Abstract
Purpose
Cockroach (CR) is an important inhalant allergen and can induce allergic asthma. However, the mechanism by which CR induces airway allergic inflammation and the role of endotoxin in CR extract are not clearly understood in regards to the development of airway inflammation. In this study, we evaluated whether endotoxin is essential to the development of CR induced airway allergic inflammation in mice.
Materials and Methods
Airway allergic inflammation was induced by intranasal administration of either CR extract, CR with additional endotoxin, or endotoxin depleted CR extract, respectively, in BALB/c wild type mice. CR induced inflammation was also evaluated with toll like receptor-4 (TLR-4) mutant (C3H/HeJ) and wild type (C3H/HeN) mice.
Results
Intranasal administration of CR extracts significantly induced airway hyperresponsiveness (AHR), eosinophilic and neutrophilic airway inflammation, as well as goblet cell hyperplasia in a dose-dependent manner. The addition of endotoxin along with CR allergen attenuated eosinophilic inflammation, interleukin (IL)-13 level, and goblet cell hyperplasia of respiratory epithelium; however, it did not affect the development of AHR. Endotoxin depletion in CR extract did not attenuate eosinophilic inflammation and lymphocytosis in BAL fluid, AHR and IL-13 expression in the lungs compared to CR alone. The attenuation of AHR, eosinophilic inflammation, and goblet cell hyperplasia induced by CR extract alone was not different between TLR-4 mutant and the wild type mice. In addition, heat inactivated CR extract administration induced attenuated AHR and eosinophilic inflammation.
Asthma morbidity continues to rise, especially among inner-city children. Recent data indicate that the disproportionate increase of asthma in densely populated urban areas appears to be due to increases in allergen exposure. In particular, Cockroach (CR) allergen exposure has demonstrated a significant correlation with a rise in adolescent and childhood asthma, in more densely crowded inner cities, where large numbers of CRs can be found.1,2 Busse and Mitchell3 reported evidence that sensitization to CR allergen and the level of exposure was associated with hospital admission and emergency room visits due to asthma for both adults and children.
For the last 10 years, mouse models of asthmas has been developed to investigate the mechanisms of asthma.4 Among them, OVA and indoor allergens, such as CR and dust mite, are commonly used as allergens for mouse models.5-7 However, each model has its own advantages and disadvantages, and no model is identical to the conditions found in human asthma.8 CR is common and has been associated with the sensitization and severity of asthma in humans.1,2 Moreover, CR can sensitize a host via the respiratory system without any adjuvant,9,10 and has other initiating factors for innate immune responses, such as endotoxin,11 protease,12,13 and chitin,14 which are certainly present and play important roles in the sensitization and induction of allergic inflammation.15
Endotoxin is an outer layer component of the outer cell membrane of all Gram-negative bacteria, and influences disease severity by acting as a natural adjuvant to allergic inflammation. A significant amount of data has shown that exposure to endotoxin in the environment or via cigarette smoke leads to exacerbation of pre-existing asthma.16,17 However, other recent studies have claimed that endotoxin has protective effects against developing asthma,18-20 and high endotoxin exposure in rural houses which kept stable animals prevented the development of asthma.18 These results suggested not only the existence of an early protective window period, but also that exposure of endotoxin after allergen sensitization aggravates allergic disease.21 In this endotoxin-allergy relationship, genetic factors are also involved in host responses.22,23 Further complicating the enigma, the essential immunostimulant of each indoor allergen may be different. For house dust mites, β-glucan, instead of protease activity or endotoxin, may be essential for activation of respiratory epithelium and recruitment of dendritic cells; however, it is not found in CR extract.24
CR extracts sensitize via the respiratory system without adjuvant and lead to chronic inflammation rather than tolerance in mouse models. However, the underlying mechanism of the CR mouse model of asthma and the role of endotoxin in CR extracts remains controversial.25 Therefore, we evaluated the role of endotoxin in a CR-induced mouse model of asthma using BALB/c and TLR-4, a crucial molecule for the function of endotoxin, mutant mice.
Female BALB/c (6-8 weeks old), TLR-4 mutant (C3H/HeJ), and TLR-4 wild type (C3H/HeN) mice were purchased from Jackson Laboratories (Seoul, Korea). All mice were housed under specific pathogen free conditions, the American Association for the Accreditation of Laboratory Animal Care-approved facilities, and maintained on a 12 hour light-dark cycle with food and water ad libitum. All experiments described in this study were approved by the Animal Research Ethics Board of Yonsei University (Seoul, Korea).
We prepared crude German CR extract as previously mentioned.26 Thirty grams of live or frozen Blattela germanica were pulverized in liquid nitrogen. The sample was then defatted in 200 mL of 1 : 1 ethyl ether/ethyl acetate and extracted overnight with slow stirring at 4℃ in phosphate-buffered saline (PBS) (pH 7.4) containing 6 mM 2-mercaptoethanol and 1 mg/mL of 1-phenyl-3-(2-thiazolyl)-2-thiourea to prevent melanization. The extract was then centrifuged at 9300 g for 30 min at 4℃, and the supernatant was filtered through a 0.22 mm filter, and then lyophilized. Supernatant contained 0.11 U of Bla g 1 per mg of CR extract. The level of endotoxin in the lyophilized extract was 5810 EU/mg according to the QCL-1000 chromogenic LAL kit (Cambrex, East Rutherford, NJ, USA). For the preparation of endotoxin added to CR extract, 2 µg of endotoxin (10000 EU; Sigma-Aldrich, MO, USA) was added to 300 µg of CR extract.
Endotoxin was eliminated from the above crude CR extract by endotoxin removing columns (Thermo, Rockford, IL, USA). Before use, detoxi-gel resin was washed sequentially with five resin-bed volumes of 1% sodium deoxycholate, followed by 3-5 resin-bed volumes of pyrogen-free buffer or water to remove the detergent. The crude CR extract was analapplied
to the column, and the column was incubated for one hour before collecting the sample. After storing the sample, the level of endotoxin was found to be 0.0876 EU/mg according to the QCL-1000 chromogenic LAL kit (Cambrex, East Rutherford, NJ, USA).
BALB/c mice were administered with an intranasal saline or CR allergen (18.8 µg, 75 µg, or 300 µg of CR allergen in 40 µL of PBS/mouse, respectively) twice per week during the five-week dose-dependent experiment. The total amount of CR extract administered to each mouse of this experiment was 188 µg, 750 µg or 3000 µg, respectively. Also, BALB/c mice were administered twice per week with an intranasal saline, CR extract (300 µg of CR/40 µL of PBS), CR extractwith additional endotoxin (10000 EU) or endotoxin depleted CR extractfor endotoxin dependent experiments without any adjuvant during the five weeks under isoflurane anesthesia. To investigate the function of endotoxin, TLR-4 mutant mice (C3H/HeJ) and TLR-4 wild type mice (C3H/HeN) were used. C3H/HeJ mice are an inbred strain that are TLR4 defective, and demonstrate endotoxin hypo-responsiveness.27 These mice were administered twice per week with an intranasal saline or CR allergen (300 µg of CR/40 µL of PBS) without any adjuvant during the five weeks under isoflurane anesthesia. Finally, CR extracts were treated with heat at 65℃ for 30 minutes to remove protease activity, which were then given to BALB/c mice to investigate the role of protease in CR extracts. Each group comprised five or six mice, and all experiments were performed more than three times.
Forty-eight hours after the last challenge, AHR to methacholine (MCh) was measured in mice intubated with an 18 gauge steel tube. Mice were ventilated with a flexiVent 5.1® small animal ventilator (SCIREQ, Montreal, PQ, Canada) at 120 breaths per minute and a tidal volume of 30 mL/kg. A positive end expiratory pressure was set at 2 cmH2O. The ventilation rate was set above the normal breathing rate to suppress spontaneous breathing during measurements.
Mice were challenged with a saline control aerosol followed by increasing concentrations of MCh (Sigma-Aldrich, St. Louis, MO, USA; 3.1, 6.25, 12.5, 25, and 50 mg/mL). Aerosols were generated with an ultrasonic nebulizer and delivered to the inspiratory line of the flexiVent using a bias flow of medical air. Each aerosol was delivered for 12 seconds during regular ventilation. Two measurements were made at one-minute intervals following each aerosol.
To collect BAL fluid, lungs were lavaged with 1 mL of Hank's balanced salt solution via an intubated tube. Total cell numbers were counted with a hemocytometer. After that procedure, BAL fluid was centrifuged at 1500 rpm for 3 minutes at 4℃, and then smears of BAL cells were prepared by cytocentrifugation (cytospin3, Thermo, MA, USA) at 1000 rpm for 3 minutes. All smears were stained with Diff Quick staining. Differential cell counts in BAL cells were done with at least 200 leukocytes, using the standard hemocytologic procedure to classify the cells as either neutrophils, eosinophils, lymphocytes, or other mononuclear leukocytes (macrophages and monocytes). After collecting BAL fluid, one side of the lungs were removed and homogenized in 3 mL of lysis buffer containing 0.5% Triton X-100, 150 mM NaCl, 15 mM Tris, 1 mM CaCl, 1 mM MgC1, and protease inhibitor (Sigma-Aldrich, St. Louis, MO, USA) at a pH of 7.40, using a tissue homogenizer (Biospec Products, Bartlesville, OK, USA). Homogenates were incubated on ice for 30 min, and then centrifuged at 2500 rpm for 10 min. Supernatants were collected, passed through a 0.45-micron filter (Gelman Sciences, Ann Arbor, MI, USA), and then stored at -20℃ for assessment of cytokine levels.
ELISA kits were purchased from R&D Systems (Minneapolis, MN, USA). Interleukin (IL)-5, 13, and interferon-gamma (IFN-γ) in the homogenate of lung tissue were detected by sandwich ELISA according to the manufacturer's recommendations.
After BAL fluid collection, one side of the lungs was inflated with 10% formalin at a standard pressure. Tissues were then embedded in paraffin, and 3 µm thick sections were cut and stained with H&E, trichrome for fibrosis, and Periodic Acid-Schiff for goblet cell hyperplasia.
Statistical differences in the mean values among groups were determined by using one-way ANOVA. The paired Student's t-test was used to compare the mean between two groups. Differences in AHR were determined by F-test analysis which compared values over the entire curve between each treatment group. From this F-score, a value for p was generated. In all cases, a value of p<0.05 was considered statistically significant.
We evaluated dose-dependency for the development of CR extract-induced asthma in BALB/c mice. Mice sensitized with 75 and 300 µg/mL concentrations of CR extract demonstrated significant increases in AHR at MCh concentrations of 25 and 50 mg/mL, compared to the sham animal (p<0.05), and AHR had a tendency to increase in a dose-dependent manner (Fig. 1A).
Analysis of BAL fluid revealed significant differences in the number of total cells, macrophages, lymphocytes, eosinophils, and neutrophils between mice sensitized with any concentration of CR extract compared with sham mice. The numbers of these cells in BAL fluid were increased dose-dependently (Fig. 1B). We also observed that the expression of IL-13 in lung homogenate was significantly higher in the mice administered with any concentration of CR extract than sham mice. The levels of IL-13 in lung homogenate were also increased in a dose-dependent manner (Fig. 1C).
The lungs of CR extractadministered mice exhibited remarkable eosinophilic perivascular and peribronchial inflammation with clear evidence of goblet cell hyperplasia (data not shown).
We evaluated the effects of endotoxin on the development of CR extract-induced asthma in BALB/c mice. Endotoxin addition (10000 EU) or endotoxin depletion in CR extract did not affect the development of AHR (Fig. 2A). Analysis of BAL fluid revealed significant differences in the number of eosinophils between mice sensitized with CR, CR without endotoxin, and CR with additional endotoxin. The number of eosinophils in mice administered with CR with additional endotoxin was significantly lower than in those administered with CR alone (p<0.05) (Fig. 2B).
The level of IL-13 and IL-5 in mice administered with CR without endotoxin was not different from mice administered with CR alone, whereas the levels in mice administered with additional endotoxin were significantly lower than in mice administered with CR alone (Fig. 2C and D). We also observed remarkable perivascular and peribronchial inflammation in all of the CR administered mice, regardless of endotoxin. However, goblet cell hyperplasia in respiratory epithelium was markedly diminished with the addition of endotoxin; nevertheless, perivascular and peribronchial inflammatory cell infiltrations were prominent in these mice (Fig. 3).
We evaluated the effects of TLR-4 on the development of CR-induced asthma in C3H/HeN mice and C3H/HeJ mice. The mutant mice developed AHR at all MCh concentrations (p<0.05); however, but AHR in wild type mice was not different from that of sham mice (Fig. 4A). There were significantly increased numbers of total cells, macrophages, lymphocytes, eosinophils and neutrophils in the BAL fluid of the C3H/HeN and C3H/HeJ mice sensitized to CR compared to sham mice. However, eosinophil count in BAL fluid was more prominent in the mutant mice than in the wild type mice (p<0.05) (Fig. 4B). IL-13 expression in the TLR-4 mutant mice was significantly higher than that of the TLR-4 wild type mice (p<0.05). However, the expression of IFN-γ was not affected by TLR-4 mutation and sensitization of CR extract (Fig. 4C and D). We also observed more remarkable perivascular and peribronchial inflammation in CR-sensitized TLR-4 mutant mice than in the wild type, (Fig. 5A-E) but there was no difference in peribronchial fibrosis between the two groups (Fig. 5F-J).
It is known that the protease activity of allergens participates in the development of mucosal allergic sensitization. Thus, we examined whether allergic responses to CR extracts were dependent on protease activity. We removed CR protease activity using heat inactivation, which eliminated protease activity.13 Mice that were administrated with heat-inactivated CR extractshowed significantly decreased AHR and lower numbers of inflammatory cells in their BAL fluid, with dramatically lower eosinophilic infiltration, compared with non-heat-treated CR mice. These results indicated that the presence of heat-sensitive protease activity in CR extracts plays an important role in the development of allergic airway inflammation, particularly in terms of eosinophil infiltration (Fig. 6).
Several animal models of allergic asthma, using parasite, OVA, and plant extracts as allergens, have been developed.28,29 Among these models, the OVA asthma model using aluminum hydroxide as an adjuvant is the standard animal model and has greatly contributed to the understanding of many important mechanisms of asthma and the basic processes that occur during allergic airway responses.30 Nevertheless, the OVA model is somewhat misrepresentative from that of human asthma: allergic diseases in humans are chronic in nature resulting from intermittent or continuous exposure to allergen, and structural changes such as airway remodeling are characteristic. On the other hand, animal models using OVA are inadequate for evaluating the processes involved in human airway remodeling.31,32
Therefore, many investigators have attempted to develop a physiologic asthma model more resemblant to that of humans. Johnson, et al.33 also developed a physiologic asthma model using house dust mites (HDM). Continuous exposure to HDM for up to 7 weeks resulted in sustained AHR and airway inflammation characterized by Th2 effector cell and eosinophil accumulation. These presentations resemble those that have been observed in human asthmatic patients; therefore, we are able to explain a more detail mechanism of the inflammatory process as well as the structural change of the airway following allergen exposure as a result of the development of this model.
CR is not only an important human allergen, but also a potent allergen in mice. Exposure to CR is closely correlated to asthma severity, and it can sensitize a host via the respiratory system without any adjuvant.9,10 In the present study, we administered CR extracts via only intranasal administration, and our results showed that CR extracts induced AHR, eosinophilic and neutrophilic inflammation, and goblet cell hyperplasia of respiratory epithelium in a dose-dependent manner.
Although some asthma animal models using CR have been introduced, there are some critical problems with these models.34,35 These studies did not explain the function of endotoxin in CR extracts: crude CR extractcontains a relatively large amount of endotoxin,10 and the role of endotoxin contaminated in CR extracts is controversial. Some investigators have attempted to explain the function of endotoxin by challenging mice with endotoxin;36,37 however, the clinical relevance of this model is not clear. It has been suggested that exposure to low levels of endotoxin may exacerbate asthmatic responses, whereas exposure to high levels might be protective. This is based on two animal studies with opposite findings. Wan, et al.38 showed that mice exposed to 40 ng/mL of aerosolized endotoxin prior to OVA challenge resulted in higher specific IgE levels and inhibited tolerance to OVA compared to mice exposed to saline prior to OVA challenge. On the other hand, Tulic, et al.39 found that exposure to 50 µg/mL of endotoxin with OVA challenge showed a protective effect and prevented the IgE response in rats. These studies clearly showed the role of endotoxin in a pure OVA asthma model. In addition to endotoxin and allergens, CR extracts contain several important immunostimulants such as proteases and chitins.14,15 Recent studies have proven that protease activity in CR extracts activates innate immune responses during sensitization, leads to the development of CR induced allergic inflammation, and overcomes allergen tolerance in mice.12,13,40 We also showed that elimination of protease activity in CR extracts can inhibit the development of AHR and allergic inflammation in mice. Serine protease's receptor, protease activating receptor-2 (PAR-2), is found on a variety cells, including macrophages and dendritic cells. PAR-2 activation in the airway concurrent with exposure to inhaled OVA induces allergic sensitization via dendritic cell activation, whereas exposure to OVA alone may induce allergen tolerance.12
The lack of a good animal model using endotoxin has so far made it difficult for investigators to explain the relationship between endotoxin, allergen and asthmatic responses. In this study, we showed that CR extract induced AHR and airway inflammation in a dose-dependent manner in BALB/c mice. Moreover, the results obtained with this model showed that the addition of endotoxin did not improve AHR induced by CR allergen extracts, but rather decreased eosinophilic inflammation in BAL fluid and decreased IL-13, IL-5, and goblet cell hyperplasia, compared with those induced by crude CR extracts alone. Also, depletion of endotoxin did not demonstrate a difference in AHR, Th2 cytokine (IL-13, IL-5), and goblet cells in respiratory epithelium. These data agree quite well with previous studies. Natarajan, et al.,41 who administered endotoxin depleted CR extract to mice, reported no significant difference in the infiltration of allergic inflammation, and the development of AHR between endotoxin reduced and the other groups. They also demonstrated elevated production of IL-5, IL-13 and IFN-γ in endotoxin reduced group. We also used TLR-4 mutant (C3H/HeJ) and TLR-4 wild type (C3H/HeJ) mice to evaluate the function of endotoxin in the CR extract-induced asthma model. TLR-4 functions as the signal transduction receptor for endotoxin in the cells of immune systems including macrophages and dendritic cells.42 Our results showed that AHR, eosinophil count in BAL fluid, IL-13 in lung homogenate, and goblet cell hyperplasia induced in C3H/HeJ mice by CR extractwere similar to that of the wild type mice (C3H/HeN). These results indicated that endotoxin in CR extracts plays a limited role in inducing allergic inflammation, according to this model. Consistent to our study, Arizmendi, et al.13 also reported that the protease activity of CR extract is sufficient for CR sensitization and the induction of allergic inflammation even in TLR-4 knock-out mice. These results are also concordant with a human epidemiologic study, which showed that endotoxin levels in samples of dust from a child's mattress were inversely related to the occurrence of hay fever, atopic asthma, and atopic sensitization, and cytokine production by leukocytes.18
In the present study, we characterized a mouse asthma model in response to CR extract and investigated the roles of endotoxin and protease therein. CR exposure can elicit asthma-like pulmonary inflammation characterized by AHR, eosinophilia, elevation of Th2 cytokine and goblet cell hyperplasia, which were related to the amount of CR extract exposure. CR extract-induced allergic responses developed not as a result of endotoxin, but rather from protease. Even though high dose endotoxin inhibited eosinophilic inflammation, Th2 cytokine levels and goblet cell hyperplasia were induced by CR allergen. The CR extract-induced mouse asthma model presented herein will be a valuable tool and may allow for the discovery of the mechanism of allergen sensitization and its relationship with innate immune responses in allergic asthma.
Figures and Tables
Fig. 1
Dose-dependency of CR extract-induced asthma model in BALB/c mice. Developments of AHR (A), airway inflammation (B), and IL-13 level in the lungs (C) showed the dose-dependency of CR extracts. (A) CR 75 and 300 µg/mL administrated mice demonstrated significant increases in AHR at MCh concentrations of 25 and 50 mg/mL compared to the sham animal. (B) Total cell number, lymphocyte, eosinophil and neutrophil counts demonstrated statistical differences between sham mice and CR extractadministered mice of any concentration, and (C) IL-13 levels demonstrated significant differences at any concentration of CR extract administration compared to sham mice). *p<0.01, †p<0.05. CR, cockroach; AHR, airway hyperresponsiveness; IL, interleukin; MCh, methacholine.
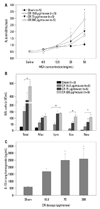
Fig. 2
Effects of endotoxin in CR extract-induced asthma model in BALB/c mice. Development of AHR (A) and airway inflammation (B). Cytokine levels (C and D) in the lungs of CR extract administrated BALB/c mice according to endotoxin level. (A) All CR administrated mice showed significant AHR increase at MCh concentrations of 25 and 50 mg/mL compared to sham mice. *p<0.01, †p<0.05. CR, cockroach; AHR, airway hyperresponsiveness; MCh, methacholine.
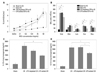
Fig. 3
PAS and trichrome staining of CR extract-induced asthma model in BALB/c mice. Representative histology of PAS (A-D) and trichrome (F-I) staining in the lung tissues. Sham mice are A and F. CR induced asthma mice are B and G. Endotoxin depleted CR induced asthma mice are C and H. Endotoxin addition CR induced asthma mice are D and I. The results of PAS and trichrome staining were quantified in E and J. *p<0.05. CR, cockroach; PAS, Periodic Acid-Schiff.
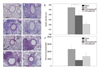
Fig. 4
CR extractinduced asthma model in TLR-4 wild type and mutant mice. Development of AHR (A), airway inflammation in BAL fluid (B), expression of IL-13 (C), and IFN-γ (D) in the lungs of TLR-4 wild type (C3HeN) and TLR-4 mutant (C3HeJ) mice in the CR induced asthma model. CR administrated C3HeN mice showed significant AHR increase at all MCh concentrations compared to sham mice. *p<0.01, †p<0.05. CR, cockroach; TLR-4, receptor-4; AHR, airway hyperresponsiveness; IL, interleukin; IFN-γ, interferon-gamma; MCh, methacholine.
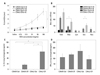
Fig. 5
PAS and trichrome staining of the CR extract-induced asthma model in TLR-4 wild type and mutant mice. Representative histology of PAS (A-D) and trichrome (F-I) staining in the lung tissues. No treated wild type mice are A and F. CR treated wild type mice are B and G. No treated TLR-4 mutant mice are C and H. CR treated TLR-4 mutant mice are D and I. The results of PAS and trichrome staining were quantified in E and J. *p<0.01, †p<0.05. CR, cockroach; PAS, Periodic Acid-Schiff; TLR-4, receptor-4.
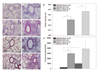
Fig. 6
Effects of heat inactivation on the CR extract-induced asthma model in BALB/c mice. Development of AHR (A) and airway inflammation (B) in BALB/c mice administered with heat inactivated CR or non-heat-treated CR. Heat inactivated CR administrated mice showed significant AHR decrease at MCh concentrations of 25 and 50 mg/mL compared to non-heat-treated CR administrated mice. *p<0.01, †p<0.05. CR, cockroach; AHR, airway hyperresponsiveness; MCh, methacholine.
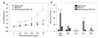
ACKNOWLEDGEMENTS
This study was supported by a grant from the Korea Healthcare Technology R&D Project, Ministry for Health, Welfare & Family Affairs, Republic of Korea (A092076).
References
1. Sarpong SB, Hamilton RG, Eggleston PA, Adkinson NF Jr. Socioeconomic status and race as risk factors for cockroach allergen exposure and sensitization in children with asthma. J Allergy Clin Immunol. 1996. 97:1393–1401.


2. Rosenstreich DL, Eggleston P, Kattan M, Baker D, Slavin RG, Gergen P, et al. The role of cockroach allergy and exposure to cockroach allergen in causing morbidity among inner-city children with asthma. N Engl J Med. 1997. 336:1356–1363.


3. Busse WW, Mitchell H. Addressing issues of asthma in inner-city children. J Allergy Clin Immunol. 2007. 119:43–49.


4. Bice DE, Seagrave J, Green FH. Animal models of asthma: potential usefulness for studying health effects of inhaled particles. Inhal Toxicol. 2000. 12:829–862.


5. de Siqueira AL, Russo M, Steil AA, Facincone S, Mariano M, Jancar S. A new murine model of pulmonary eosinophilic hypersensitivity: contribution to experimental asthma. J Allergy Clin Immunol. 1997. 100:383–388.


6. Clarke AH, Thomas WR, Rolland JM, Dow C, O'Brien RM. Murine allergic respiratory responses to the major house dust mite allergen Der p 1. Int Arch Allergy Immunol. 1999. 120:126–134.


7. Warner RL, Lukacs NW, Shapiro SD, Bhagarvathula N, Nerusu KC, Varani J, et al. Role of metalloelastase in a model of allergic lung responses induced by cockroach allergen. Am J Pathol. 2004. 165:1921–1930.


9. Arruda LK, Vailes LD, Ferriani VP, Santos AB, Pomés A, Chapman MD. Cockroach allergens and asthma. J Allergy Clin Immunol. 2001. 107:419–428.


10. Hong JH, Lee SI, Kim KE, Yong TS, Seo JT, Sohn MH, et al. German cockroach extract activates protease-activated receptor 2 in human airway epithelial cells. J Allergy Clin Immunol. 2004. 113:315–319.


11. Kim J, Merry AC, Nemzek JA, Bolgos GL, Siddiqui J, Remick DG. Eotaxin represents the principal eosinophil chemoattractant in a novel murine asthma model induced by house dust containing cockroach allergens. J Immunol. 2001. 167:2808–2815.


12. Ebeling C, Lam T, Gordon JR, Hollenberg MD, Vliagoftis H. Proteinase-activated receptor-2 promotes allergic sensitization to an inhaled antigen through a TNF-mediated pathway. J Immunol. 2007. 179:2910–2917.


13. Arizmendi NG, Abel M, Mihara K, Davidson C, Polley D, Nadeem A, et al. Mucosal allergic sensitization to cockroach allergens is dependent on proteinase activity and proteinase-activated receptor-2 activation. J Immunol. 2011. 186:3164–3172.


14. Lee CG. Chitin, chitinases and chitinase-like proteins in allergic inflammation and tissue remodeling. Yonsei Med J. 2009. 50:22–30.


15. Jeong KY, Hong CS, Lee JS, Park JW. Optimization of allergen standardization. Yonsei Med J. 2011. 52:393–400.


16. Hasday JD, Bascom R, Costa JJ, Fitzgerald T, Dubin W. Bacterial endotoxin is an active component of cigarette smoke. Chest. 1999. 115:829–835.


17. Reed CE, Milton DK. Endotoxin-stimulated innate immunity: a contributing factor for asthma. J Allergy Clin Immunol. 2001. 108:157–166.


18. Braun-Fahrländer C, Riedler J, Herz U, Eder W, Waser M, Grize L, et al. Environmental exposure to endotoxin and its relation to asthma in school-age children. N Engl J Med. 2002. 347:869–877.


19. Strachan DP. Family size, infection and atopy: the first decade of the "hygiene hypothesis". Thorax. 2000. 55:Suppl 1. S2–S10.


20. Matricardi PM, Franzinelli F, Franco A, Caprio G, Murru F, Cioffi D, et al. Sibship size, birth order, and atopy in 11,371 Italian young men. J Allergy Clin Immunol. 1998. 101(4 Pt 1):439–444.


21. Michel O, Kips J, Duchateau J, Vertongen F, Robert L, Collet H, et al. Severity of asthma is related to endotoxin in house dust. Am J Respir Crit Care Med. 1996. 154(6 Pt 1):1641–1646.


22. Eder W, Klimecki W, Yu L, von Mutius E, Riedler J, Braun-Fahrländer C, et al. Opposite effects of CD 14/-260 on serum IgE levels in children raised in different environments. J Allergy Clin Immunol. 2005. 116:601–607.


23. Zambelli-Weiner A, Ehrlich E, Stockton ML, Grant AV, Zhang S, Levett PN, et al. Evaluation of the CD14/-260 polymorphism and house dust endotoxin exposure in the Barbados Asthma Genetics Study. J Allergy Clin Immunol. 2005. 115:1203–1209.


24. Nathan AT, Peterson EA, Chakir J, Wills-Karp M. Innate immune responses of airway epithelium to house dust mite are mediated through beta-glucan-dependent pathways. J Allergy Clin Immunol. 2009. 123:612–618.


25. Campbell EM, Kunkel SL, Strieter RM, Lukacs NW. Temporal role of chemokines in a murine model of cockroach allergen-induced airway hyperreactivity and eosinophilia. J Immunol. 1998. 161:7047–7053.
26. Lee KE, Kim JW, Jeong KY, Kim KE, Yong TS, Sohn MH. Regulation of German cockroach extract-induced IL-8 expression in human airway epithelial cells. Clin Exp Allergy. 2007. 37:1364–1373.


27. Russo M, Lutton JD. Decreased in vivo and in vitro colony stimulating activity responses to bacterial lipopolysaccharide in C3H/HeJ mice. J Cell Physiol. 1977. 92:303–307.


28. Pritchard DI, Eady RP, Harper ST, Jackson DM, Orr TS, Richards IM, et al. Laboratory infection of primates with Ascaris suum to provide a model of allergic bronchoconstriction. Clin Exp Immunol. 1983. 54:469–476.
29. Mapp C, Hartiala J, Frick OL, Shields RL, Gold WM. Airway responsiveness to inhaled antigen, histamine, and methacholine in inbred, ragweed-sensitized dogs. Am Rev Respir Dis. 1985. 132:292–298.
30. Flach TL, Ng G, Hari A, Desrosiers MD, Zhang P, Ward SM, et al. Alum interaction with dendritic cell membrane lipids is essential for its adjuvanticity. Nat Med. 2011. 17:479–487.


31. Leigh R, Ellis R, Wattie J, Southam DS, De Hoogh M, Gauldie J, et al. Dysfunction and remodeling of the mouse airway persist after resolution of acute allergen-induced airway inflammation. Am J Respir Cell Mol Biol. 2002. 27:526–535.


32. Blyth DI, Pedrick MS, Savage TJ, Hessel EM, Fattah D. Lung inflammation and epithelial changes in a murine model of atopic asthma. Am J Respir Cell Mol Biol. 1996. 14:425–438.


33. Johnson JR, Wiley RE, Fattouh R, Swirski FK, Gajewska BU, Coyle AJ, et al. Continuous exposure to house dust mite elicits chronic airway inflammation and structural remodeling. Am J Respir Crit Care Med. 2004. 169:378–385.


34. Zhou D, Chen G, Kim JT, Lee LY, Kang BC. A dose-response relationship between exposure to cockroach allergens and induction of sensitization in an experimental asthma in Hartley guinea pigs. J Allergy Clin Immunol. 1998. 101:653–659.


35. McKinley L, Kim J, Bolgos GL, Siddiqui J, Remick DG. Reproducibility of a novel model of murine asthma-like pulmonary inflammation. Clin Exp Immunol. 2004. 136:224–231.


36. Eisenbarth SC, Piggott DA, Huleatt JW, Visintin I, Herrick CA, Bottomly K. Lipopolysaccharide-enhanced, toll-like receptor 4-dependent T helper cell type 2 responses to inhaled antigen. J Exp Med. 2002. 196:1645–1651.


37. Piggott DA, Eisenbarth SC, Xu L, Constant SL, Huleatt JW, Herrick CA, et al. MyD88-dependent induction of allergic Th2 responses to intranasal antigen. J Clin Invest. 2005. 115:459–467.


38. Wan GH, Li CS, Lin RH. Airborne endotoxin exposure and the development of airway antigen-specific allergic responses. Clin Exp Allergy. 2000. 30:426–432.


39. Tulic MK, Holt PG, Sly PD. Modification of acute and late-phase allergic responses to ovalbumin with lipopolysaccharide. Int Arch Allergy Immunol. 2002. 129:119–128.


40. Kheradmand F, Kiss A, Xu J, Lee SH, Kolattukudy PE, Corry DB. A protease-activated pathway underlying Th cell type 2 activation and allergic lung disease. J Immunol. 2002. 169:5904–5911.

