Abstract
Purpose
Despite the fact that desflurane prolongs the QTC interval in humans, little is known about the mechanisms that underlie these actions. We investigated the effects of desflurane on action potential (AP) duration and underlying electrophysiological mechanisms in rat ventricular myocytes.
Materials and Methods
Rat ventricular myocytes were enzymatically isolated and studied at room temperature. AP was measured using a current clamp technique. The effects of 6% (0.78 mM) and 12% (1.23 mM) desflurane on transient outward K+ current (Ito), sustained outward current (Isus), inward rectifier K+ current (IKI), and L-type Ca2+ current were determined using a whole cell voltage clamp.
Results
Desflurane prolonged AP duration, while the amplitude and resting membrane potential remained unchanged. Desflurane at 0.78 mM and 1.23 mM significantly reduced the peak Ito by 20±8% and 32±7%, respectively, at +60 mV. Desflurane (1.23 mM) shifted the steady-state inactivation curve in a hyperpolarizing direction and accelerated inactivation of the current. While desflurane (1.23 mM) had no effects on Isus and IKI, it reduced the L-type Ca2+ current by 40±6% (p<0.05).
Desflurane has been reported to prolong the QTc interval in healthy adults1-3 and children4 during anesthesia induction. In guinea pig ventricular myocardium in vitro,5 desflurane apparently prolonged the action potential (AP) duration by inhibiting the slowly activating delayed outward K+ current.
Cardiac AP duration is determined by balance between the inward and outward membrane currents.6,7 In most species, the transient outward K+ current (Ito) is responsible for the initial phase of repolarization, and the L-type Ca2+ current (ICa,L) is the main inward current during the plateau phase. Near the end of the ventricular AP plateau, delayed outward K+ current (IK) activation initiates repolarization, and the inward rectifier K+ current (IKI) plays an important role in generating the resting membrane potential and modulating the final repolarization phase of ventricular AP.7
The contributions of these currents vary between species and are responsible for characteristic differences in AP shape. For example, rat ventricular myocytes possess a prominent Ito, the major outward current of the repolarization phase but little IK, and have a short AP duration. In contrast, guinea pig ventricular cells lack Ito, but have a slowly activating IK, resulting in a long-lasting AP.8 While the current density of Ito in human ventricular myocytes (8.2±0.7 pA/pF at +60 mV) is two to three times smaller than that of rat myocytes, it is, nonetheless, a prominent K+ outward current in human atrial and ventricular tissue.9-11 Small changes in Ito during the early phase of AP can profoundly affect the activation of the other plateau currents, such as Ca2+ and IK, influencing AP duration. Although desflurane appears to inhibit the slowly activating IK and ICa,L in guinea pig ventricular myocytes,5 the effects of desflurane on Ito have not been identified. We explored the effects of desflurane on Ito, IKI, and ICa,L in order to further elucidate the mechanisms of prolonging APs in rat ventricular myocytes.
The animal procedures conformed to a protocol approved by the Yonsei University College of Medicine Animal Research Committee (Seoul, Korea).
The rat hearts (Sprague-Dawley, weighing 250-300 g) were quickly excised following enflurane anesthesia and retrogradely perfused using a Langendorff perfusion system for 5 min at 37℃. Perfusion was done at a rate of 7 mL/min with a modified Tyrode solution (143 mM NaCl, 5.4 mM KCl, 1.8 mM CaCl2, 0.5 mM MgCl2, 5 mM HEPES, and 5.5 mM glucose, pH 7.4). The perfusate was then switched to a nominally Ca2+-free Tyrode solution for 5 min, followed by perfusion with the same solution containing collagenase (0.4 mg/mL, Worthington type II, Worthington Biochemical Corporation, Lakewood, NJ, USA) and hyaluronidase (0.4 mg/mL, Sigma type II, Sigma-Aldrich Co., St. Louis, MO, USA). After a 10-12 min enzymatic treatment, a final perfusion was performed for 5 min with Kraftbrühe solution (10 mM taurine, 10 mM oxalic acid, 70 mM glutamic acid, 35 mM KCl, 10 mM H2PO4, 11 mM glucose, 0.5 mM EGTA, and 10 mM HEPES, pH 7.4). The ventricles were then cut off, minced with scissors, and agitated in a small beaker of Kraftbrühe solution. The resulting slurry was filtered through a 200-µm nylon mesh. The isolated ventricular cells were stored in Kraftbrühe solution for 1 hour at room temperature (21-22℃), then kept at 4℃, and used within a period of 8 hours. Only rod-shaped cells with apparent striations that remained quiescent in the solution containing 2 mM CaCl2 were used for the experiments. The whole-cell voltage-clamp studies were performed at room temperature to enhance the viability of the isolated myocytes during recording.
Fifty-seven ventricular cells from 30 rats were used in this study. Single cells were used in most experiments, but replicate experiments in single cells were carried out in part in IKI measurements. In nine cells used for measurement of IKI, we replicated measurement of IKI in six cells following measurements of Ito, because recovery of Ito following wash-out was usually complete. We confirmed that the results of IKI between three single cells and six replicate cells were consistent with no alteration of IKI by desflurane.
Isolated myocytes were allowed to settle to the bottom of a recording chamber and mounted on an inverted microscope, where bathing solutions could be exchanged. The chamber was continuously perfused at a constant rate (2 mL/min). Standard whole-cell voltage-clamp methods were used.12 In order to establish a stable baseline, an interval of 4-6 min was allowed after initiating the whole-cell recording configuration. Voltage-clamp measurements were performed using an Axopatch 200B patch clamp amplifier (Axon Instruments Inc., Foster City, CA, USA). Patch electrodes were prepared from a borosilicate glass model KIMAX-51 (American Scientific, Charlotte, NC, USA) with a two-stage micropipette puller. The pipette tips were heat-polished, using a microforge, giving a typical resistance of 2-3 MΩ when filled with an internal solution. Data acquisition was performed using a pCLAMP system version 6.0 (Axon Instruments Inc., Foster city, CA, USA).
The APs were elicited in current-clamp mode by 5-ms, 800-pA current injections at a frequency of 1 Hz.
To examine the Ito, the cells were depolarized to -40 mV for 50 ms from a holding potential of -80 mV to inactivate the Na+ current, then depolarized to test potentials up to +60 mV in 10-mV increments for 300 ms. To obtain more information about the possible mechanism of the desflurane-induced voltage blockade of K+ currents, the voltage dependence of steady-state inactivation was determined using a standard double-pulse protocol. The membrane potential was initially clamped at -80 mV and stepped to different potentials ranging from -100 to 0 mV in 10-mV increments for 500 ms followed by a 200-ms test pulse to +80 mV. The voltage clamp protocol was repeated every 2 s. Steady state inactivation data were fitted to a Boltzmann distribution of the following equation: I/Imax=I/{1+exp [(V-V1/2)]/κ}, where Imax is the maximal current, V1/2 is the membrane potential producing 50% inactivation, and κ is the slope factor.
To determine whether the acceleration of Ito inactivation in the presence of desflurane could be associated with time-dependent closure of the open channels, the magnitude of current inhibition at various times after the initiation of the depolarizing pulse was determined at a membrane potential of +60 mV. The outward current in the presence of each desflurane concentration, normalized to the control outward current, was plotted as a function of time after the start of depolarization. Because inactivation increases exponentially during depolarization, the equation [(Icontrol-Idrug)/Icontrol=a1×(1-e-t/τ)+a2] was used to describe the rate of inhibition of Ito at each desflurane concentration where τ is the time constant of inhibition development. (Icontrol-Idrug)/Icontrol equals the amount of inhibition at time t, a1 equals the maximum inhibition at a drug concentration, and a2 equals the zero intercept. The extrapolation to "time zero" shows the proportion of channels inhibited before the start of the pulse, which is an indirect measure of the proportion of channels inhibited in the resting state.13
The outward currents activated by depolarizing voltage steps in rat ventricular myocytes consist of a rapidly inactivating component, Ito, and a non-inactivating, sustained component, Isus.14,15 The sustained outward current (Isus) was recorded with the same voltage protocol after addition of 5 mM 4-aminopyridine in a modified Tyrode solution, which preferentially blocks Ito. Isus in adult rat ventricular myocytes is responsible for the slower phase of AP repolarization back to the resting membrane potential.16 The Isus, comprised of IK and a small time-independent outward current,14 remain in the presence of 4-aminopyridine.
IKI was measured following step depolarizations from -140 to -40 mV from a holding potential of -40 mV in 20 mV increments, using a 200-ms pulse applied at 5-s intervals.
Voltage-dependent ICa,L was evoked by step depolarization for 500 ms from a holding potential of -40 mV to +0 mV in one step at a frequency of 0.1 Hz.
After baseline measurements, the myocytes were exposed to 6% or 12% desflurane for 2-3 min, and recovery responses were measured after washing for 2-3 min to remove the drug. Applying desflurane for 2 min produced a stable and consistent effect in the pilot experiments.
Before establishing the whole-cell recording configuration, modified Tyrode solution (140 mM NaCl, 5.4 mM KCl, 1 mM CaCl2, 1 mM MgCl2, 5 mM HEPES, 10 mM glucose, adjusted to pH 7.4 with 1 N NaOH) was used as an external bathing solution. For K+ current measurements, a patch pipette solution (20 mM KCl, 110 mM K-aspartate, 10 mM EGTA, 10 mM HEPES, 1 mM MgCl2, 5 mM K2ATP, 1 mM CaCl2, 10 mM NaCl, adjusted to pH 7.2 with 3 N KOH) was used. To measure Isus, 5 mM 4-aminopyridine was added to the modified Tyrode solution and the pH corrected to 7.4 with HCl. In order to eliminate any confounding Ca2+ current from the K+ current studies, 0.2 mM CdCl2 was added to the external solution after establishing the whole-cell voltage-clamp.
The inward Ca2+ current was measured using a patch pipette solution (30 mM CsCl, 100 mM aspartic acid, 100 mM CsOH, 10 mM BAPTA, 10 mM HEPES, 10 mM phosphocreatine, 1 mM Na2GTP, 5 mM Na2ATP, 10 mM glucose, 2 mM MgCl2, adjusted to pH 7.2 with 1 M CsOH). Once whole-cell recording was achieved, the bathing solution was exchanged for a solution containing 140 mM NaCl, 5.4 mM CsCl, 2 mM CaCl2, 1 mM MgCl2, 10 mM HEPES, adjusted to pH 7.4 with 1 M CsOH. Desflurane was purchased from the Baxter Healthcare Corporation (Deerfield, IL, USA). All other chemicals were purchased from Sigma-Aldrich Co.
Before perfusion, the external bathing solution was equilibrated in a reservoir with desflurane, which was supplied by a gas dispersion tube connected to an outlet of the desflurane vaporizer for 15 min. One hundred percent O2 (flow rate: 0.2 L/min) was passed through a desflurane vaporizer (Devapor Type M32600, Dräger AG, Lübeck, Germany). The end-tidal concentrations of desflurane were monitored using a calibrated gas analyzer (Capnomac, Datex, Helsinki, Finland). Six and 12% desflurane corresponded to 0.78±0.05 mM (n=3) and 1.23±0.03 mM (n=3) respectively. Given a Tyrode solution/gas partition coefficient of 0.27 at 22℃,17 the aforementioned desflurane concentrations are equivalent to gas phase concentrations of 7.0% and 11.0%, respectively.
One-way repeated measures of ANOVA followed by the Student-Newman-Keuls test were applied to test for significant differences among the control, drug application, and washout. Unpaired t-tests were used to compare differences in Ito currents between 6% and 12% desflurane. All values are expressed as mean±SD. A p value of less than 0.05 was considered significant. In the case of results that were not normally distributed, ANOVA of ranks was used, followed by the Student-Newman-Keuls test. In this case, results are expressed as median (IQR). Statistical comparisons were conducted with Sigmastat (SPSS Inc., Chicago, IL, USA) and all figures were prepared using Origin (Microcal Software Inc., Northampton, MA, USA).
Fig. 1 shows the concentration-dependent prolongation of AP in a rat ventricular myocyte. APD50 and APD90 was prolonged by 42±26% (p<0.05) and 21±13% (p<0.05), respectively, in the presence of 1.23 mM desflurane in 10 cells. AP amplitude and resting membrane potential remained unaltered (Table 1). AP duration returned to the baseline following washout.
At +60 mV, 0.78 mM desflurane reduced the control peak currents of Ito (3.17±0.86 nA) by 20±8% in 10 cells (p<0.05), and the plateau currents measured at the end of depolarization [1.12 (0.92-1.35) nA] by 9% (8.8-9.6%) (p<0.05). Desflurane 1.23 mM reduced the control peak currents of Ito (3.27±1.03 nA) by 32±7% in 10 cells (p<0.05) and the plateau currents (1.37±0.32 nA)] by 13±14% (p<0.05) (Fig. 2). Both peak and plateau currents were completely recovered after wash.
Under control conditions, the voltage required for half-inactivation (V1/2) and slope factor (κ) for Ito were -29.3±0.6 mV and 7.8±0.5 mV, respectively, in 10 cells. Desflurane 1.23 mM significantly shifted the steady-state inactivation curve in a hyperpolarizing direction (V1/2=-34.3±0.9 mV, κ=9.95±0.8) (Fig. 3).
Fig. 2A shows that desflurane accelerates decay of the current during the pulse, so we evaluated the effect of desflurane on Ito inactivation kinetics. Individual current records, evoked by a test pulse to +60 mV, were fitted to an exponential function. Unlike the control currents in which the decay could be fitted by a single exponential, inactivation of Ito in the presence of desflurane was better described by a double exponential. Fig. 4A and B show the quality of fit of the single and double exponential functions to the inactivation phase of Ito under the control and 1.23 mM desflurane conditions. The control τ1 (26±10 ms) was reduced to 9±4 ms by 1.23 mM desflurane in 7 cells (p<0.05). Inactivation kinetics returned to baseline values following washout (24±8 ms) (Fig. 4C).
Fig. 5 shows inhibition of Ito between the control and in the presence of 0.78 mM and 1.23 mM desflurane during the first 60 ms of depolarization. Inhibition induced by desflurane was an exponential function of time. Both the magnitude of the maximum inhibition and its rate of development appeared to be concentration-dependent. The maximum amount of inhibition by 0.78 mM and 1.23 mM desflurane were 0.58±0.11 and 0.82±0.09 in 8 cells, respectively. The time constants for the increased decline in current were 32±13 ms and 12±7 ms, respectively, for 0.78 mM and 1.23 mM desflurane.
Desflurane (1.23 mM) had no effect on Isus (Fig. 6). Before desflurane exposure, the baseline Isus values at the end of the plateau at +60 mV were 1.12±0.24 nA in six cells.
At membrane potential ranging from -140 mV to -40 mV, 1.23 mM desflurane did not alter IKI in nine cells (Fig. 7). IKI was measured at the end of the pulse duration. Prior to desflurane exposure, the baseline values during test potential of -140 mV were -2.52±0.73 nA.
At +10 mV, 1.23 mM desflurane reduced the ICa,L by 40±6% in 8 cells (p<0.05) (Fig. 8). The effects of desflurane on ICa,L were completely reversed following washout. The baseline values before exposure to 1.23 mM desflurane were -2.24±0.83 nA.
Our results showed that clinically relevant desflurane concentrations prolonged AP duration in isolated rat ventricular myocytes and significantly inhibited Ito and ICa,L. Desflurane significantly accelerated current inactivation of Ito and shifted the steady-state inactivation curve.
Desflurane prolongs ventricular AP duration in guinea pigs,5 which supports our results in rats. Considering that the QT interval is in accordance with AP duration in the ventricular myocardium, the prolonged AP duration in guinea pig5 and rat ventricular myocytes in our results may support clinical evidence of a prolonged QTc interval in healthy adults1-3 and children4 during induction of anesthesia with desflurane.
In rat ventricular myocytes, the cardiac voltage-activated K+ current consists of Ito, which is sensitive to 4-aminopyridine, and IK, which is sensitive to tetraethylammonium.16 IK is the prominent voltage-activated outward K+ current in frog18 and guinea pig ventricular myocytes.19 However, in rat,20 dog,21 rabbit,22 and human myocytes,10 Ito is prominent and plays a significant role in the repolarization phase, particularly in the early phase of AP. In several cardiac tissues, two types of Ito have been identified; one is voltage- and Ca2+-in dependent, and the other is Ca2+ -dependent.23,24 In rat ventricular myocytes, only Ca2+-independent Ito has been identified.25 The Ca2+-independent Ito carried predominantly by K+ ions has been suggested to be a major determinant of cardiac AP duration because of its size and pronounced frequency dependence.24
Prolonged AP duration caused by inhibition of Ito has been demonstrated with tedisamil, an Ito and IK blocker in mammalian cardiac cells, in isolated rat ventricular myocytes26 and in rat papillary muscles.27 The reduction of Ito by approximately 20% by 0.78 mM desflurane appears to be a fractional reduction of the current. However, considering the large current density of Ito in rat ventricular myocytes, 19.9±2.8 pA/pF at +60 mV,9 desflurane would have a greater effect on the AP plateau phase, prolonging AP.
The results of the present study show that desflurane not only decreased the peak Ito but also accelerated the current decay during a sustained depolarization. With sustained depolarization, there was an apparent anesthetic-induced acceleration of the current inactivation that followed an exponential time course. This channel inhibition could be due to an acceleration of the inactivation process involving the channel molecular rearrangement to accelerate the closing of the gate, or might represent desflurane binding and blockade of the open channel that follows an exponential time course during continued depolarization. Nevertheless, the extrapolation to time zero shows that desflurane does not significantly inhibit Ito before its activation at the onset of depolarization. The hyperpolarizing shift of the inactivation curve indicates that desflurane does depress Ito in part by reducing the availability of Ito channels.
Isus contributes to the overall repolarization process in rat ventricular myocytes. In the present study, Isus was not affected by desflurane, whereas desflurane 1.23 mM significantly depressed IK in guinea pig ventricular cells, by approximately 40%.5 Although IK suppression by desflurane in guinea pig ventricular myocytes is mainly responsible for AP prolongation,5 Isus is not likely to contribute to prolongation of AP duration by desflurane in rat ventricular myocytes. Presumably, the subtle change in Isus by desflurane may be attributed to relative variations of Ito and IK (Ito/IK) among rat ventricular cells,16 significant inhibition of IK by 4-aminopyridine at concentrations above 1 mM,14 and/or species differences.
The IKI is the primary current that maintains stable cardiac resting membrane potential near the K+ equilibrium potential. Thus, inhibition of IKI can result in diastolic depolarization, which can increase cardiac excitability28 and lead to dysarrythmias and abnormal automaticity.29 In our results, desflurane did not alter the inward component of IKI, similar to the modest effects on inward and outward components of IKI by desflurane in guinea pig ventricular myocytes.5 The lack of change in the resting membrane potential by desflurane in our results may reflect the lack of effect on IKI. In the present study at steady state inactivation, approximately 80% of the Ito channels appeared to be available for activation at -40 mV. This indicates that the outward component of IKI above -40 mV includes Ito so that the outward component of IKI above -40 mV can be influenced by Ito in this preparation.
Even with a prolonged AP duration, our whole-cell voltage-clamp studies revealed a reduction of peak ICa,L. Indirect evidence for inhibition of Ca2+ entry by desflurane has been proposed in rat isolated myocardial preparations,30 and suppression of ICa,L by desflurane has been reported in guinea pig ventricular myocytes.5 Inhibition of ICa,L would shorten AP duration, but reduction of Ito appears to have a greater effect, resulting in lengthening of AP duration.
In human ventricular myocytes, although the current density of Ito (8.2±0.7 pA/pF at +60 mV) has been reported to be two or three times smaller than that of rat myocytes, it is, nonetheless, a major outward current.9-11 Small changes in Ito during the early phase of AP in human ventricular myocytes can profoundly affect activation of other plateau currents, such as Ca2+ and delayed outward K+ currents, influencing both AP duration and Ca2+ influx. The overall results of this study demonstrate that, at equivalent anesthetic concentrations, the actions of desflurane are almost identical to sevoflurane.31 The similarity in these basic electrophysiological effects is paralleled by the similarity in clinically observed changes.1-3
In conclusion, clinically relevant desflurane concentrations appear to prolong AP duration in rat ventricular myocytes by suppressing Ito. Considering the similar voltage- and time-dependence of Ito between human and rat heart cells,9 desflurane suppression of Ito may partially account for clinical observations of QTc prolongation in humans.
Figures and Tables
Fig. 1
Effect of desflurane (DES) on action potential duration in a rat ventricular myocyte. C, control.
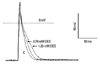
Fig. 2
Effects of desflurane (DES) on transient outward K+ current (Ito) in rat ventricular myocytes. (A) Recordings of control, 1.23 mM DES, and recovery in rat ventricular myocytes. Closed and open circles indicate the peak current of Ito at every potential in the control and 1.23 mM DES. Triangles represent the plateau currents at the end of the test pulses before (closed) and after (open) applying of 1.23 mM DES. Squares represent the peak (closed) and plateau (open) currents after wash. The currents shown are for depolarization from -40 to +60 mV in 10 mV steps. (B) Current-voltage relations of Ito in 10 cells. Closed and open circles indicate the peak current of Ito at every potential in the control and 1.23 mM DES. Triangles represent the plateau currents at the end of the test pulses before (closed) and after (open) application of 1.23 mM DES. (C) Effects of 0.78 mM and 1.23 mM DES on the amplitude of the peak Ito at +60 mV in 10 cells. *p<0.05 versus control. †p<0.05 versus 0.78 mM DES. Error bars indicate mean±SD.
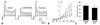
Fig. 3
Steady state inactivation curves of transient outward K+ currents under control conditions and 1.23 mM desflurane (DES). Closed and open circles indicate control and 1.23 mM DES, respectively. Data are presented as mean±SD for 10 cells and were fitted with the Boltzman function. The half inactivations (V1/2) of the control and 1.23 mM DES were -29.3±0.6 mV and -34.3±0.9 mV, respectively.
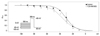
Fig. 4
Effect of desflurane (DES) on current inactivation. The inactivation phase of transient outward K+ current (Ito) were best fitted by single and double exponential functions under control conditions (A) and 1.23 mM DES (B), respectively, in a rat ventricular myocyte. Mean values of T1 in 7 cells under control conditions, 1.23 mM DES, and after washout (C). *p<0.05 versus control and recovery. Error bars indicate mean±SD.
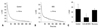
Fig. 5
Time-dependent inhibition of transient outward K+ currents (Ito) by desflurane. Time course of the development of inhibition by 0.78 mM and 1.23 mM desflurane in each of the 8 cells during a depolarizing pulse to +60 mV from a holding potential of -40 mV. The reduction of Ito in the presence of desflurane is expressed as a proportion of the control current at any given time after the start of the depolarizing pulse.
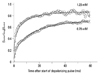
Fig. 6
The effects of desflurane (DES) on sustained outward currents (Isus) in a rat ventricular myocyte. (A) A control recording of transient outward K+ currents (Ito). (B) Isus obtained after application of 5 mM 4-aminopyridine (4-AP), which preferentially blocks Ito. (C) 1.23 mM DES exposure after application of 5 mM 4-aminopyridine.
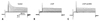
Fig. 7
Effect of desflurane (DES) on the inward rectifier K+ current (IKI) in rat ventricular myocytes. (A) Closed and open circles indicate control and 1.23 mM DES in a rat ventricular myocyte, respectively, at a membrane potential of -140 mV. The dotted line indicates recovery after wash. (B) Current-voltage relations for IKI before and after applying 1.23 mM DES in 9 cells. Closed and open circles indicate control and 1.23 mM DES, respectively. Error bars indicate mean±SD.
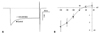
Fig. 8
Effect of desflurane (DES) on L-type Ca2+ current (ICa,L). (A) A representative example of the effect of DES on ICa,L in a rat ventricular myocyte. The open circles represent the peak of an individual current record. The horizontal bar indicates the period of DES application. (B) An example of individual currents recorded in the presence of 1.23 mM DES. Closed and open circles indicate control and 1.23 mM DES, respectively. The dotted line indicates recovery after wash.
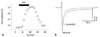
ACKNOWLEDGEMENTS
The authors would like to thank Carl Lynch III, MD., Ph.D., Professor, Department of Anesthesiology, University of Virginia Health system, Charlottesville, Virginia, USA for reading and commenting on the manuscript.
This work was supported by a Faculty Research Grant (6-2007-0172), 2007, Yonsei University College of Medicine, Seoul, Korea.
References
1. Yildirim H, Adanir T, Atay A, Katirciolu K, Savaci S. The effects of sevoflurane, isoflurane and desflurane on QT interval of the ECG. Eur J Anaesthesiol. 2004. 21:566–570.


2. Silay E, Kati I, Tekin M, Guler N, Huseyinoglu UA, Coskuner I, et al. Comparison of the effects of desflurane and sevoflurane on the QTc interval and QT dispersion. Acta Cardiol. 2005. 60:459–464.


3. Owczuk R, Wujtewicz MA, Sawicka W, Lasek J, Wujtewicz M. The Influence of desflurane on QTc interval. Anesth Analg. 2005. 101:419–422.


4. Aypar E, Karagoz AH, Ozer S, Celiker A, Ocal T. The effects of sevoflurane and desflurane anesthesia on QTc interval and cardiac rhythm in children. Paediatr Anaesth. 2007. 17:563–567.


5. Park WK, Kim MH, Ahn DS, Chae JE, Jee YS, Chung N, et al. Myocardial depressant effects of desflurane: mechanical and electrophysiologic actions in vitro. Anesthesiology. 2007. 106:956–966.
7. Coraboeuf E. Ionic basis of electrical activity in cardiac tissues. Am J Physiol. 1978. 234:H101–H116.


8. Rees S, Curtis MJ. Which cardiac potassium channel subtype is the preferable target for suppression of ventricular arrhythmias? Pharmacol Ther. 1996. 69:199–217.


9. Wettwer E, Amos G, Gath J, Zerkowski HR, Reidemeister JC, Ravens U. Transient outward current in human and rat ventricular myocytes. Cardiovasc Res. 1993. 27:1662–1669.


10. Näbauer M, Beuckelmann DJ, Erdmann E. Characteristics of transient outward current in human ventricular myocytes from patients with terminal heart failure. Circ Res. 1993. 73:386–394.


11. Nánási PP, Varró A, Lathrop DA. Ionic currents in ventricular myocytes isolated from the heart of a patient with idiopathic cardiomyopathy. Cardioscience. 1992. 3:85–89.
12. Hamill OP, Marty A, Neher E, Sakmann B, Sigworth FJ. Improved patch-clamp techniques for high-resolution current recording from cells and cell-free membrane patches. Pflugers Arch. 1981. 391:85–100.


13. Castle NA. Bupivacaine inhibits the transient outward K+ current but not the inward rectifier in rat ventricular myocytes. J Pharmacol Exp Ther. 1990. 255:1038–1046.
14. Slawsky MT, Castle NA. K+ channel blocking actions of flecainide compared with those of propafenone and quinidine in adult rat ventricular myocytes. J Pharmacol Exp Ther. 1994. 269:66–74.
15. He J, Kargacin ME, Kargacin GJ, Ward CA. Tamoxifen inhibits Na+ and K+ currents in rat ventricular myocytes. Am J Physiol Heart Circ Physiol. 2003. 285:H661–H668.
16. Apkon M, Nerbonne JM. Characterization of two distinct depolarization-activated K+ currents in isolated adult rat ventricular myocytes. J Gen Physiol. 1991. 97:973–1011.


17. Hönemann CW, Washington J, Hönemann MC, Nietgen GW, Durieux ME. Partition coefficients of volatile anesthetics in aqueous electrolyte solutions at various temperatures. Anesthesiology. 1998. 89:1032–1035.


18. Hume JR, Giles W. Ionic currents in single isolated bullfrog atrial cells. J Gen Physiol. 1983. 81:153–194.


19. Hume JR, Uehara A. Ionic basis of the different action potential configurations of single guinea-pig atrial and ventricular myocytes. J Physiol. 1985. 368:525–544.


20. Josephson IR, Sanchez-Chapula J, Brown AM. Early outward current in rat single ventricular cells. Circ Res. 1984. 54:157–162.


21. Litovsky SH, Antzelevitch C. Transient outward current prominent in canine ventricular epicardium but not endocardium. Circ Res. 1988. 62:116–126.


22. Giles W, Shimoni Y. Comparison of sodium-calcium exchanger and transient inward currents in single cells from rabbit ventricle. J Physiol. 1989. 417:465–481.


23. Coraboeuf E, Carmeliet E. Existence of two transient outward currents in sheep cardiac Purkinje fibers. Pflugers Arch. 1982. 392:352–359.


24. Escande D, Coulombe A, Faivre JF, Deroubaix E, Coraboeuf E. Two types of transient outward currents in adult human atrial cells. Am J Physiol. 1987. 252:H142–H148.


25. Dukes ID, Morad M. The transient K+ current in rat ventricular myocytes: evaluation of its Ca2+ and Na+ dependence. J Physiol. 1991. 435:395–420.


26. Dukes ID, Cleemann L, Morad M. Tedisamil blocks the transient and delayed rectifier K+ currents in mammalian cardiac and glial cells. J Pharmacol Exp Ther. 1990. 254:560–569.
27. Walker MJ, Beatch GN. Electrically induced arrhythmias in the rat. Proc West Pharmacol Soc. 1988. 31:167–170.
28. Nichols CG, Makhina EN, Pearson WL, Sha Q, Lopatin AN. Inward rectification and implications for cardiac excitability. Circ Res. 1996. 78:1–7.


29. Task Force of the Working Group on Arrhythmias of the European Society of Cardiology. The Sicilian gambit. A new approach to the classification of antiarrhythmic drugs based on their actions on arrhythmogenic mechanisms. Circulation. 1991. 84:1831–1851.
30. Gueugniaud PY, Hanouz JL, Vivien B, Lecarpentier Y, Coriat P, Riou B. Effects of desflurane in rat myocardium: comparison with isoflurane and halothane. Anesthesiology. 1997. 87:599–609.