Abstract
Purpose
This study was undertaken to investigate the effects of gamma linolenic acid (GLA) on inflammation and extracellular matrix (ECM) synthesis in mesangial and tubular epithelial cells under diabetic conditions.
Materials and Methods
Sprague-Dawley rats were intraperitoneally injected with either a diluent [n=16, control (C)] or streptozotocin [n=16, diabetes (DM)], and eight rats each from the control and diabetic groups were treated with evening primrose oil by gavage for three months. Rat mesangial cells and NRK-52E cells were exposed to medium containing 5.6 mM glucose and 30 mM glucose (HG), with or without GLA (10 or 100 µM). Intercellular adhesion molecule-1 (ICAM-1), monocyte chemoattractant protein-1 (MCP-1), and fibronectin (FN) mRNA and protein expression levels were evaluated.
Results
Twenty-four-hour urinary albumin excretion was significantly increased in DM compared to C rats, and GLA treatment significantly reduced albuminuria in DM rats. ICAM-1, MCP-1, FN mRNA and protein expression levels were significantly higher in DM than in C kidneys, and these increases were significantly abrogated by GLA treatment. In vitro, GLA significantly inhibited increases in MCP-1 mRNA expression and protein levels under high glucose conditions in HG-stimulated mesangial and tubular epithelial cells (p<0.05, respectively). ICAM-1 and FN expression showed a similar pattern to the expression of MCP-1.
Diabetic nephropathy, the leading cause of end-stage renal disease worldwide, is characterized pathologically by cellular hypertrophy and increased extracellular matrix (ECM) accumulation.1 The ECM accumulation in diabetic nephropathy results in mesangial expansion, tubulointerstitial fibrosis, and irreversible deterioration of renal function.2 Even though previous studies have shown that ECM accumulation under diabetic conditions is attributable to hyperglycemia per se, advanced glycation end-products, hemodynamic changes, and local growth factors such as angiotensin II (AII) and transforming growth factor (TGF)-β1,3 the precise molecular and cellular mechanisms responsible for this have yet to be resolved.
Recently, accumulating evidence has suggested that the inflammatory process also plays an important role in the pathogenesis of diabetic nephropathy.4 Infiltration of inflammatory cells in glomeruli and renal tubulointerstitium is commonly seen in both human diabetic patients and experimental diabetic animals.5,6 In addition, intracellular adhesion molecule-1 (ICAM-1) and monocyte chemoattractant protein-1 (MCP-1), which mediates the recruitment and infiltration of monocytes/macrophages, have been shown to be involved in the pathogenesis of diabetic nephropathy.7,8 Based on these findings, modulation of the inflammatory process is considered to be a potential means of preventing the development and progression of diabetic nephropathy, and some immunosuppressive agents and anti-inflammatory drugs have been found to be beneficial in diabetic nephropathy.9,10 Nevertheless, chronic use of these drugs in the clinical field is not appropriate due to many systemic side effects. Therefore, safe agents for chronic treatment of diabetic nephropathy are undoubtedly needed.
Polyunsaturated fatty acids (PUFAs), which exist in high concentrations in cell membranes as structural phospholipids, are essential to cell integrity and viability.11,12 There are two classes of PUFAs: ω-3 and ω-6, designated according to their carbon ring structure.12 γ-linolenic acid (GLA), a member of ω-6 PUFA, is produced from linoleic acid by the enzyme δ-6 desaturase, and is elongated to dihomogamma linolenic acid (DGLA).11-13 In a previous study, GLA was shown to abrogate renal fibrosis in a 5/6 nephrectomy model,14 and other investigations have demonstrated that GLA treatment improved autoimmune diseases and diabetic neuropathy via an anti-inflammatory mechanism.15,16 As mentioned earlier, since the inflammatory process is also involved in the pathogenesis of diabetic nephropathy, there is a possibility that GLA may ameliorate diabetic nephropathy, but this has never been explored.
In this study, therefore, we investigated the effects of GLA in experimental diabetic kidneys as well as in high glucose-stimulated mesangial cells and tubular epithelial cells in regards to inflammation and ECM synthesis.
All animal studies were conducted using an approved protocol the committee for the care and use of laboratory animals of Yonsei University College of Medicine. Sprague-Dawley rats weighing 250-280 g were intraperitoneally injected with either a diluent [n=16, control (C)] or 65 mg/kg streptozotocin [n=16, diabetes (DM)]. Diabetes was confirmed by tail vein blood glucose levels on the third post-injection day. After confirming diabetes, eight rats each from the C and DM groups were treated with 450 mg/kg/day of evening primrose oil (EPO, a generous gift from Dalim Biotech, Seoul, Korea) by gavage (C+GLA or DM+ GLA) for three months. EPO contained 8-10% GLA, and the amount of EPO used in this study provided an approximate GLA dose of 40 mg/kg/day. Rats were housed in a temperature-controlled room and given free access to water and standard laboratory chow during the three-month study period.
Body weight and serum glucose level were checked monthly, and kidney weight and 24-hour urinary albumin excretion were checked at the time of sacrifice. Blood glucose was measured by a glucometer, and 24-hour urinary albumin excretion was determined by enzyme-linked immunosorbent assay (ELISA) (Nephrat II, Exocell, Inc., Philadelphia, PA, USA).
Primary culture of glomerular mesangial cells was performed as previously described.17 Identification of mesangial cells was performed by their characteristic stellate appearance in culture and confirmed by immunofluorescent microscopy for the presence of actin, myosin, and Thy-1 antigen, as well as the absence of factor VIII and cytokeratin (Synbiotics, San Diego, CA, USA). Mesangial and NRK-52E cells and immortalized rat tubular epithelial cells, were maintained, respectively, in RPMI 1640 and DMEM medium supplemented with 5% fetal bovine serum, 100 U/mL penicillin, 100 mg/mL streptomycin, 26 mM NaHCO3, and grown at 37℃ in humidified 5% CO2 in air. Subconfluent mesangial cells and NRK-52E cells were serum restricted for 24 hours, after which the medium was replaced by serum-free medium containing 5.6 mM glucose (NG), NG+24.4 mM mannitol (NG+M), or 30 mM glucose (HG) with or without 10 or 100 µM GLA (Sigma Chemical Co., St. Louis, MO, USA). In addition, both of the cells were treated by 10 or 100 µM linoleic acid (Sigma Chemical Co., St. Louis, MO, USA). At 24 hours after the media change, the cells were harvested and conditioned culture media were collected.
Total RNA from the renal cortical tissue was extracted as previously described.17 Briefly, 100 µL of RNA STAT-60 reagent (Tel-Test, Inc., Friendswood, TX, USA) was added to the renal cortical tissues, which were lysed by freezing and thawing three times. Another 700 µL of RNA STAT-60 reagent was then added and the mixture was vortexed and stored for five minutes at room temperature. Next, 160 µL of chloroform was added and the mixture was shaken vigorously for 30 seconds. After three minutes, the mixture was centrifuged at 12000×g for 15 minutes at 4℃ and the upper aqueous phase containing the extracted RNA was transferred to a new tube. RNA was precipitated from the aqueous phase by adding 400 µL of isopropanol and then pelleted by centrifugation at 12000×g for 30 minutes at 4℃. The RNA precipitate was washed with 70% ice-ethanol, dried using a Speed Vac, and dissolved in DEPC-treated distilled water. RNA yield and quality were assessed based on spectrophotometric measurements at wavelengths of 260 and 280 nm. Total RNA from the mesangial and NRK-52E cells was similarly extracted.
First strand cDNA was synthesized using a Boehringer Mannheim cDNA synthesis kit (Boehringer Mannheim GmbH, Mannheim, Germany). Two µg of total RNA extracted from the renal cortex and cultured cells were reverse transcribed using 10 µM random hexanucleotide primer, 1 mM dNTP, 8 mM MgCl2, 30 mM KCl, 50 mM Tris-HCl, pH 8.5, 0.2 mM dithiothreitol, 25 U RNase inhibitor, and 40 U AMV reverse transcriptase. The mixture was incubated at 30℃ for 10 minutes and 42℃ for one hour, followed by inactivation of the enzyme at 99℃ for five minutes.
The primers used for ICAM-1, MCP-1, fibronectin, and 18s amplification were as follows: ICAM-1, sense 5'-AGGTATCCATCCATCCCAC-3', antisense 5'-GCCGAGGTTCTCGTCTTC-3'; MCP-1, sense 5'-TCTCTTCCTCCACCACTATGCA-3', antisense 5'-GGCTGAGACAGCACGTGGAT-3'; fibronectin, sense 5'-TGACAACTGCCGTAGACCTGG-3', antisense 5'-TACTGGTTGTAGGTGTGG. CCG-3'; and 18s, sense 5'-AGTCCCTGCCCTTTGTACACA-3', antisense 5'-GATCCGAGGGCCTCACTAAAC-3'. cDNAs from 25 ng RNA of the renal cortical tissue or cultured cells per reaction tube were used for amplification.
Using the ABI PRISM® 7700 Sequence Detection System (Applied Biosystems, Foster City, CA, USA), PCR was performed with a total volume of 20 µL in each well, containing 10 µL of SYBR Green® PCR Master Mix (Applied Biosystems), 5 µL of cDNA, and 5 pM sense and antisense primers. Primer concentrations were determined by preliminary experiments that analyzed the optimal concentrations of each primer. Each sample was run in triplicate in separate tubes. The PCR conditions were as follows: 35 cycles of denaturation at 94.5℃ for 30 sec, annealing at 60℃ for 30 sec, and extension at 72℃ for one minute. Initial heating at 95℃ for nine minutes and final extension at 72℃ for seven minutes were performed for all PCRs.
After real-time PCR, the temperature was increased from 60 to 95℃ at a rate of 2℃/min to construct a melting curve. A control without cDNA was run in parallel with each assay. The cDNA content of each specimen was determined using a comparative CT method with 2-ΔΔT. The results are given as relative expressions of ICAM-1, MCP-1, and fibronectin normalized to the expression of the 18s housekeeping gene.
The renal cortical tissue and cultured cells harvested from plates were lysed in sodium dodecyl sulfate (SDS) sample buffer [2% sodium dodecyl sulfate, 10 mM Tris-HCl, pH 6.8, 10% (vol/vol) glycerol], treated with Laemmli sample buffer, heated at 100℃ for five minutes, and electrophoresed in an 8% acrylamide denaturing SDS-polyacrylamide gel. Proteins were then transferred to a Hybond-ECL membrane using a Hoeffer semidry blotting apparatus (Hoeffer Instruments, San Francisco, CA, USA). The membrane was then incubated in blocking buffer A (1×PBS, 0.1% Tween-20, and 8% nonfat milk) at room temperature for one hour, followed by an overnight incubation at 4℃ in a 1 : 1000 dilution of polyclonal antibodies to ICAM-1 (R&D systems, Minneapolis, MN, USA), fibronectin (Santa Cruz Biotechnology, Inc., Santa Cruz, CA, USA), or β-actin (Sigma Chemical Co., St. Louis, MO, USA). The membrane was then washed once for 15 minutes and twice for five minutes in 1×PBS with 0.1% Tween-20. Next, the membrane was incubated in buffer A containing a 1 : 1000 dilution of horseradish peroxidase-linked donkey anti-goat IgG (Amersham Life Science, Inc., Arlington Heights, IL, USA). The washes were repeated, and the membrane was developed with a chemiluminescent agent (ECL; Amersham Life Science, Inc., Arlington Heights, IL, USA). The band densities were measured using TINA image software (Raytest, Straubenhardt, Germany).
The levels of MCP-1 in the renal cortical tissue and culture media were determined using a commercial ELISA kit (BD Biosciences, San Diego, CA, USA) according to the manufacturer's protocol. The kit for rat MCP-1 was species-specific and sensitive up to 750 pg/mL. All concentrations of MCP-1 were normalized to the total protein amount.
For immunohistochemical staining, slices of kidney were snap-frozen in optimal cutting temperature solution and 4 µm sections of tissues were utilized. Slides were fixed in acetone for 10 minutes, air dried at room temperature for 10 minutes, and blocked with 10% donkey serum at room temperature for 20 minutes. For ICAM-1, fibronectin and ED-1 staining, the primary polyclonal antibody to ICAM-1, the extracellular domain of fibronectin (Chemicon International, Inc., Billerica, MA, USA) or ED-1 (Chemicon International, Inc., Billerica, MA, USA), respectively, was diluted to 1 : 100 with 2% casein in BSA and was applied for overnight incubation at room temperature. After washing, a secondary donkey anti-goat antibody was added for 20 minutes and the slides were then washed and incubated with a tertiary PAP complex for 20 minutes. DAB was added for two minutes and the slides were counterstained with hematoxylin. A semi-quantitative score for measuring the intensity of ICAM-1 and fibronectin staining within the glomeruli and tubulointerstitial area was determined by examining at least 20 glomeruli under ×400 magnification and 20 tubulointerstitial fields under ×200 magnification, respectively, and by a digital image analyzer (MetaMorph version 4.6r5, Universal Imaging Corp., Downingtown, PA, USA) as previously described.17
The degree of staining was semi-quantitated on a scale of 0-4+. The staining score was obtained by multiplying the intensity of staining by the percentage of glomeruli or tubulointerstitium staining for that intensity, and these numbers were then added for each experimental animal to give the staining score [Σ=(Intensity of staining)×(% of glomeruli or tubulointerstitium with that intensity)]. The number of ED-1 positive cells was counted in at least 20 glomeruli and 20 fields of the tubulointerstitium/section under ×400 magnification.
All values are expressed as the mean±standard error of the mean. Statistical analysis was performed using the statistical package SPSS for Windows Ver. 11.0 (SPSS, Inc., Chicago, IL, USA). Results were analyzed using the Kruskal-Wallis non-parametric test for multiple comparisons. Significant differences on the Kruskal-Wallis test were further confirmed by the Mann-Whitney U test. p-values less than 0.05 were considered statistically significant.
All the animals gained weight over the three-month experimental period, but body weight was highest among the C rats (593±11 g). The ratio of kidney to body weight in the DM rats (1.17±0.15%) was significantly higher than those of the C (0.58±0.05%), C+GLA (0.53±0.06%) (p<0.01), and DM+GLA (0.88±0.11) (p<0.05) rats. The mean blood glucose levels of C, C+GLA, DM, and DM+GLA rats were 104.1±3.9, 97.6±3.5, 489.5±14.0, and 474.0±13.0 mg/dL, respectively (p<0.01). Compared to the C group (0.35±0.07 mg/day), 24-hour urinary albumin excretion at three months was significantly higher in DM rats (2.51±0.28 mg/day) (p<0.01), and GLA treatment significantly reduced albuminuria in DM rats (1.11±0.12 mg/day) (p<0.05) (Table 1).
Renal MCP-1 mRNA expression assessed by real-time PCR was significantly higher in DM than in C rats (p<0.01), and this increase in MCP-1 mRNA expression was significantly inhibited by administration of GLA (p<0.05). The MCP-1 mRNA/18s rRNA ratio was 2.1-fold higher in DM than in C kidneys, and GLA treatment significantly abrogated this increase by 65.7%. The ratios of ICAM-1 and fibronectin mRNA/18s rRNA were also significantly higher in DM compared to C and C+GLA kidneys (p<0.01), and GLA treatment significantly attenuated increases in renal ICAM-1 and fibronectin mRNA expression in DM rats (p<0.05) (Fig. 1).
The levels of renal MCP-1 assessed by ELISA were also significantly higher in DM than in C rats (563.5±42.9 vs. 287.1±22.3 ng/µg, p<0.01), and the increase in MCP-1 levels in DM rats was significantly ameliorated by GLA treatment (354.9±31.3 ng/µg, p<0.05) (Fig. 2). Renal ICAM-1 and fibronectin protein expression assessed by Western blot was also significantly increased in DM rats relative to C and C+GLA rats (p<0.01), and GLA treatment significantly abrogated these increases in DM rats (p<0.05) (Fig. 3). In addition, immunohistochemical staining for ICAM-1 and fibronectin confirmed the real-time PCR and Western blot findings. There were significant increases in glomerular and tubulointerstitial ICAM-1 and fibronectin staining in the DM group compared to the C and C+GLA groups, and these increases in DM rats were significantly ameliorated by the administration of GLA (Fig. 4).
The number of macrophages within glomeruli and tubulointerstitium assessed by immunohistochemical staining with ED-1 antibody was significantly higher in DM rats than in C rats (27.8±4.3 vs. 3.6±0.8, p<0.01), and GLA treatment significantly abrogated the number of ED-1-positive cells in DM rats (10.1±1.2) (p<0.05) (Fig. 4).
MCP-1 mRNA expression assessed by real-time PCR was significantly increased in HG-stimulated mesangial cells and NRK-52E cells (p<0.01), and this increase in MCP-1 mRNA expression was significantly abrogated by GLA treatment (p<0.05). Compared to NG cells, the MCP-1 mRNA/18s rRNA ratios were 2.0- and 2.1-fold higher in mesangial and NRK-52E cells exposed to HG medium (p<0.01), respectively, and GLA treatment significantly ameliorated these increases in a dose-dependent manner (p<0.05) (Fig. 5). The levels of MCP-1 protein in conditioned culture media assessed by ELISA showed a similar pattern to the mRNA expression (Fig. 5). However, linoleic acid did not induce significant changes in these patterns (data not shown).
High glucose significantly induced ICAM-1 and fibronectin mRNA expression in mesangial and NRK-52E cells. Compared to NG cells, the ICAM-1 mRNA/18s rRNA ratios were 2.2- and 1.8-fold higher in HG-stimulated mesangial cells and tubular epithelial cells (p<0.01), respectively, and these increases were significantly attenuated by 60.9% and 62.3%, respectively, with 10 µM GLA treatment, and by 70.7% and 76.1%, respectively, with 100 µM GLA treatment (Fig. 6). Fibronectin mRNA/18s rRNA ratios were significantly increased in HG-stimulated mesangial and NRK-52E cells relative to NG cells by 154.4% and 122.2% (p<0.01), respectively, and these increases were significantly abrogated by GLA in a dose-dependent manner (p<0.05) (Fig. 6).
ICAM-1 and fibronectin protein expression showed similar patterns to their mRNA expressions. GLA also significantly inhibited HG-induced ICAM-1 and fibronectin protein expression in cultured mesangial cells and NRK-52E cells (Fig. 7).
In the present study, we demonstrated that GLA has a renoprotective effect via its anti-inflammatory and anti-fibrotic actions in experimental diabetic nephropathy. In addition, the results of this study suggested that the anti-inflammatory effect of GLA under diabetic conditions is partly mediated by inhibiting increases in MCP-1 and ICAM-1 expression under diabetic conditions.
Even though the diabetic milieu per se, hemodynamic changes, and local growth factors such as AII and TGF-β are considered mediators in the pathogenesis of diabetic nephropathy,3,6 recent studies suggest that an inflammatory mechanism may also contribute to the development of diabetic nephropathy based on the pathological findings of inflammatory cell infiltration in diabetic kidneys.4 Monocytes/macrophages are the major inflammatory cells found in diabetic kidneys.5,17 They are extravasculated from the bloodstream and attracted to the target tissue through a process mediated by various chemokines and adhesion molecules such as MCP-1 and ICAM-1.7,18 In the kidneys, MCP-1 is expressed in mesangial cells and tubular epithelial cells and is known to be involved in the pathogenesis of various renal diseases, including diabetic nephropathy.7,19 Previous studies have demonstrated that plasma MCP-1 levels are higher in type 1 diabetes with microalbuminuria20 and that urinary levels of MCP-1 are higher in accordance with the extent of albuminuria.7 Renal expression of ICAM-1, a cell surface glycoprotein that plays a major role in the regulation of interactions with immune cells and whose expression is upregulated at sites of inflammation,8,21 is also known to be increased in experimental type 1 and type 2 diabetic animals.17,21 These findings suggest that MCP-1 and ICAM-1 may play an important role in the pathogenesis of diabetic nephropathy by inducing inflammatory cell infiltration.7,8 Once the recruited monocytes/macrophages are activated, they release lysosomal enzymes, nitric oxide, reactive oxygen species, platelet-derived growth factor (PDGF), tumor necrosis factor-α, interleukin (IL)-1, and TGF-β, in turn promoting renal injury.5,6,22 PDGF stimulates fibroblast proliferation6 and IL-1 induces the expression of TGF-β, the most well-known profibrotic cytokine, in fibroblasts.5 In experimental diabetic nephropathy, various anti-inflammatory agents inhibited not only inflammatory cell infiltration by abrogating increases in MCP-1 and ICAM-1 expression but also ameliorated ECM accumulation.17,23 In addition, renal fibrosis was significantly inhibited along with less inflammatory cell infiltration in MCP-1- and ICAM-1-deficient diabetic mice.24,25 Taken together, this suggests that the inhibition of inflammatory cell recruitment may lead to an attenuation of ECM accumulation. In this study, we demonstrated that MCP-1 and ICAM-1 expression were increased in experimental diabetic nephropathy, which were associated with glomerular and tubulointerstitial fibrosis, and in high glucose-stimulated mesangial cells and tubular epithelial cells, and these increases under diabetic conditions were inhibited by GLA treatment. In addition, GLA reduced the ratio of kidney/body weight in diabetic rats, suggesting GLA prevents diabetes-induced kidney enlargement. A recent study also demonstrated that prevention of glomerular hypertrophy ameliorates the development of diabetic nephropathy, including proteinuria and podocytopenia.26 Taken together, the anti-inflammatory, anti-fibrotic, and anti-hypertrophic effects of GLA in diabetic nephropathy may be partly attributable to the suppression of MCP-1 and ICAM-1 expression by GLA, by which inflammatory cell infiltration is abrogated, in turn ameliorating ECM accumulation.
PUFAs are important constituents of all cell membranes. Since PUFAs are not synthesized in humans, they can only be obtained by diet.11 There are two classes of PUFAs: ω-3 and ω-6, designated according to their carbon ring structure;12 and accumulating evidence has shown that these PUFAs are beneficial to one's overall health and a number of various diseases.13 In cases of kidney disease, PUFAs have been reported to have beneficial effects on IgA nephropathy,27 chronic renal failure, and diabetic nephropathy via anti-oxidant, anti-inflammation, and anti-fibrotic mechanisms.13,28 In contrast, the effect of GLA, a member of the ω-6 PUFA family, on kidney diseases has been less explored. Ingram, et al.14 observed that administration of borage oil (BO), a rich source of GLA, was effective in a rat 5/6-renal-ablation model. BO prevented increases in blood pressure and proteinuria, rising plasma cholesterol levels, and declining glomerular filtration rates. In addition, glomerular macrophage infiltration, mesangial expansion, and glomerulosclerosis were attenuated in BO-treated rats compared to the control diet group. Meanwhile, since these glomerular changes are also characteristic of diabetic nephropathy, the activities of δ-5 and δ-6 desaturase are decreased along with low levels of GLA and DGLA in diabetes,29 and since GLA has proven useful in diabetic neuropathy,16,30 supplementation of GLA and/or DGLA may also be of benefit in diabetic nephropathy. However, the effect of ω-6 PUFA has never been elucidated in diabetic nephropathy. The results of the present study showed for the first time that GLA inhibits inflammatory cell infiltration and ECM accumulation in experimental diabetic kidneys, suggesting the usefulness of GLA in patients with diabetic nephropathy.
Even though the underlying mechanisms of the anti-inflammatory effect of GLA in this study are not completely understood, several plausible explanations can be proposed. First, PUFAs, including GLA, are known to serve as endogenous ligands of peroxisome proliferator activated receptors (PPARs) and to bind and activate all PPARs isoforms.31 Additionally, 15-HETE, one of the metabolites of GLA, has been reported to upregulate nuclear PPAR-γ expression.32 PPARs participate in regulating inflammatory responses by inhibiting monocyte expression of proinflammatory cytokines such as IL-6, IL-1β, and TNF-α.33 Furthermore, PPAR-γ attenuates the nuclear factor-κB-mediated transcriptional activation of proinflammatory genes.33 Recent studies have also demonstrated that PPAR-γ agonist exerts a renoprotective effect through an anti-inflammatory mechanism in diabetic nephropathy.34 Taken together, GLA, as a ligand of PPARs and its metabolite, as well as an upregulator of PPAR-γ, could induce an anti-inflammatory effect in diabetic nephropathy. Second, a small amount of DGLA can be converted to prostacyclin (PGI2) and prostaglandin E1 (PGE1) by δ-5 desaturases. Because PGI2 and PGE1 inhibit platelet aggregation, and PGI2 analogue abrogates glomerular hyperfiltration and macrophage infiltration in diabetic kidneys,35 the effect of GLA may be in part attributed to these consequences. Third, 15-HETE markedly inhibits the generation of leukotriene, which is a potent pro-inflammatory mediator, via stimulating adhesion molecule expression and macrophage infiltration.36 Collectively, the anti-inflammatory effect of GLA seems to be attributable to the modulation of biological cascades at multiple sites by itself and/or its metabolites. Prolonged use of anti-inflammatory drugs may be harmful and is not appropriate for long-term use due to many systemic side effects in patients with chronic metabolic disturbances such as diabetic nephropathy. However, since the activities of both δ-5 and δ-6 desaturase are already reduced in diabetes and the toxic effects of GLA as a medicinal oil have not been reported, chronic administration of GLA, even at a high dosage, may not induce accumulation of its metabolites and thus would not be harmful.
In summary, the results of the present study demonstrate that GLA exerts anti-inflammatory and anti-fibrotic effects in experimental diabetic nephropathy and in high glucose-stimulated renal cells, suggesting that GLA supplementation could be a valuable therapeutic option for the treatment of diabetic nephropathy.
Figures and Tables
Fig. 1
Renal MCP-1, ICAM-1, and fibronectin mRNA/18s rRNA ratios in control (C), C+gamma linolenic acid (GLA), diabetic (DM), and DM+GLA rats. There was a 2.1-fold increase in MCP-1 mRNA/18s rRNA, a 1.8-fold increase in ICAM-1 mRNA/18s rRNA, and a 2.7-fold increase in fibronectin mRNA/18s rRNA ratios in DM rats compared to C rats, and GLA treatment significantly abrogated these increases in mRNA/18s rRNA ratios in DM rats. *p<0.01 vs. C and C+GLA groups. †p<0.05 vs. DM group. MCP-1, monocyte chemoattractant protein-1; ICAM-1, intracellular adhesion molecule-1.
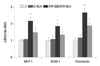
Fig. 2
Renal MCP-1 protein levels in C, C+GLA, DM, and DM+GLA rats. There was a 2.0-fold increase in renal MCP-1 protein levels in DM rats compared to C rats, and this increase in the DM rats was significantly ameliorated by GLA treatment. *p<0.01 vs. C and C+GLA groups. †p<0.05 vs. DM group. C, control; GLA, γ-linolenic acid; DM, diabetes; MCP-1, monocyte chemoattractant protein-1.
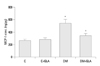
Fig. 3
Renal ICAM-1 and fibronectin protein expression in C, C+GLA, DM, and DM+GLA rats. There was a 3.2-fold increase in ICAM-1 and a 3.7-fold increase in fibronectin protein expression in DM rats compared to C rats, and these increases were significantly attenuated by administration of GLA. *p<0.01 vs. C and C+GLA groups. †p<0.05 vs. DM group. ICAM-1, intracellular adhesion molecule-1; C, control; GLA, γ-linolenic acid; DM, diabetes.
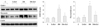
Fig. 4
Immunohistochemical staining for glomerular and tubulointerstitial ICAM-1, fibronectin, and ED-1 (as a marker of macrophage) in C, C+GLA, DM, and DM+GLA rats. Glomerular (A) and tubulointerstitial (B) ICAM-1 and fibronectin staining was significantly increased in DM rats compared to C rats, and GLA treatment significantly inhibited these increases in DM rats. The number of ED-1-positive cells was significantly higher in DM rats than in C rats, and GLA treatment significantly abrogated the number of glomerular and tubulointerstitial macrophages in DM rats. (C) IHC scores for ICAM-1 and fibronectin within the glomeruli and the tubulointerstitial area were significantly higher in DM rats relative to C rats, and GLA treatment significantly attenuated these increases in DM rats (×400). *p<0.05 vs. other groups. †p<0.01 vs. C and C+GLA groups. ‡p<0.05 vs. DM group. ICAM-1, intracellular adhesion molecule-1; C, control; GLA, γ-linolenic acid; DM, diabetes.
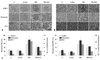
Fig. 5
MCP-1 mRNA/18s rRNA ratios and secreted MCP-1 protein levels in mesangial cells (A) and NRK-52E cells (B) exposed to 5.6 mM glucose (NG), NG+24.4 mM mannitol (NG+M), NG+10 or 100 µM GLA (NG+GLA), 30 mM glucose (HG), and HG+10 or 100 µM GLA (HG+GLA). There were 2.0- and 2.1-fold increases in MCP-1 mRNA/18s rRNA ratios in the HG-stimulated mesangial and NRK-52E cells, respectively, compared to the NG cells, and these increases in MCP-1 mRNA expression were significantly ameliorated by GLA treatment in a dose-dependent manner. There were 2.4- and 3.6-fold increases in MCP-1 levels in HG-stimulated mesangial cells and NRK-52E cells, respectively, compared to NG cells, and GLA significantly attenuated these increases in MCP-1 levels in a dose-dependent manner. *p<0.01 vs. NG, NG+M, and NG+GLA groups. †p<0.05 vs. HG group. MCP-1, monocyte chemoattractant protein-1; GLA, γ-linolenic acid.
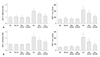
Fig. 6
ICAM-1 and fibronectin mRNA/18s rRNA ratios in mesangial cells (A) and NRK-52E cells (B) exposed to 5.6 mM glucose (NG), NG+24.4 mM mannitol (NG+M), NG+10 or 100 µM GLA (NG+GLA), 30 mM glucose (HG), and H+10 or 100 µM GLA (HG+GLA). There were 2.2- and 1.8-fold increases in ICAM-1 mRNA/18s rRNA ratios in HG-stimulated mesangial cells and NRK-52E cells, respectively, compared to NG cells, and these increases in ICAM-1 mRNA/18s rRNA ratios were significantly abrogated by administration of GLA. There were 2.5-fold and 2.2-fold increases in fibronectin mRNA/18s rRNA ratios in HG-stimulated mesangial cells and NRK-52E cells, respectively, compared to NG cells, and GLA treatment significantly attenuated these increases in fibronectin mRNA/18s rRNA ratios in a dose-dependent manner. *p<0.01 vs. NG, NG+M, and NG+GLA groups. †p<0.05 vs. HG group. ICAM-1, intracellular adhesion molecule-1, GLA, γ-linolenic acid.
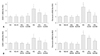
Fig. 7
Representative Western blots of ICAM-1 and fibronectin in cultured mesangial cells (A) and NRK-52E cells (B). There were significant increases in ICAM-1 and fibronectin protein expression in HG-stimulated cells as compared to NG cells, and these increases were significantly ameliorated with GLA treatment. *p<0.01 vs. NG, NG+M, and NG+GLA groups. †p<0.05 vs. HG group. ICAM-1, intracellular adhesion molecule-1, GLA, γ-linolenic acid.
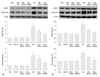
ACKNOWLEDGEMENTS
This study was supported by a faculty research grant of Yonsei University College of Medicine for 2008 (6-2008-0105).
References
1. Wolf G, Ziyadeh FN. Molecular mechanisms of diabetic renal hypertrophy. Kidney Int. 1999. 56:393–405.


2. Chen S, Jim B, Ziyadeh FN. Diabetic nephropathy and transforming growth factor-beta: transforming our view of glomerulosclerosis and fibrosis build-up. Semin Nephrol. 2003. 23:532–543.


3. Solini A, Iacobini C, Ricci C, Chiozzi P, Amadio L, Pricci F, et al. Purinergic modulation of mesangial extracellular matrix production: role in diabetic and other glomerular diseases. Kidney Int. 2005. 67:875–885.


4. Navarro-González JF, Mora-Fernández C. The role of inflammatory cytokines in diabetic nephropathy. J Am Soc Nephrol. 2008. 19:433–442.


5. Furuta T, Saito T, Ootaka T, Soma J, Obara K, Abe K, et al. The role of macrophages in diabetic glomerulosclerosis. Am J Kidney Dis. 1993. 21:480–485.


6. Young BA, Johnson RJ, Alpers CE, Eng E, Gordon K, Floege J, et al. Cellular events in the evolution of experimental diabetic nephropathy. Kidney Int. 1995. 47:935–944.


7. Banba N, Nakamura T, Matsumura M, Kuroda H, Hattori Y, Kasai K. Possible relationship of monocyte chemoattractant protein-1 with diabetic nephropathy. Kidney Int. 2000. 58:684–690.


8. Sugimoto H, Shikata K, Hirata K, Akiyama K, Matsuda M, Kushiro M, et al. Increased expression of intercellular adhesion molecule-1 (ICAM-1) in diabetic rat glomeruli: glomerular hyperfiltration is a potential mechanism of ICAM-1 upregulation. Diabetes. 1997. 46:2075–2081.


9. Utimura R, Fujihara CK, Mattar AL, Malheiros DM, Noronha IL, Zatz R. Mycophenolate mofetil prevents the development of glomerular injury in experimental diabetes. Kidney Int. 2003. 63:209–216.


10. Nasrallah R, Robertson SJ, Hébert RL. Chronic COX inhibition reduces diabetes-induced hyperfiltration, proteinuria, and renal pathological markers in 36-week B6-Ins2 (Akita) mice. Am J Nephrol. 2009. 30:346–353.


11. Benatti P, Peluso G, Nicolai R, Calvani M. Polyunsaturated fatty acids: biochemical, nutritional and epigenetic properties. J Am Coll Nutr. 2004. 23:281–302.


12. Buist PH. Fatty acid desaturases: selecting the dehydrogenation channel. Nat Prod Rep. 2004. 21:249–262.


13. Fan YY, Chapkin RS. Importance of dietary gamma-linolenic acid in human health and nutrition. J Nutr. 1998. 128:1411–1414.
14. Ingram AJ, Parbtani A, Clark WF, Spanner E, Huff MW, Philbrick DJ, et al. The Nutrition & Kidney Disease Research Group. Dietary alteration of dihomogamma-linolenic acid/arachidonic acid ratio in a rat 5/6-renal-ablation model. J Am Soc Nephrol. 1996. 7:1024–1031.


15. Zurier RB, Rossetti RG, Jacobson EW, DeMarco DM, Liu NY, Temming JE, et al. gamma-Linolenic acid treatment of rheumatoid arthritis. A randomized, placebo-controlled trial. Arthritis Rheum. 1996. 39:1808–1817.


16. Keen H, Payan J, Allawi J, Walker J, Jamal GA, Weir AI, et al. The gamma-Linolenic Acid Multicenter Trial Group. Treatment of diabetic neuropathy with gamma-linolenic acid. Diabetes Care. 1993. 16:8–15.
17. Li JJ, Lee SH, Kim DK, Jin R, Jung DS, Kwak SJ, et al. Colchicine attenuates inflammatory cell infiltration and extracellular matrix accumulation in diabetic nephropathy. Am J Physiol Renal Physiol. 2009. 297:F200–F209.


18. Huang GT, Eckmann L, Savidge TC, Kagnoff MF. Infection of human intestinal epithelial cells with invasive bacteria upregulates apical intercellular adhesion molecule-1 (ICAM)-1) expression and neutrophil adhesion. J Clin Invest. 1996. 98:572–583.


19. Rovin BH, Yoshiumura T, Tan L. Cytokine-induced production of monocyte chemoattractant protein-1 by cultured human mesangial cells. J Immunol. 1992. 148:2148–2153.
20. Chiarelli F, Cipollone F, Mohn A, Marini M, Iezzi A, Fazia M, et al. Circulating monocyte chemoattractant protein-1 and early development of nephropathy in type 1 diabetes. Diabetes Care. 2002. 25:1829–1834.


21. Coimbra TM, Janssen U, Gröne HJ, Ostendorf T, Kunter U, Schmidt H, et al. Early events leading to renal injury in obese Zucker (fatty) rats with type II diabetes. Kidney Int. 2000. 57:167–182.


22. Yeo ES, Hwang JY, Park JE, Choi YJ, Huh KB, Kim WY. Tumor necrosis factor (TNF-alpha) and C-reactive protein (CRP) are positively associated with the risk of chronic kidney disease in patients with type 2 diabetes. Yonsei Med J. 2010. 51:519–525.


23. Kikuchi Y, Imakiire T, Yamada M, Saigusa T, Hyodo T, Hyodo N, et al. Mizoribine reduces renal injury and macrophage infiltration in non-insulin-dependent diabetic rats. Nephrol Dial Transplant. 2005. 20:1573–1581.


24. Chow FY, Nikolic-Paterson DJ, Ozols E, Atkins RC, Tesch GH. Intercellular adhesion molecule-1 deficiency is protective against nephropathy in type 2 diabetic db/db mice. J Am Soc Nephrol. 2005. 16:1711–1722.


25. Chow FY, Nikolic-Paterson DJ, Ma FY, Ozols E, Rollins BJ, Tesch GH. Monocyte chemoattractant protein-1-induced tissue inflammation is critical for the development of renal injury but not type 2 diabetes in obese db/db mice. Diabetologia. 2007. 50:471–480.


26. Kim DK, Nam BY, Li JJ, Park JT, Lee SH, Kim DH, et al. Translationally controlled tumour protein is associated with podocyte hypertrophy in a mouse model of type 1 diabetes. Diabetologia. 2012. 55:1205–1217.


27. Nolin L, Courteau M. Management of IgA nephropathy: evidence-based recommendations. Kidney Int Suppl. 1999. 70:S56–S62.


28. Fassett RG, Gobe GC, Peake JM, Coombes JS. Omega-3 polyunsaturated fatty acids in the treatment of kidney disease. Am J Kidney Dis. 2010. 56:728–742.


29. Das UN. Essential fatty acid metabolism in patients with essential hypertension, diabetes mellitus and coronary heart disease. Prostaglandins Leukot Essent Fatty Acids. 1995. 52:387–391.


30. Hounsom L, Horrobin DF, Tritschler H, Corder R, Tomlinson DR. A lipoic acid-gamma linolenic acid conjugate is effective against multiple indices of experimental diabetic neuropathy. Diabetologia. 1998. 41:839–843.


31. Forman BM, Chen J, Evans RM. Hypolipidemic drugs, polyunsaturated fatty acids, and eicosanoids are ligands for peroxisome proliferator-activated receptors alpha and delta. Proc Natl Acad Sci U S A. 1997. 94:4312–4317.


32. Pham H, Banerjee T, Nalbandian GM, Ziboh VA. Activation of peroxisome proliferator-activated receptor (PPAR)-gamma by 15S-hydroxyeicosatrienoic acid parallels growth suppression of androgen-dependent prostatic adenocarcinoma cells. Cancer Lett. 2003. 189:17–25.


33. Daynes RA, Jones DC. Emerging roles of PPARs in inflammation and immunity. Nat Rev Immunol. 2002. 2:748–759.


34. Zhang Y, Guan Y. PPAR-gamma agonists and diabetic nephropathy. Curr Diab Rep. 2005. 5:470–475.