stimulated the expression of PPARγ in diabetic rats.
Total RNA was isolated from the kidney tissues of Groups I to V rats, and semi-quantitative RT-PCR was carried out to investigate the expression levels of PPARγ. PPARγ expression levels in normal, diabetic and Corni Fructus-treated rats are shown in Fig. 5. In STZ-induced diabetic rats, PPARγ levels decreased significantly by 43.3%, whereas the expression levels of PPARγ in Corni Fructus-treated diabetic rats (100, 200 and 400 mg/kg) were higher compared with diabetic control rats (p<0.01). These results also show that the Corni Fructus treatment significantly stimulated the expression of PPARγ in diabetic rats.
Diabetic nephropathy is the most serious complication in diabetes mellitus, and one of the most important microvascular complications of diabetic patients. It has been suggested to result from interactions between metabolic and hemodynamic factors involved with hyperglycemia. Currently available drugs for diabetes have a number of limitations, such as adverse effects and high rates of secondary failure.19,20 This situation has led to the search for alternative therapies from natural products that have low or no side effects and multi-target actions.21 Even the WHO expert committee on diabetes has recommended that this area warrants further attention.22 In the present study, the main compound of Corni Fructus was shown to be ursolic acid after ultrasonic extraction and purification using macroporous resins, and pharmacological intervention with Corni Fructus (200 and 400 mg/kg body mass) significantly relieved hyperglycemia, hyperlipidemia, and diabetic nephropathy in STZ-induced diabetic rats. OGTT data indicated that the postprandial blood glucose was markedly decreased after treatment with Corni Fructus suggesting that Corni Fructus can improve the sensitivity or stimulate secretion of insulin in the STZ-induced diabetic rats. Nevertheless, further research is needed.
Diabetes is a metabolic disorder affecting carbohydrate, fat and protein metabolism, and is complicated by multiorgan deterioration in the latter stages of the illness. Urinary albumin excretion has been shown to be a good clinical predictor of renal lesions in diabetic nephropathy.23,24 The increase in urinary albumin excretion, one of the most marked renal pathological findings in diabetic nephropathy results from mesangial expansion, due to pathological accumulation of extra-cellular matrix components.25 In the present study, increase in urinary albumin concentration corresponding to the hyperglycemia was more pronounced following induction of diabetes. In addition, levels of serum creatinine and BUN, which are generally considered as markers of renal function, were higher in STZ-diabetic rats than in normal rats, indicating the presence of diabetic nephropathy with renal hyperfiltration. Forty-day treatment with Corni Fructus attenuated albuminuria and ameliorated the loss of renal function and glomerular hyperfiltration in STZ-diabetic rats. Increased kidney weight and accelerated mesangial expansion in the glomeruli of STZ-diabetic rats were alleviated by 40 days of Corni Fructus treatment. These results demonstrate that Corni Fructus has potent effects on the deterioration of renal expansion and hyperfiltration by reducing renal extra-cellular matrix accumulation in STZ-diabetic rats, possibly leading to the amelioration or delay in the development of advanced diabetic renal injury.
Streptozotocin-induced diabetes is characterized by a severe loss in body weight,26 which might be the result of protein wasting due to the unavailability of carbohydrate as an energy source.27 In our study, oral administration of Corni Fructus reduced the decline in body weight in diabetic rats. In addition, we observed significant increases in the levels of TC, TG and LDL-C in diabetic rats, and the 40-day Corni Fructus treatment not only lowered the levels of TC, TG and LDL-C, but also enhanced the levels of the cardioprotective lipid HDL-C. It is likely that the hypolipidemic effect of Corni Fructus results from the inhibition of cellular cholesterol and triglyceride synthesis, thus suggesting that Corni Fructus may be beneficial to diabetic individuals with hyperlipidemia.
Several studies have documented that oxidative stress is accelerated in diabetes mellitus owing to an increase in the production of oxygen free radicals, lipid peroxidation and low-density lipoprotein.28,29 Free radicals can diffuse intracelluarly and result in mitochondrial enzyme damage and DNA breaks, all of which impair cellular function and contribute to the pathophysiology of diabetes.30,31 Oxygen free radicals exert their cytotoxic effects on membrane phospholipids, resulting in the formation of MDA. As a product of lipid peroxidation, the levels of MDA reflect the degree of oxidation in the body. As components of the free radical scavenging system, SOD, CAT and GSH-px exist in all oxygen-metabolizing cells, prevent damage to cells by free radicals and provide a repair mechanism for oxidized membrane components. In the present study, diabetic nephropathy was significantly improved in rats receiving Corni Fructus. Furthermore, we observed a significant increase in SOD, CAT and GSH-px activities in Corni Fructus-treated diabetic groups compared with the untreated diabetic control group, and the treatment of Corni Fructus also decreased the level of MDA in the diabetic rats. The increases in antioxidases provide effective protection from oxidative damage.
It is worth noting that chronic diabetic nephropathy reduces the PPARγ mRNA levels in glomeruli.32 PPARγ is a master transcriptional regulator in lipid and glucose metabolism, and induces lipogenic gene transcription and dipocyte differentiation.33-35 Also, many animal and clinical experiments demonstrated that upregulation or activation of PPARγ can normalize lipid and glucose metabolism disorders, enhance insulin sensitivity of peripheral organs by lipoprotein lipase hydrolyzing TG, and deliver fatty acids to the tissue for storage or oxidation.36,37 Moreover, rosiglitazone has been shown in a recent study to prevent the development of diabetic nephropathy by reducing podocyte loss, downregulating the expression of glomerular fibronectin and inhibiting ROS accumulation in the glomerules of diabetic mice with nephropathy,38 and PPARγ agonists have a protective role against oxidative stress.39 The effects of PPARγ are mediated by the modulation of ROS generation and activation of redox-sensitive transcription factor nuclear factor-kB and hypoxia inducible factor (HIF-1a) in allergic airway disease of mice.40 However, the effects of PPARγ on antioxidative stress in STZ-induced diabetic rats with hyperlipidemia have not yet been clarified. In the present study, glucose and lipid in the plasma were normalized by activating PPARγ in Corni Fructus-treated diabetic rats, showing that activation of PPARγ modulated diabetes-induced kidney damage. Corni Fructus also increased antioxidant enzyme activities in kidney tissues and reduced oxidative damage and glomerulosclerosis through upregulation PPARγ expression. Therefore, Corni Fructus may be a PPARγ agonist to ameliorate abnormal lipid and glucose metabolism.
In conclusion, our present study reveals that Corni Fructus has beneficial effects inimproving blood glucose and lipid levels in diabetic rats, and prevents diabetic nephropathy. This study provides experimental evidence that Corni Fructus may be a potential therapeutic agent for hyperglycemia that is associated with diabetic complications including diabetic nephropathy.
Figures and Tables
Fig. 1
Effects of oral glucose tolerance test on Corni Fructus-treated diabetic rats. The values are means±SE of eight rats per group. *p<0.05 vs. the diabetic control group (Group II).
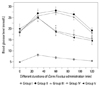
Fig. 2
Effect of Corni Fructus treatment on blood glucose levels in diabetic rats. The values are means±SE of eight rats per group. *p<0.05 vs. the diabetic control group (Group II). †p<0.01 vs. the diabetic control group (Group II).
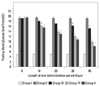
Fig. 3
Representative photomicrographs (original magnification, ×400) of PAS stained kidney sections. (A) Group I (B) Group II (C) Group III (D) Group IV (E) Group V rats (F) Glomerular volume, and (G) expansion of matrix index expressed as a quantitative estimate score. All values are means±SD. *p<0.05, compared with STZ-treated group, †p<0.05, compared with STZ-treated group. PAS, periodic acid-schiff; STZ, streptozotocin; SD, standard deviation.

Fig. 4
Levels of PPARγ in kidney tissues of experimental rats. Western blotting analysis shows the expression levels of PPARγ in kidney tissues. The values are means±SD of eight rats per group. *p<0.01 vs. the diabetic control group (Group II). PPAR, peroxisome proliferator-activated receptor; SD, standard deviation.
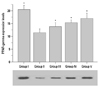
Fig. 5
PPARγ transcription level in the kidney tissues of experimental rats. RT-PCR analysis shows the expression levels of PPARγ (top panel), and β-actin (bottom panel) in kidney tissues. The values are means±SD of eight rats per group; *p<0.01 vs the diabetic control group (Group II). PPAR, peroxisome proliferator-activated receptor; RT-PCR, reverse transcriptase polymerase chain reaction; SD, standard deviation.
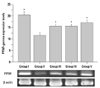
ACKNOWLEDGEMENTS
This work was financially supported by the research grant from the Chinese Ministry of Education Doctor Degree (No. 20101333120011), a grant from Hebei Province Natural Science Fund (No. C2011203137, 11965152D), and a Chinese Postdoctoral grant (480013).
References
1. Cooper ME, Allen TJ, O'Brien RC, Macmillan PA, Clarke B, Jerums G, et al. Effects of genetic hypertension on diabetic nephropathy in the rat--functional and structural characteristics. J Hypertens. 1988. 6:1009–1016.


2. Prabhakar S, Starnes J, Shi S, Lonis B, Tran R. Diabetic nephropathy is associated with oxidative stress and decreased renal nitric oxide production. J Am Soc Nephrol. 2007. 18:2945–2952.


3. Ceriello A, Bortolotti N, Falleti E, Taboga C, Tonutti L, Crescentini A, et al. Total radical-trapping antioxidant parameter in NIDDM patients. Diabetes Care. 1997. 20:194–197.


4. Yang HC, Ma LJ, Ma J, Fogo AB. Peroxisome proliferator-activated receptor-gamma agonist is protective in podocyte injury-associated sclerosis. Kidney Int. 2006. 69:1756–1764.


5. Pascual G, Fong AL, Ogawa S, Gamliel A, Li AC, Perissi V, et al. A SUMOylation-dependent pathway mediates transrepression of inflammatory response genes by PPAR-gamma. Nature. 2005. 437:759–763.


6. Rubenstrunk A, Hanf R, Hum DW, Fruchart JC, Staels B. Safety issues and prospects for future generations of PPAR modulators. Biochim Biophys Acta. 2007. 1771:1065–1081.


7. Kuroe A, Taniuguchi A, Fukushima M, Nakai Y, Ohgushi M, Ohya M, et al. Early and late onset side effects of short-acting insulin analogue in seven Japanese diabetic patients. Diabetes Res Clin Pract. 2007. 77:412–413.


8. Shoji Y, Nakashima H. Glucose-lowering effect of powder formulation of African black tea extract in KK-A(y)/TaJcl diabetic mouse. Arch Pharm Res. 2006. 29:786–794.


9. Kochhar A, Nagi M. Effect of supplementation of traditional medicinal plants on blood glucose in non-insulin-dependent diabetics: a pilot study. J Med Food. 2005. 8:545–549.


10. Qian DS, Zhu YF, Zhu Q. [Effect of alcohol extract of Cornus officinalis Sieb. et Zucc on GLUT4 expression in skeletal muscle in type 2 (non-insulin-dependent) diabetic mellitus rats]. Zhongguo Zhong Yao Za Zhi. 2001. 26:859–862.
11. Wu VC, Qiu X, Peggy Hsieh YH. Evaluation of Escherichia coli O157:H7 in apple juice with Cornus fruit (Cornus officinalis Sieb. et Zucc.) extract by conventional media and thin agar layer method. Food Microbiol. 2008. 25:190–195.


12. Peng Q, Wei Z, Lau BH. Fructus corni attenuates oxidative stress in macrophages and endothelial cells. Am J Chin Med. 1998. 26:291–300.


13. Gao D, Li N, Li Q, Li J, Han Z, Fan Y, et al. Study of the extraction, purification and antidiabetic potential of ursolic acid from Cornus officinalis Sieb. et Zucc. Therapy. 2008. 5:697–705.


14. Gao D, Li Q, Li Y, Liu Z, Liu Z, Fan Y, et al. Antidiabetic potential of oleanolic acid from Ligustrum lucidum Ait. Can J Physiol Pharmacol. 2007. 85:1076–1083.
15. Trinder P. Determination of blood glucose using an oxidase-peroxidase system with a non-carcinogenic chromogen. J Clin Pathol. 1969. 22:158–161.


16. Asami-Miyagishi R, Iseki S, Usui M, Uchida K, Kubo H, Morita I. Expression and function of PPARgamma in rat placental development. Biochem Biophys Res Commun. 2004. 315:497–501.
17. van Bamme B, Koudstaal J. Measuring glomerular diameters in tissue sections. Virchows Arch A Pathol Anat Histol. 1976. 369:283–291.


18. Liu IM, Tzeng TF, Liou SS, Chang CJ. The amelioration of streptozotocin diabetes-induced renal damage by Wu-Ling-San (Hoelen Five Herb Formula), a traditional Chinese prescription. J Ethnopharmacol. 2009. 124:211–218.


19. Shapiro K, Gong WC. Natural products used for diabetes. J Am Pharm Assoc (Wash). 2002. 42:217–226.


20. Ajjan RA, Grant PJ. Cardiovascular disease prevention in patients with type 2 diabetes: the role of oral anti-diabetic agents. Diab Vasc Dis Res. 2006. 3:147–158.


21. Ozsoy-Sacan O, Karabulut-Bulan O, Bolkent S, Yanardag R, Ozgey Y. Effects of chard (Beta vulgaris L. var cicla) on the liver of the diabetic rats: a morphological and biochemical study. Biosci Biotechnol Biochem. 2004. 68:1640–1648.


22. Technical report series 646. WHO Expert Committee on Diabetes Mellitus: Second Report. 1980. Geneva, Switzerland: World Health Organization;18.
23. Parving HH, Lehnert H, Bröchner-Mortensen J, Gomis R, Andersen S, Arner P. Irbesartan in Patients with Type 2 Diabetes and Microalbuminuria Study Group. The effect of irbesartan on the development of diabetic nephropathy in patients with type 2 diabetes. N Engl J Med. 2001. 345:870–878.


24. Ahn CW, Song YD, Kim JH, Lim SK, Choi KH, Kim KR, et al. The validity of random urine specimen albumin measurement as a screening test for diabetic nephropathy. Yonsei Med J. 1999. 40:40–45.


25. Falk RJ, Scheinman JI, Mauer SM, Michael AF. Polyantigenic expansion of basement membrane constituents in diabetic nephropathy. Diabetes. 1983. 32:Suppl 2. 34–39.


26. Soria B, Roche E, Berná G, León-Quinto T, Reig JA, Martín F. Insulin-secreting cells derived from embryonic stem cells normalize glycemia in streptozotocin-induced diabetic mice. Diabetes. 2000. 49:157–162.


27. Chen V, Ianuzzo CD. Dosage effect of streptozotocin on rat tissue enzyme activities and glycogen concentration. Can J Physiol Pharmacol. 1982. 60:1251–1256.


28. Rabinovitch A, Suarez WL, Thomas PD, Strynadka K, Simpson I. Cytotoxic effects of cytokines on rat islets: evidence for involvement of free radicals and lipid peroxidation. Diabetologia. 1992. 35:409–413.


29. Reddy SV, Tiwari AK, Kumar US, Rao RJ, Rao JM. Free radical scavenging, enzyme inhibitory constituents from antidiabetic Ayurvedic medicinal plant Hydnocarpus wightiana Blume. Phytother Res. 2005. 19:277–281.


30. Hunt JV, Smith CC, Wolff SP. Autoxidative glycosylation and possible involvement of peroxides and free radicals in LDL modification by glucose. Diabetes. 1990. 39:1420–1424.


31. Bonnefont-Rousselot D, Bastard JP, Jaudon MC, Delattre J. Consequences of the diabetic status on the oxidant/antioxidant balance. Diabetes Metab. 2000. 26:163–176.
32. Zheng F, Fornoni A, Elliot SJ, Guan Y, Breyer MD, Striker LJ, et al. Upregulation of type I collagen by TGF-beta in mesangial cells is blocked by PPARgamma activation. Am J Physiol Renal Physiol. 2002. 282:F639–F648.
34. Hamm JK, el Jack AK, Pilch PF, Farmer SR. Role of PPAR gamma in regulating adipocyte differentiation and insulin-responsive glucose uptake. Ann N Y Acad Sci. 1999. 892:134–145.


35. Tontonoz P, Hu E, Spiegelman BM. Stimulation of adipogenesis in fibroblasts by PPAR gamma 2, a lipid-activated transcription factor. Cell. 1994. 79:1147–1156.


36. Iwata M, Haruta T, Usui I, Takata Y, Takano A, Uno T, et al. Pioglitazone ameliorates tumor necrosis factor-alpha-induced insulin resistance by a mechanism independent of adipogenic activity of peroxisome proliferator--activated receptor-gamma. Diabetes. 2001. 50:1083–1092.


37. Li D, Wang X, Ren W, Ren J, Lan X, Wang F, et al. High expression of liver histone deacetylase 3 contributes to high-fat-diet-induced metabolic syndrome by suppressing the PPAR-γ and LXR-α-pathways in E3 rats. Mol Cell Endocrinol. 2011. 344:69–80.


38. Zhang H, Saha J, Byun J, Schin M, Lorenz M, Kennedy RT, et al. Rosiglitazone reduces renal and plasma markers of oxidative injury and reverses urinary metabolite abnormalities in the amelioration of diabetic nephropathy. Am J Physiol Renal Physiol. 2008. 295:F1071–F1081.


39. Rose M, Balakumar P, Singh M. Ameliorative effect of combination of fenofibrate and rosiglitazone in pressure overload-induced cardiac hypertrophy in rats. Pharmacology. 2007. 80:177–184.


40. Lee KS, Kim SR, Park SJ, Park HS, Min KH, Jin SM, et al. Peroxisome proliferator activated receptor-gamma modulates reactive oxygen species generation and activation of nuclear factor-kappaB and hypoxia-inducible factor 1alpha in allergic airway disease of mice. J Allergy Clin Immunol. 2006. 118:120–127.

