Abstract
Purpose
In patients with diabetic end stage renal disease (ESRD), glycated albumin (GA) reflects recent glycemic control more accurately than glycated hemoglobin (HbA1c). We evaluated the relationship between GA and average blood glucose (AG) level and developed an estimating equation for translating GA values into easier-to-understand AG levels.
Materials and Methods
A total of 185 ESRD patients, including 154 diabetic and 31 non-diabetic participants, were enrolled (108 hemodialysis, 77 peritoneal dialysis). Patients were asked to perform four-point daily self-monitoring of capillary blood glucose (SMBG) at least three consecutive days each week for four weeks. Serum levels of GA, HbA1c and other biochemical parameters were checked at baseline, as well as at 4 and 8 weeks.
Results
Approximately 74.3±7.0 SMBG readings were obtained from each participant and mean AG was 169.1±48.2 mg/dL. The correlation coefficient between serum GA and AG levels (r=0.70, p<0.001) was higher than that of HbA1c and AG (r=0.54, p<0.001). Linear regression analysis yielded the following equation: estimated AG (eAG) (mg/dL)=4.71×GA%+73.35, and with this formula, serum GA levels could be easily translated to eAG levels. Multivariate analysis revealed significant contributions of postprandial hyperglycemia (β=0.25, p=0.03) and serum albumin (β=0.17, p=0.04) in determining serum GA level, independent to other clinical parameters.
Numerous studies have emphasized the importance of strict blood glucose control for protecting against microvascular and macrovascular diabetic complications.1-4 In addition to patients without overt diabetic nephropathy, the benefits of adequate glycemic control have also been emphasized in patients with end-stage renal disease (ESRD), even after the start of dialysis.2-5 A seven-year observational study with 114 diabetic hemodialysis patients showed significantly higher mortality and worse prognoses in patients with poor glycemic control (HbA1c≥8.0%).6 Shima, et al.7 also reported that intensive management of diabetic control at a stringent mean postprandial glucose level of less than 180 mg/dL could improve the life expectancy of diabetic dialysis patients. Although an issue of considerable debate, currently recommended glucose targets in ESRD patients are identical to those for the general population:8 a fasting blood glucose level below 140 mg/dL, a postprandial value of less than 200 mg/dL, and HbA1c less than 6.5-7%.9-11
To date, HbA1c has been the most widely recognized marker of glycemic control, reflecting one's average glycemic status for the preceding 2 to 3 months.12 However in ESRD patients, the availability of HbA1c is a limitation to its use as a marker of glycemic control, because it can be influenced by various clinical factors such as reduced red-blood cell (RBC) lifespan, recent transfusion, iron deficiency, metabolic acidosis or frequent erythropoietin injection.10-13 As a result, HbA1c tends to underestimate glycemic status, especially in patients with reduced renal function.12,14,15 On the basis of a study involving Japanese hemodialysis patients, serum glycated albumin (GA) has been proposed as an alternative marker of glycemic control in dialysis patients,12 since levels of GA are unaffected by changes in RBC survival time or erythropoietin use.16 Compared to HbA1c, GA responds much sooner to changes in glycemic levels, reflecting short-term glycemic control over the previous 2 to 3 weeks. Furthermore, serum GA may also be a marker of the development of cardiovascular diseases.17 In an observational study by Fukuoka, et al.,5 high GA (≥29%) was a significant predictor of cardiovascular death compared to low GA (<29%), but this was not observed with HbA1c. Similarly, Yamada, et al.18 showed that serum GA level, not HbA1c, is closely associated with peripheral vascular calcification and pulse-wave velocity, an indicator of advanced atherosclerosis and arterial stiffness in diabetic dialysis patients.
Nevertheless, there are some limitations to the use of GA in clinical practice, due to lack of validated reference ranges; even though the GA/HbA1c ratio is usually estimated as 3.0, this empirical estimate has no statistical background.19 Furthermore compared to HbA1c, for which there is an established equation to translate it into average glucose (AG) values,20-22 there have been few reports on the mathematical method of converting GA measurements into easier-to-understand AG levels in ESRD patients, including peritoneal dialysis (PD) patients.
Therefore, we sought to confirm the measurement of GA as a substitute to HbA1c quantification for the accurate assessment of glycemic control in patients with ESRD. Moreover, we attempted to develop an estimating equation for investigating the relationship between GA and AG levels. The optimal target range for GA in diabetic dialysis patients was also examined in this study.
This was a single-center prospective study conducted at Severance Hospital in Korea between March 2010 and October 2010. Type 1 and 2 diabetic ESRD patients as well as non-diabetic patients between the ages of 18 and 70 years were recruited. Diabetic patients received either an oral hypoglycemic agent, insulin or both, and were required to have relatively stable glycemic control as manifested by at least two HbA1c results in the previous six months with no more than 1% difference. Patients were also classified as hemodialysis (HD) or PD group according to modality of maintaining dialysis during the study period. The duration of diabetes was determined based on medical records. For patients not diagnosed at our center, however, the duration was presumed based on the patients' medical history. Non-diabetic patients included those with no history of diabetes, a plasma glucose level <126 mg/dL after an overnight fast, and an HbA1c level <6.5% at the time of presentation. Criteria for exclusion were extremely young or old age, presence of severe diabetic retinopathy, history of recent hospitalization, and non-compliance. Patients with complicated comorbid conditions or who had taken medications that could influence plasma glucose levels during the previous three-month period were also excluded. This study was performed with approval from the local Institutional Review Board, and informed consent was obtained from all participants.
To measure casual blood glucose level, all participants were asked to perform four-point daily self-monitoring of capillary blood glucose (SMBG) (OneTouch Ultra; Lifescan, Milipitas, CA, USA) for at least three consecutive days per week for four weeks. Capillary blood glucose was typically tested at fasting (before breakfast), postprandial (120 min after breakfast), postabsorptive (at 5:00 P.M), and at bedtime. To be enrolled in this study, the number of recorded SMBGs must have totaled at least 40 measurements or more during the study period (a minimum of twice a day for at least two days per week).
A small drop of blood obtained by pricking the skin with a lancet was used to measure capillary glucose level, whereas, serum glucose level was determined using a centrifuged blood sample (i.e., serum). While RBCs in whole blood have a higher concentration of protein, serum has higher water content and consequently more dissolved glucose than whole blood. In general, serum glucose level could reach a level equal to capillary blood glucose level multiplied by 1.15. The results of SMBG tests were recorded and any events including overeating, missing a meal, feelings of dizziness, or other symptoms suggesting episodes of hypoglycemia were also documented. To encourage blood sugar testing and to reduce hypoglycemic events, all patients were provided appropriate feedback by dialysis staff once per week. After eight weeks of initial SMBG, all patients were asked to measure follow-up SMBG for two weeks to verify stable glycemic state.
Average glucose levels for each testing (fasting, postprandial, postabsorptive, and bedtime) and the mean glucose value of total SMBG tests were calculated by the area-under-the-curve (AUC) of each glucose profile using the trapezoidal rule.23
Blood samples for serum GA, HbA1c levels and biochemical parameters including serum hemoglobin, albumin, blood urea nitrogen, and creatinine were obtained at baseline and at the end of 4 and 8 weeks. To confirm the accuracy of SMBG results, fasting plasma glucose were measured weekly at the dialysis center before the start of each session of dialysis and compared with the results of SMBG.
Serum HbA1c levels were analyzed using routine high-performance liquid chromatography (HPLC, Bio-Rad Laboratories Inc., Hercules, CA, USA), and GA level was measured by enzymatic methods using the Lucica GA-L kit (Asahi Kasei Pharma Corp., Tokyo, Japan) with an automatic spectrophotometer. GA level was given as a percentage of GA divided by the total amount of serum albumin.
Demographic biochemical data were expressed as mean±standard deviation (SD) or as percentages. Chi-square and independent t-tests were used to compare the DM with non-DM group or HD with PD group. Pearson's correlation coefficients were calculated to assess the strengths of the relationships between clinical variables. Simple linear regression was used to determine the association between average glucose level and GA or HbA1c, and to create a mathematical equation. For regression analysis, GA at 4 weeks and HbA1c at 8 weeks were used in consideration of their reflectiveness on glucose levels for their respective periods. Analyses of the significant contributing factors for GA were performed using multiple regression analysis. Statistical Package for the Social Sciences (SPSS) for Windows Ver. 15.0 (SPSS Inc., Chicago, IL, USA) was used and p-values <0.05 were considered statistically significant.
A total of 185 ESRD patients were included in this study. Baseline demographic characteristics of the participants are summarized in Table 1. Two patients had type 1 diabetes mellitus (DM), 152 were type 2 diabetes mellitus and the other 31 were non-diabetic ESRD patients. The median duration of diabetes was 204 (10-600) months. The mean age was 60.3±13.3 years and patients in the DM group were significantly older than those in the non-diabetic group (p<0.001). Patients in the DM group had a higher incidence of cardiovascular comorbidities (p=0.03), but there were no differences in body mass index (BMI), dialysis duration, type of dialysis, and baseline laboratory data between the DM group and the non-diabetic group, except for serum glucose level. In total, 108 (58.3%) patients were on maintenance HD, and 77 patients were undergoing PD. The median durations of HD and PD were 17.0 (9.0-147.0) and 15.0 (9.0-130.0) months, respectively, and the difference was statistically insignificant (p=0.13). When examining the baseline characteristics between HD and PD groups, AG level was similar (166.3±48.6, 172.9±47.7 mg/dL, p=0.350). HD patients were older than those with PD (62.9±12.9 years vs. 56.6±13.1 years, p=0.001) and showed higher serum albumin levels (3.50±0.6 mg/dL vs. 3.21±0.5 mg/dL, p<0.001), compared to PD patients. However, there was no significant relationship between serum albumin and PD duration (r=-0.162, p=0.160) or residual renal function (r=0.086, p=0.785) (data not shown).
SMBG by capillary finger stick tests were performed approximately 74.3±7.0 times per each participant (50.5±13.0 times during the initial measurement and 22.5±4.5 times during follow-up). The mean AG calculated by AUC was 169.1±48.2 mg/dL for all subjects, 181.6±42.6 mg/dL for diabetic patients and 106.8±13.9 mg/dL for non-diabetic patients (Table 2). For the follow-up glucose measurement taken at 8 weeks, the calculated AUC was 164.3±44.1 mg/dL, 176.8±37.1 mg/dL, and 100.1±10.9 mg/dL, respectively. Correlation coefficients between the two measurements were r=0.868 (p<0.001), r=0.800 (p<0.001) and r=0.512 (p=0.01), respectively. Based on these data, the glycemic status of the recruited patients was able to be regarded as stable throughout the study period.
As expected, capillary and plasma glucose levels as well as GA or HbA1c were significantly higher in diabetic patients: serum GA and HbA1c were 21.4±6.5% and 7.2±1.1% at 4 weeks and 21.1±6.1% and 7.1±1.3% at 8 weeks, respectively. Whereas, the level of GA and HbA1c in non-diabetic patients was 10.9±2.3% and 6.0±0.9% at 4 weeks and 10.7±1.4% and 5.8±0.4% at 8 weeks. The mean GA/HbA1c ratio (mean values of GA at 4 weeks and HbA1c at 8 weeks) was 3.1±0.8 in the DM group and 1.9±0.4 in the non-DM group; all of these differences were statistically significant.
Moreover, there was a significant discrepancy in GA level dependent upon dialysis modalities. The mean GA level was 20.8±7.3% in HD patients and 17.7±5.9% in PD patients (p<0.001), probably reflecting the lower level of serum albumin in patients undergoing PD. However, no statistically significant difference was observed in HbA1c between these two groups. There were no significant intra-individual variations of GA or HbA1c during the study period.
HbA1c and GA levels were proportional to measured AG. The strength of correlation between serum GA and AG levels (r=0.70, p<0.001) was higher than that of HbA1c and AG (r=0.54, p<0.001) (Fig. 1). The correlation had a SD of prediction error of 7.68 mg/dL for GA and 15.83 mg/dL for HbA1c.
Simple linear regression models revealed a close relationship between GA and AG levels and yielded an estimating equation as follows: Mean estimated AG (eAG) (mg/dL)=4.7×GA%+73.35, R2=0.48.
As shown in Fig. 1, the scatter around the regression line was less wide and the slope of the line was significantly steeper between GA and average blood glucose in comparison to those of HbA1c, which supported the hypothesis that HbA1c levels underestimate mean blood glucose levels. Based on the above estimating equation, serum GA values were transformed into their equivalent mean eAG (Table 3). On average, each change of 10 mg/dL in AG level gave rise to 2% changes in GA values. An average blood glucose level of 155-160 mg/dL, which is equivalent to 7% of HbA1c in patients without nephropathy according to the formula of Nathan, et al.,20 was matched to a GA value of 18-19% in patients with ESRD.
The results of the correlation analyses between various clinical parameters and HbA1c, GA or GA/HbA1c ratio are shown in Table 4. Serum GA level was closely associated with the duration of diabetes (r=0.148, p=0.04) and serum albumin (r=0.260, p=0.01), whereas HbA1c level showed a significant association with only serum hemoglobin (r=0.359, p<0.001). Among the individual time points, some different findings were observed: serum GA level showed higher correlation with postprandial glucose level compared to fasting glucose level (r=0.356, p<0.001 vs. r=0.294, p=0.01). However, serum HbA1c showed the opposite results, which had a higher correlation with fasting glucose level compared to postprandial glucose level (r=0.274, p<0.001 vs. r=0.266, p<0.001). In addition, we also found a strong and significant correlation with bedtime glucose level and GA (r=0.43, p<0.001), suggesting the possible role of bedtime glucose level for determining serum GA level.
To analyze the independent contribution of clinical factors on serum GA, we constructed a series of multivariate regression models with diabetic ESRD patients (Table 5). In unadjusted analysis (Model 1), fasting and postprandial glucose levels as well as serum albumin levels and dialysis modality were significant predictors in determining serum GA level. Even after adjustment for the clinical and laboratory parameters that might influence serum GA level (Mode 4), a significant positive association of postprandial hyperglycemia (β=0.25, p=0.03) and serum albumin (β=0.17, p=0.04) with GA level was consistently observed, independent of age, BMI, serum hemoglobin, fasting glucose, duration or type of dialysis.
Our data convincingly demonstrated that the measurement of GA is a more accurate method of judging glycemic control in ESRD patients. Similar to previous reports, higher explanatory power was observed between AG and GA (r=0.70, p<0.001) compared to HbA1c (r=0.54, p<0.001). Also, we evaluated how to calculate eAG level from GA and developed an estimating equation with a regression line of eAG=4.71×GA%+73.35. Using this mathematical equation, a serum GA level of 18-19% could be matched to an eAG level of 155-160 mg/dL, and each change of 10 mg/dL in blood glucose could give rise to a 2% increase in GA level. Conceptually, converting the GA results into an equivalent "average glucose" level might help our understanding with the interpretation of GA.
To date, serum GA has been suggested as a more reliable and sensitive marker for gestational diabetes, hemolytic anemia and other diseases that shorten the lifespan of erythrocytes,24 while a number of studies have investigated the effectiveness of GA and reviewed its clinical relevance.25 One of them, Inaba, et al.16 confirmed its superiority in 1366 Japanese hemodialysis patients. In that study, HbA1c levels were significantly higher in patients without erythropoietin use, supporting that HbA1c levels are influenced by renal anemia. Peacock, et al.12 also evaluated the accuracy of GA in US diabetic hemodialysis patients and emphasized the importance of GA measurement for long-term glycemic control. However, most previous studies to date have included only hemodialysis patients; therefore, this conclusion might not be applicable to patients with increased albumin loss, as seen in nephrotic syndrome or PD, because albumin leaks into the peritoneal dialysis solution.15 To our knowledge, this is the first study where a generalized estimating equation method was evaluated in all ESRD patients including PD patients.
In our study, the mean GA/HbA1c value was 2.8±0.9 in all participants and 3.1±0.8 in the diabetic group. This result is in good agreement with previous studies and could give additional support to the usually recognized simple equation of GA=HbA1c×3. However, this simple equation might be more suitable for diabetic ESRD patients only, because the GA, HbA1c and GA/HbA1c ratio of non-diabetic ESRD patients was 10.9, 5.8 and 1.9 in our study. Namely, the simple GA/HbA1c ratio of 3.0 could not be applied to non-diabetic ESRD patients. Nevertheless, monitoring of the plasma glucose is also important in non-diabetic patients, since many patients develop diabetes after initiation of dialysis, particularly with PD.26 Therefore, compared to GA, HbA1c and GA/HbA1c ratios, serum blood glucose level could be a more useful tool for screening for the development of diabetes in non-diabetic ESRD patients.
In this study, we also found that serum GA levels were significantly influenced by serum albumin. However, there have been conflicting reports regarding the relationship between serum albumin and GA. Koga, et al.27 reported that serum GA levels could be affected by various conditions with abnormal metabolism of albumin. Under certain conditions with shortened albumin metabolism, such as hyperthyroidism, nephrotic syndrome, PD or administered glucocorticoid treatments, serum GA levels are apparently low, whereas it may be high when albumin metabolism is prolonged, as in liver cirrhosis. However, Inaba, et al.16 could not confirm this finding. In their study with HD patients, serum albumin did not act as a significant determinant of GA. We considered that the conflicting results might come from different ranges of baseline serum albumin concentrations. In our study, serum albumin level widely ranged from 2.3 to 4.9 g/dL and a substantial portion of PD patients showed significant hypoalbuminemia of less than 3.0 g/dL. Particularly, most of these patients revealed lower levels of GA. However, further large-scale studies with heterogeneous dialysis patients are needed to substantiate our observation.
Next, a slight difference in the correlations between GA levels and mean AG values according to each SMBG time point were found. Serum GA levels were more strongly correlated with postprandial glucose level, compared to fasting glucose level. In multiple regression models, only postprandial glucose level was a significant contributing factor for serum GA (p=0.02). Considering that postprandial hyperglycemia is still present in 40% of patients who had achieved their glycemic target and that the loss of postprandial glycemic control is the first step for the deterioration of glucose homeostasis in type 2 diabetes,28 our finding is not surprising and emphasizes the importance of GA monitoring.29 Furthermore, data from large studies underlined the independent role of postprandial hyperglycemia in the increasing risk of mortality.30-32 Even when HbA1c and fasting glucose levels are within normal ranges, postprandial hyperglycemia is associated with a two-fold increase in the risk of cardiovascular mortality.33 Recently, postprandial hyperglycemia has been linked to microvascular complications as well. In Shiraiwa, et al.'s34 study with Japanese type 2 diabetic patients, postprandial hyperglycemia was a strong predictor of the progression of diabetic retinopathy. Therefore, GA values, affected by postprandial glucose level, may be a useful indicator for detecting and preventing diabetic complications.
However, although GA provides a quantitative measure of recent glycemic monitoring, there are some limitations in using only GA as a measure of glycemic control, because this assay does not assess the degree of glycemic variability that a patient may experience within or between days.30 Therefore, SMBG or continuous glucose monitoring (CGM), which reflects daily fluctuations in blood glucose, is necessary.35 In this study, we used SMBG to assess day-to-day variations in average blood glucose levels.
SMBG is frequently used as an adjunctive method for accurate prediction of glycemia, because it provides a real-time measure of blood glucose levels and can detect hypoglycemia or hyperglycemia in detail.30,36 Moreover, it provides immediate feedback to patients and clinicians on therapeutic lifestyles or anti-diabetic medications, which is essential for proper diabetes management.37 Although the optimal frequency and timing of SMBG has not been determined, four to seven times per day is regarded as adequate,30,36 and we used a four-point SMBG method in this study. Considering that every patient in our study was clinically stable during the prescreening and study period, we believe that four measurements of SMBG per day were sufficient.
There are several limitations to the present study. First, we did not perform the continuous glucose monitoring system (CGMS) for measurement of individual glucose levels. CGMS has been recently introduced as a new real-time glucose measurement device. CGMS tests interstitial blood glucose levels every five minutes, and data are transferred to a computer. However, although a CGMS profile provides a more frequent measure of glycemic status, its clinical availability is limited due to high cost. In addition, the results of meta-analysis demonstrated that use of CGMS did not result in a significant reduction in HbA1c (p=0.055) or sensitivity analysis (p=0.775).37 Therefore, SMBG should be regarded as an efficient method based upon its generalizability and cost-effectiveness. Second, there was no analysis of RBC turnover or the dose of erythropoietin use in each patient. Lastly, this was a single center study and a relatively small number of patients were analyzed. Further large-scale observational studies are needed to document the conversion equation of serum GA to mean AG.
In conclusion, our study confirmed the strong relationship between GA and AG levels and provided a direct method to translate serum GA level into an easier-to-understand AG level. Using the estimating equation, an average blood glucose level of 155-160 mg/dL could be matched to a GA value of 18-19% in patients with ESRD.
Figures and Tables
Fig. 1
Correlation between AG and serum GA or HbA1c levels. The strength of correlation between serum GA and AG levels (r=0.70, p<0.001, left) was higher than that of HbA1c and AG (r=0.54, p<0.001, right). GA, glycated albumin; AG, average blood glucose.
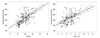
Table 4
Correlations between Clinical Parameters and GA, HbA1c or GA/HbA1c Ratio in Diabetic ESRD Patients
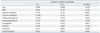
Table 5
Multiple Regression Models of GA in Diabetic ESRD Patients
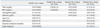
GA, glycated albumin; FBG, fasting blood glucose; PPG, postprandial glucose; BMI, body mass index; ESRD, end stage renal disease.
Model 1: unadjusted; Model 2: adjusted for fasting and postprandial glucose level; Model 3: model 2+adjusted for serum hemoglobin and albumin levels; Model 4: model 3+adjusted for age; BMI, duration and type of dialysis.
p<0.05, statistically significant.
ACKNOWLEDGEMENTS
This work was supported by a grant from the Korea Healthcare Technology R&D Project, Ministry for Health, Welfare & Family Affairs, Republic of Korea (A084001).
Notes
References
1. The Diabetes Control and Complications Trial Research Group. The effect of intensive treatment of diabetes on the development and progression of long-term complications in insulin-dependent diabetes mellitus. N Engl J Med. 1993. 329:977–986.
2. Williams ME, Lacson E Jr, Teng M, Ofsthun N, Lazarus JM. Hemodialyzed type I and type II diabetic patients in the US: characteristics, glycemic control, and survival. Kidney Int. 2006. 70:1503–1509.


3. ADVANCE Collaborative Group. Patel A, MacMahon S, Chalmers J, Neal B, Billot L, et al. Intensive blood glucose control and vascular outcomes in patients with type 2 diabetes. N Engl J Med. 2008. 358:2560–2572.


4. Genuth S, Eastman R, Kahn R, Klein R, Lachin J, Lebovitz H, et al. Implications of the United kingdom prospective diabetes study. Diabetes Care. 2003. 26:Suppl 1. S28–S32.
5. Fukuoka K, Nakao K, Morimoto H, Nakao A, Takatori Y, Arimoto K, et al. Glycated albumin levels predict long-term survival in diabetic patients undergoing haemodialysis. Nephrology (Carlton). 2008. 13:278–283.


6. Oomichi T, Emoto M, Tabata T, Morioka T, Tsujimoto Y, Tahara H, et al. Impact of glycemic control on survival of diabetic patients on chronic regular hemodialysis: a 7-year observational study. Diabetes Care. 2006. 29:1496–1500.


7. Shima K, Komatsu M, Kawahara K, Minaguchi J, Kawashima S. Stringent glycaemic control prolongs survival in diabetic patients with end-stage renal disease on haemodialysis. Nephrology (Carlton). 2010. 15:632–638.


8. Williams ME, Lacson E Jr, Wang W, Lazarus JM, Hakim R. Glycemic control and extended hemodialysis survival in patients with diabetes mellitus: comparative results of traditional and time-dependent Cox model analyses. Clin J Am Soc Nephrol. 2010. 5:1595–1601.


9. American Diabetes Association. Standards of medical care in diabetes--2007. Diabetes Care. 2007. 30:Suppl 1. S4–S41.
10. Kazempour-Ardebili S, Lecamwasam VL, Dassanyake T, Frankel AH, Tam FW, Dornhorst A, et al. Assessing glycemic control in maintenance hemodialysis patients with type 2 diabetes. Diabetes Care. 2009. 32:1137–1142.


11. Mak RH. Impact of end-stage renal disease and dialysis on glycemic control. Semin Dial. 2000. 13:4–8.
12. Peacock TP, Shihabi ZK, Bleyer AJ, Dolbare EL, Byers JR, Knovich MA, et al. Comparison of glycated albumin and hemoglobin A(1c) levels in diabetic subjects on hemodialysis. Kidney Int. 2008. 73:1062–1068.


13. Uzu T, Hatta T, Deji N, Izumiya T, Ueda H, Miyazawa I, et al. Target for glycemic control in type 2 diabetic patients on hemodialysis: effects of anemia and erythropoietin injection on hemoglobin A(1c). Ther Apher Dial. 2009. 13:89–94.


14. Freedman BI, Shenoy RN, Planer JA, Clay KD, Shihabi ZK, Burkart JM, et al. Comparison of glycated albumin and hemoglobin A1c concentrations in diabetic subjects on peritoneal and hemodialysis. Perit Dial Int. 2010. 30:72–79.


15. Chujo K, Shima K, Tada H, Oohashi T, Minakuchi J, Kawashima S. Indicators for blood glucose control in diabetics with end-stage chronic renal disease: GHb vs. glycated albumin (GA). J Med Invest. 2006. 53:223–228.


16. Inaba M, Okuno S, Kumeda Y, Yamada S, Imanishi Y, Tabata T, et al. Glycated albumin is a better glycemic indicator than glycated hemoglobin values in hemodialysis patients with diabetes: effect of anemia and erythropoietin injection. J Am Soc Nephrol. 2007. 18:896–903.


17. Okada T, Nakao T, Matsumoto H, Shino T, Nagaoka Y, Tomaru R, et al. Association between markers of glycemic control, cardiovascular complications and survival in type 2 diabetic patients with end-stage renal disease. Intern Med. 2007. 46:807–814.


18. Yamada S, Inaba M, Shidara K, Okada S, Emoto M, Ishimura E, et al. Association of glycated albumin, but not glycated hemoglobin, with peripheral vascular calcification in hemodialysis patients with type 2 diabetes. Life Sci. 2008. 83:516–519.


19. Tahara Y. Analysis of the method for conversion between levels of HbA1c and glycated albumin by linear regression analysis using a measurement error model. Diabetes Res Clin Pract. 2009. 84:224–229.


20. Nathan DM, Kuenen J, Borg R, Zheng H, Schoenfeld D, Heine RJ. A1c-Derived Average Glucose Study Group. Translating the A1C assay into estimated average glucose values. Diabetes Care. 2008. 31:1473–1478.


21. Nathan DM, Turgeon H, Regan S. Relationship between glycated haemoglobin levels and mean glucose levels over time. Diabetologia. 2007. 50:2239–2244.


22. Rohlfing CL, Wiedmeyer HM, Little RR, England JD, Tennill A, Goldstein DE. Defining the relationship between plasma glucose and HbA(1c): analysis of glucose profiles and HbA(1c) in the Diabetes Control and Complications Trial. Diabetes Care. 2002. 25:275–278.
23. Le Floch JP, Escuyer P, Baudin E, Baudon D, Perlemuter L. Blood glucose area under the curve. Methodological aspects. Diabetes Care. 1990. 13:172–175.


24. Roohk HV, Zaidi AR. A review of glycated albumin as an intermediate glycation index for controlling diabetes. J Diabetes Sci Technol. 2008. 2:1114–1121.


25. Nagayama H, Inaba M, Okabe R, Emoto M, Ishimura E, Okazaki S, et al. Glycated albumin as an improved indicator of glycemic control in hemodialysis patients with type 2 diabetes based on fasting plasma glucose and oral glucose tolerance test. Biomed Pharmacother. 2009. 63:236–240.


26. Szeto CC, Chow KM, Kwan BC, Chung KY, Leung CB, Li PK. New-onset hyperglycemia in nondiabetic chinese patients started on peritoneal dialysis. Am J Kidney Dis. 2007. 49:524–532.


27. Koga M, Murai J, Saito H, Matsumoto S, Kasayama S. Effects of thyroid hormone on serum glycated albumin levels: study on non-diabetic subjects. Diabetes Res Clin Pract. 2009. 84:163–167.


28. Monnier L, Colette C, Dunseath GJ, Owens DR. The loss of postprandial glycemic control precedes stepwise deterioration of fasting with worsening diabetes. Diabetes Care. 2007. 30:263–269.


29. Koga M, Kasayama S. Clinical impact of glycated albumin as another glycemic control marker. Endocr J. 2010. 57:751–762.


30. Dailey G. Assessing glycemic control with self-monitoring of blood glucose and hemoglobin A(1c) measurements. Mayo Clin Proc. 2007. 82:229–235.


31. The DECODE study group. European Diabetes Epidemiology Group. Diabetes Epidemiology: collaborative analysis Of Diagnostic criteria in Europe. Glucose tolerance and mortality: comparison of WHO and American Diabetes Association diagnostic criteria. Lancet. 1999. 354:617–621.
32. Daimon M, Oizumi T, Kato T. [The Funagata study--impaired glucose tolerance is a risk factor for stroke in a Japanese sample]. Nihon Rinsho. 2010. 68:843–846.
33. Gerich JE. Clinical significance, pathogenesis, and management of postprandial hyperglycemia. Arch Intern Med. 2003. 163:1306–1316.


34. Shiraiwa T, Kaneto H, Miyatsuka T, Kato K, Yamamoto K, Kawashima A, et al. Postprandial hyperglycemia is a better predictor of the progression of diabetic retinopathy than HbA1c in Japanese type 2 diabetic patients. Diabetes Care. 2005. 28:2806–2807.


35. Peel E, Douglas M, Lawton J. Self monitoring of blood glucose in type 2 diabetes: longitudinal qualitative study of patients' perspectives. BMJ. 2007. 335:493.


36. Gallichan M. Self monitoring of glucose by people with diabetes: evidence based practice. BMJ. 1997. 314:964–967.


37. Chetty VT, Almulla A, Odueyungbo A, Thabane L. The effect of continuous subcutaneous glucose monitoring (CGMS) versus intermittent whole blood finger-stick glucose monitoring (SBGM) on hemoglobin A1c (HBA1c) levels in Type I diabetic patients: a systematic review. Diabetes Res Clin Pract. 2008. 81:79–87.

