Abstract
Purpose
To examine the effects of change in weight bearing on the growth plate metabolism, a simulated animal model of weightlessness was introduced and the chondrocytes' cellular kinetics was evaluated.
Materials and Methods
Unloading condition on the hind-limb of Sprague-Dawley rats was created by fixing a tail and lifting the hind-limb. Six rats aged 6 weeks old were assigned to each group of unloading, reloading, and control groups of unloading or reloading. Unloading was maintained for three weeks, and then reloading was applied for another one week thereafter. Histomorphometry for the assessment of vertical length of the growth plate, 5-bromo-2'-deoxyuridin immunohistochemistry for cellular kinetics, and biotin nick end labeling transferase-mediated deoxyuridine triphosphate-biotin nick end labeling (TUNEL) assay for chondrocytes apoptosis in the growth plate were performed.
Results
The vertical length of the growth plate and the proliferative potential of chondrocytes were decreased in the unloading group compared to those of control groups. Inter-group differences were more significant in the proliferative and hypertrophic zones. Reloading increased the length of growth plate and proliferative potential of chondrocytes. However, apoptotic changes in the growth plate were not affected by the alterations of weight bearing.
Conclusion
Alterations in the weight bearing induced changes in the chondrocytic proliferative potential of the growth plate, however, had no effects on the apoptosis. This may explain why non-weight bearing in various clinical situations hampers normal longitudinal bone growth. Further studies on the factors for reversibility of chondrocytic proliferation upon variable mechanical stresses are needed.
Muscles and skeleton play a role in combination, and maintain the body balance and induce movement. For example, the anti-gravity muscles in the lower extremities continuously perform contraction activity to maintain the posture, and skeleton plays a role of the lever that transmits muscle actions. On the other hand, in the cases when weight bearing is reduced or under insufficient condition, the body no longer receives normal weight bearing stress, and atrophy and weakness phenomena are developed by muscle contracted protein and bone matrix loss. Furthermore, reduction of number of osteoblasts, reduction of osteoid volume of cortical bone and trabecular bone, and decrease of bone density by abnormal formation of minerals in the cortical bone, etc. are detected.1-5
Changes occurring in the skeletal system are a frequently developed pathological phenomenon in the cases which require long bed rest due to trauma, or various internal or surgical diseases, in disabled individuals who depend on a wheel chair in person with autonomic imbalance, or in the elderly with reduced activity. If exercise were performed to overcome this, it is difficult to withstand the loading delivered suddenly, and ensuring complications and impairment are very severe. Previous studies on the changes of the skeletal system are biased on the observation of osteoporosis caused by the lack of weight bearing or the change of bone associated with bone loss.6,7 In the case of animal experiments, since the periosteal bone formation is shown to be substantially faster than humans, it is extremely difficult to interpret the results and to apply clinically. In addition, the remodeling of bone is controlled not only by the intramembranous bone formation but also by the endochondral bone formation. It is, therefore, essential to observe the pattern of the change of cell activity in the growth plate according to the change of weight bearing.
In our present study, the change of activity of chondrocytes in the growth plate of rats by the change of weight bearing was examined by the analysis of histomorphometric measurement and cellular kinetics.
As a lower-limb suspension method, the method of immobilizing
the back and the tail of Sprague-Dawley rats was used by improving the model of Nyhan, et al.3 To create the unloading state by lifting the lower extremities of rats, tygon tubing was fixed to the dorsal skin, and subsequently, the tail was immobilized using a tape. The tubing connected to the rat was connected to the restraint equipment of the lower-limb suspension equipment, and the angle was always maintained constant. The animals were thus free to move in a 360° arc. The rats were suspended with 30° head-down tilt in order to initiate a fluid shift similar to that experienced during space flight. This method of suspension resulted in a total loss of weight-bearing function in the hind limbs. The fore limbs were in contact with the plastic grid floor of the model, but some loss of weightbearing might have occurred. The suspended rats could eat food and drink water (Fig. 1).
For each group, 6 weeks old 6 Sprague-Dawley rats (weighing around 100 g) were used. By obtaining unloading and reloading conditions by the use of the lower limb suspension method, the change of weight bearing and redistribution of body fluid were induced. Animals were divided to the experiment groups experiencing the lower limb suspension for 3 weeks (unloading group, 9 weeks old) and reloading for 1 week after the lower limb suspension (reloading group, 10 weeks old), and the corresponding control group (age-matched 2 control groups; 9 weeks & 10 weeks old, respectively).
Animals were sacrificed under ether inhalation anesthesia, and the knee joint area containing the proximal tibia was extracted by performing osteotomy on the tibia and the femoral neck area. The extracted tissues were fixed with 4% paraformaldehyde, decalcified for 2 weeks with 10% ethylenediaminetetracetic acid, and embedded in paraffin by a conventional method. Subsequently, the samples were sectioned as 4 µm in thickness, fixed using silane coating slides, stained with hematoxylin and eosin, and examined under a light microscope.
In the unloading and reloading group as well as their control group, the lengths of the entire growth plate, the resting zone, the proliferation zone and the hypertrophic zone were measured using the ImagePro® program.8 Regarding all histological examination, four areas were selected randomly, and the entire length as well as the length of each zone were measured vertically.
5-bromo-2'-deoxyuridin (BrdU) injected peritoneally (100 mg/kg) twice, 25 hours and 1 hour prior to the sacrifice of experiment animals. The slides prepared by a conventional method were treated with xylen for 10 minutes 3 times, and for rehydration, the samples were washed with 100, 90, 70% ethanol and double distilled water sequentially. Endogenous tissue peroxidase was inactivated with 3% hydrogen peroxide (H2O2), and the tissues were reacted with 0.4% pepsin for 20 minutes. The samples were denatured with 2N HCl for 30 minutes, reacted with goat serum diluted to 20%, and reacted with BrdU primary antibody (SIGMA, St. Louis, MO, USA) at room temperature for 12 hours. By applying the Avidin-biotin method, immunohistochemistry was performed. The samples were stained with 3,3'-diaminobenzidine (DAB), counterstained with Mayer's hematoxylin, and examined under a light microscope. To count the number of BrdU positive-staining cells, three areas of each slide were selected randomly, and the stained cells within a rectangle of 150×250 µm in size among the entire cells within the growth plate were counted, and in addition, the positive cell rate in each zone of the growth plate was calculated.
Each tissue slide was pretreated with Tris-HCl solution (pH 8.0) for 10 minutes, and subsequently treated with proteinase K for 20 minutes. After washing with phosphate buffered saline (PBS), endogenous tissue peroxidase was inactivated with 3% H2O2, and the samples were washed again with PBS. The positive control of immunohistochemical examination was pretreated with DNA buffer for 10 minutes, and subsequently reacted with DNase I for 20 minutes, and thus DNA was fragmented artificially. After the pretreatment with transferase-mediated deoxyuridin triphosphated buffer for 15 minutes, reacted with terminaldeoxytransferase and Biotin-16-2'-deoxy-uridine-5'-triphosphate (dUTP) at 37℃ for 2 hours and 30 minutes, and thus dUTP was labeled. The negative control group was not treated with terminaldeoxytransferase. The reaction was terminated by immersing the samples in TB buffer for 10 minutes, and subsequently blocked with 2% bovine serum albumin for 15 minutes, washing them with PBS, reacting them with streptavidin-peroxidase for 30 minutes, and then staining them with DAB. For counter staining, nuclear fast red was used, and the samples were dehydrated again with 70, 90, and 100% ethyl alcohol, and mounted. To count the number of TUNEL positive-staining cells, three areas in each slide were selected, the number of entire cells and the number of stained cells in a rectangle of 150×250 µm in size were counted, and the rate of positive cells within the growth plate was calculated as the ratio of positive cells to the entire cells.
In the case of the change of the growth plate of each group during the experiment period, in the unloading group and the reloading group, the entire length of the growth plate was shorter than the control group (unloading control group; 382.9±11.7 µm, reloading control group; 324.5±13.8 µm), and it was 305.3±14.2 µm (79.7% of the control group) and 281.7±10.3 µm (86.8% of the control group), respectively, and the recovery of the reduction of the length after reloading was detected (Fig. 2).
In addition, in the case of the comparison of the length of each zone within the growth plate, a large difference of the length according to the change of loading in the proliferation zone and the hypertrophic zone could be detected (Fig. 3).
In all groups, BrdU positive cells representing proliferation were detected in the proliferation zone of the growth plate (Fig. 4), and in the unloading control group and reloading control group, 22.9±7.4% and 15.1±4.8% positive cell rate was shown. In the cases of the unloading group, the positive cell rate was 13.3±4.1%, and it was measured to be 57.8% of the control group, on the other hand, in the reloading cases, it was 11.0±3.8%, and the result of the recovery to 86.8% of the control group was shown (Fig. 5).
In all four groups, apoptosis in the growth plate was detected (Fig. 6), and in the unloading control group and the reloading control group, 31.9±8.7% and 32.9±9.4% positive cell rate was shown respectively. However, the unloading group and the reloading group showed 35.8±7.2% and 36.3±6.9% apoptosis level, respectively, and a significant difference among the groups was not detected (Fig. 7), in the assessment of each zone, a significant difference among the groups was not detected (data not shown).
Rats are the animals most often used in experiments studying the effects of locomotor disuse. Hindlimb rat suspension is considered to be the model of choice for simulating the effects of hypoactivity and weightlessness, with its use having been described in >800 articles.9 With respect to the skeletal system, tail suspension is an appropriate model for bone disuse studies, as shown by Bloomfield, et al.,10 who established a correlation between suspension and bone morrephometry. In our study, we took into consideration many of the recommendations of Morey-Holton and Globus.9 We used more than one control to correctly interpret the results. Two control groups were created (baseline controls) because we used young adult animals that were still developing and could present differences caused by maturation during the experimental period.10 Control for suspended group was sacrificed at 9 weeks, control for reloading group was sacrificed at 10 weeks. The differing results of ultimate stress between the two control groups reinforce the correctness of our decision.
In the growth plate, histologically distinguishable 4 types of chondrocytes form a continuous columnar pattern, and it is classified to the resting zone, the proliferation zone, the hypertrophic zone and the mineralizing zone.4,11 Chondrocytes in the resting zone are inactive flat cells that supply new cells for the proliferation to cartilage cells, cells in the proliferation zone repeat cell division and mature to a round shape, increase cell volumes and thus create a vertical space, and on the other hand, produce extracellular matrix resulting in the distance between cells becomes expanded and progresses to the hypertrophic zone. In the hypertrophic zone, the accumulation and release of calcium in chondrocytes and the calcification of the stroma are achieved, and simultaneously, the neovasculature infiltrates from the metaphysis, and cells required for bone formation, osteogenic precursor cells and chondroclasts are infiltrated. In addition, together with the infiltration of these cells, while undergoing the apoptosis process or the death process, the length grows transforming to the bone, and so called "cell kinetics" that undergoes the overall process such as the proliferation, hypertrophy, apoptosis of chondrocytes, etc.11-16 On the other hand, it has been well known that the activity of chondrocytes in the growth plate is controlled by biological factors, by various hormones, local growth factors, etc., nevertheless, actually in clinics, instead of the changes due to biological factors; the cases undergoing the reduction of dynamic weight bearing due to their condition are more prevalent.
In our study, the length of the growth plate of the suspended group was shorter than that control group because of the decrease in the length of the proliferating and hypertrophic zones, while the resting zone were not changed. After reloading, we observed that the length of the hypertrophic zone was recovered, while the proliferating zone didn't. Our results are not consistent with previous studies made by examining of growth plates by electron and light microscopy.17,18 This studies reported that there was no significant difference in the width of growth plate between the spaceflight and weight-bearing control groups. However, these studies were performed in different experimental environment (spaceflight) and were conducted in short unloading and reloading periods, so it is not appropriate to compare to our study. Some studies19,20 experimented the simulated weightlessness using the method performed in our study. They reported that the skeleton of growing rats is capable of a rapid recovery from the adverse effects of simulated weightlessness. However, this study has been biased toward the change of the bone matrix in the cortical bone or the sponge bone depending on the dynamic loading changes, and studies on the change of the chondrocytes activity within the growth plate according to the change of dynamic loading changes and its reversibility have not been conducted.
Among a variety of methods to assess the cell kinetics of chondrocytes in the growth plate, in our study, BrdU immunohistochemistry measuring cell proliferation capacity was performed. This measures cell proliferation capacity by the application of the principle that BrdU substitutes thymidine during the S-phase of DNA replication, undergoes the cell division process, and labeled, proliferating cells were assessed.13,21,22 The cell proliferation rate in the growth plate measured in our study of each control group was detected to be 22.9% and 15.1%, and it was found that with aging, the proliferation rate becomed lower. In addition, in the case of the experiment groups, the unloading group and the reloading group, in comparison with the corresponding control group, it showed 57.8% and 86.8%, and it was observed to be lowered noticeably in comparison with the normal group and the cell proliferation rate was on the recovery by the reloading.
Apoptosis in the growth plate is a physiological cell change occurred during the process of the transformation to the bone through the calcification process in the lower hypertrophic zone primarily,16,21,22 and the level substituted to the bone could be detected, and apoptosis could be induced by the damage of the growth plate by the necrosis in the secondary ossification center.16,23,24 In our study, all four groups showed approximately 34% apoptosis rate, and a significant difference among the groups was not shown. Contrary to this result, Basso and Heersche25 reported that the percentage of apoptotic chondrocytes in the growth plate cartilage was increased by unloading and that was associated with a 21% decrease in growth plate width. They suggested that apoptosis was likely a significant mechanism by which reduced load affected longitudinal growth. However, we thought that stress caused by weight bearing did not mediate a close effect on apoptosis, and it suggested that the reduction of the length of the growth plate by unloading was caused by the defect in the proliferation process of chondrocytes in the proliferation zone rather than the defect in the process of the transformation to the bone in the lower hypertrophic zone. In addition, it is considered that to elucidate whether such reduction of cell activity is caused by the change of the single process chondrocytes proliferation or the secondary due to the change of the expression of stress protein caused by the decrease of loading, additional studies should be performed in future, and it is thought that studies characterizing kinetic factors involved in irreversible cell proliferation within the growth plate are required.
In conclusion, due to the change within the growth plate in rats caused by the lack of weight bearing for 3 weeks, the reduction of the entire length, particularly, the reduction of the length in the proliferation zone and the hypertrophic zone was distinct, which was caused by the reduction of cell proliferation capacity. In addition, the change of loading did not influence the apoptosis occurring in the growth plate, and in the cases delivered reloading for 1 week after unloading, activation of chondrocytes was accelerated and thus the acceleration of the growth of the growth plate length and the recovery of the chondrocyte proliferation capacity were induced.
Figures and Tables
Fig. 2
Total length of the growth plate (*p<0.05). UG, unloading group; CUG, control for unloading; RG, reloading group; CRG, control for reloading group.
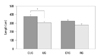
Fig. 3
Length of each zone of the growth plate (*p<0.05). (A) Comparison between unloaded and control group. (B) Comparison between reloaded and control group. rest, resting zone; prol, proliferative zone; hyper, hypertrophic zone; CUG, control for unloading; RG, reloading group; CRG, control for reloading group; UG, unloading group.
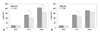
Fig. 4
Findings of BrdU immunohistochemistry (×100). (A) Control group. (B) Unloading group. (C) Control for reloading group. (D) Reloading group. BrdU, 5-bromo-2'-deoxyuridin.
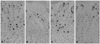
Fig. 5
Comparison of BrdU Immunohistochemistry between groups (*p<0.05). UG, unloading group; CUG, control for unloading; RG, reloading group; CRG, control for reloading group; BrdU, 5-bromo-2'-deoxyuridin.
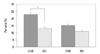
ACKNOWLEDGEMENTS
This study was supported by Yonsei University College of Medicine (#6-2006-0069) and the Korea Healthcare technology
R&D Project, Ministry for Health, Welfare & Family Affairs, Republic of Korea (#A084120).
References
1. Globus RK, Bikle DD, Morey-Holton E. Effects of simulated weightlessness on bone mineral metabolism. Endocrinology. 1984. 114:2264–2270.


2. Matsumoto T, Nakayama K, Kodama Y, Fuse H, Nakamura T, Fukumoto S. Effect of mechanical unloading and reloading on periosteal bone formation and gene expression in tail-suspended rapidly growing rats. Bone. 1998. 22:5 Suppl. 89S–93S.


3. Nyhan D, Kim S, Dunbar S, Li D, Shoukas A, Berkowitz DE. Impaired pulmonary artery contractile responses in a rat model of microgravity: role of nitric oxide. J Appl Physiol. 2002. 92:33–40.


4. Vico L, Lafage-Proust MH, Alexandre C. Effects of gravitational changes on the bone system in vitro and in vivo. Bone. 1998. 22:5 Suppl. 95S–100S.


5. Mayr W, Bijak M, Girsch W, Hofer C, Lanmüller H, Rafolt D, et al. MYOSTIM-FES to prevent muscle atrophy in microgravity and bed rest: preliminary report. Artif Organs. 1999. 23:428–431.


6. de Rooij PP, Siebrecht MA, Tägil M, Aspenberg P. The fate of mechanically induced cartilage in an unloaded environment. J Biomech. 2001. 34:961–966.


7. Gardner TN, Mishra S. The biomechanical environment of a bone fracture and its influence upon the morphology of healing. Med Eng Phys. 2003. 25:455–464.


8. Kong SY, Kim HW, Park HW, Lee SY, Lee KS. Effects of multiple drilling on the ischemic capital femoral epiphysis of immature piglets. Yonsei Med J. 2011. 52:809–817.


9. Morey-Holton ER, Globus RK. Hindlimb unloading rodent model: technical aspects. J Appl Physiol. 2002. 92:1367–1377.


10. Bloomfield SA, Allen MR, Hogan HA, Delp MD. Site- and compartment-specific changes in bone with hindlimb unloading in mature adult rats. Bone. 2002. 31:149–157.


11. Streeter GL. Developmental horizons in human embryos; a review of the histogenesis of cartilage and bone. Contrib Embryol. 1949. 33:149–168.
12. Chung UI. Essential role of hypertrophic chondrocytes in endochondral bone development. Endocr J. 2004. 51:19–24.


13. Wilsman NJ, Farnum CE, Green EM, Lieferman EM, Clayton MK. Cell cycle analysis of proliferative zone chondrocytes in growth plates elongating at different rates. J Orthop Res. 1996. 14:562–572.


16. Matsuno T, Ishida O, Arihiro K, Sunagawa T, Mori N, Ikuta Y. Cell proliferation and death of growth plate chondrocyte caused by ischemia and reperfusion. Microsurgery. 2001. 21:30–36.


17. Duke PJ, Durnova G, Montufar-Solis D. Histomorphometric and electron microscopic analyses of tibial epiphyseal plates from Cosmos 1887 rats. FASEB J. 1990. 4:41–46.


18. Sibonga JD, Zhang M, Evans GL, Westerlind KC, Cavolina JM, Morey-Holton E, et al. Effects of spaceflight and simulated weightlessness on longitudinal bone growth. Bone. 2000. 27:535–540.


19. Shimano MM, Volpon JB. Biomechanics and structural adaptations of the rat femur after hindlimb suspension and treadmill running. Braz J Med Biol Res. 2009. 42:330–338.


20. Wronski TJ, Morey ER. Recovery of the rat skeleton from the adverse effects of simulated weightlessness. Metab Bone Dis Relat Res. 1983. 4:347–352.
21. Farnum CE, Wilsman NJ. Determination of proliferative characteristics of growth plate chondrocytes by labeling with bromodeoxyuridine. Calcif Tissue Int. 1993. 52:110–119.


22. Vanky P, Brockstedt U, Hjerpe A, Wikström B. Kinetic studies on epiphyseal growth cartilage in the normal mouse. Bone. 1998. 22:331–339.


23. Roach HI, Erenpreisa J, Aigner T. Osteogenic differentiation of hypertrophic chondrocytes involves asymmetric cell divisions and apoptosis. J Cell Biol. 1995. 131:483–494.

