Abstract
Purpose
The aim of this study was to identify the adipocyte-specific gene expression patterns in chorion-derived mesenchymal stem cells during adipogenic differentiation.
Materials and Methods
Chorionic cells were isolated from the third trimester chorions from human placenta at birth and identified morphologically and by fluorescence-activated cell sorting analysis. After inducing adipogenic differentiation for 28 days, cells at days 3, 10, 21 and 28 were analyzed by Oil red O staining and RNA extraction in order to assess the expression levels of adipocyte marker genes, including CCAAT-enhancer binding protein α (C/EBPα), peroxisome proliferator-activated receptor γ (PPARγ), fatty acid binding protein 4 (FABP4) and Glycerol-3-phosphate dehydrogenase (GPD2). Cells not induced for differentiation were compared with the induced cells as a control group.
Results
Chorion-derived cells showed the same pattern as fibroblasts, and expressed CD73, CD105, and CD166 antigens, but not CD45, CD34, and HLA-DR antigens. On day 3 after differentiation, cells began to stain positively upon Oil red O staining, and continuously increased in lipid granules for 4 weeks. The expression level of C/EBPα increased 4.6 fold on day 3 after induction, and continued to increase for 4 weeks. PPARγ was expressed at a maximum of 2.9 fold on day 21. FABP4 and GPD2 were significantly expressed at 4.7- and 3.0-fold, respectively, on day 21, compared to controls, and further increased thereafter.
Mesenchymal stem cells (MSCs), isolated for the first time from human bone marrow, can be induced to differentiate into various connective tissue cells, such as osteocytes, chondrocytes, adipocytes, atrial myocytes, myoblasts and neurons.1-3 Their ability to differentiate into various connective tissue cells is useful for treating diseases caused by tissue destruction or abnormal cell function. For this purpose, many studies have set out to determine the characteristics of cell differentiation by extracting and culturing mesenchymal stem cells from various tissues.4
Recently, ongoing studies have attempted to reveal the characteristics of stem cells by examining time series of gene expressions as well as changes in the cell phenotype of mesenchymal stem cells throughout differentiation into specific cells.5 Hepatocytes (AT-MSC-Hepa), differentiated from human fat-derived mesenchymal stem cells, and liver showed a striking similarity in gene expression related to liver-specific functions, such as blood clotting cascade complementary activation.6 Using analysis of periodic expression of estrogen receptor alpha (ER-α) messenger RNA (mRNA) of rat bone marrow-derived stem cells, the alteration of ER-α mRNA proved to be correlated with the growth and osteogenic differentiation of bone marrow-derived stem cells.7
Adult bone marrow mesenchymal stem cells have been the most common studied, but the sampling method thereof is invasive and the retrieval rate of cells from human adult bone marrow decreases with age. Embryonic stem cells and fetal tissues are rich in mesenchymal stem cells; however, ethical limitations as well as both the stability and efficiency of these cells limit their use.8-10 Recently, mesenchymal stem cells have been isolated and studied using by-products from pregnancies, such as cord blood, umbilical Wharton's jelly, amniotic fluid, fetal membranes and placenta.11-14
The fetal membrane is composed of the amnion and chorion; mesenchymal stem cells isolated from each of them exhibit high proliferative capacity and various levels of differentiation into ectoderm, mesoderm and endoderm-type cells.12,15 As mesenchymal stem cells isolated from pregnancy by-products provide richer sources than other tissues with no ethical limitations, as well as show high proliferative capacity and differentiation, they are expected to allow for greater clinical applications easier than stem cells derived from other tissues.
In the process of differentiation to adipocytes, progenitor cells show the periodic biochemical changes, which involve expression of genes regulating transcription in the early periods and sequential expression of genes associated with the adipogenesis and insulin sensitivity of mature adipocytes, as the differentiation proceeds, along with structural changes of generation and accumulation of lipid granules in the cytoplasm.16 However, Hutley, et al.17 found that the amount of lipid granules generated in the cell during adipose differentiation from pre-adipocyte was not commensurate with the specific gene expression level of mature adipocytes. This means that generation of lipid granules cannot solely prove the mature adipogenic differentiation. Previous studies of chorion-derived mesenchymal stem cells determined the differentiation into adipocyte by formation of lipid granules and increased single gene expression;15,18 however, to date, no study has continuously observed the expression pattern of adipocyte marker genes after induction of differentiation.
In this study, we isolated mesenchymal stem cells from the chorion of the third trimester pregnancy, and studied changes in cell morphology over time as well as the expression of adipocyte marker genes during adipogenic differentiation.
The chorions used in this study were isolated from placentas obtained during uncomplicated elective Cesarean sections with informed consent from each donor patient (n=3). We washed and removed blood from the chorions using Hank's balanced salt solution (HBSS; GIBCO, Grand Island, NY, USA). Each chorion was then trypsinized in 0.5% Trypsin EDTA (GIBCO) at 37℃ for 5 minutes, and impurities were removed. After two cycles of trypsinization at 37℃ for 30 minutes, the cells were filtered through 100 µm cell strainers (BD Bioscience, San Jose, CA, USA), and washed 3 times with HBSS. After plating on 100-mm-diameter culture dishes, the cells were cultured in the incubator (37℃, 5% CO2) for 3-5 days in Dulbecco's modified eagle medium (DMEM; GIBCO) including 15% fetal bovine serum (FBS; GIBCO) and 1% antibiotic-antimycotic (GIBCO). Culture was maintained for 3-4 weeks, replacing the medium twice a week until 70-80% confluency. When colonies of 50 or more fibroblast like cells appeared in the culture, cell clusters were subcultured at 1×104 cells per cm2 into a different culture dish using trypsin. In total, cell clusters were subcultured for 8 times.
These experiments were conducted after all three chorion donors were provided with all relevant information and approval was obtained from the Institutional Review Board of The Catholic University of Korea (approval No. KC09WZZZ0173).
After removing the cultured cells from the medium and washing twice with PBS, the adherent cells were detached with 0.25% trypsin-EDTA (GIBCO) and centrifuged at 1500 rpm for 5 minutes. After removing the supernatant, a sufficient amount of PBS was added, and cells were subcultured in 1.5 mL Eppendorf tubes. The mesenchymal stem cell phenotype was determined using the following antibodies: CD73-phycoerythrin (PE; BD Bioscience, San Jose, CA, USA), CD105-PE (Beckman coulter, Marseille, France), CD166-PE (BD Bioscience), CD34-PE (BD Bioscience), CD45-PE (BD Bioscience) and HLA-DR-fluorescein isothiocyanate (BD Bioscience). Cells were incubated for 30 minutes at room temperature. After centrifuging for 5 minutes at 2100 rpm, the supernatant was removed, and 500 µL of PBS were added. Then, 1 µL of 7-amino-actinomycin D (BD Bioscience) was added, transferred to a 5 mL Fluorescence activated cell sorter (FACS) tube, and analyzed using flow cytometer (FACSCalibur; Becton Dickinson, Franklin Lakes, NJ, USA).
Cells from passage 7 were used for adipose differentiation. After colonizing in 6-well plates (Corning, Lowell, MA, USA) with sterile cover slides, cells were cultured for 7 days in a growth culture medium, comprising DMEM/GlutaMAX™-I (GIBCO), 10% FBS (GIBCO) and 1% penicillin-streptomycin. Then, the differentiation process was observed for 4 weeks in a 100 mm culture dish with the low glucose adipocyte induction medium DMEM-L (GIBCO), supplemented with 10 µg/mL each of transforming growth factor-β, 100 nM dexamethasone, 50 µg/mL of ascorbic acid, 100 µg/mL of sodium pyruvate, and 40 µg/mL of proline and insulin-transferring-sodium selenite, while exchanging the culture medium every 3 days. In the control group, cells not induced for differentiation were cultured in DMEM-Ham's F-12 medium (GIBCO), supplemented with 10% FBS. In both the study and control groups, cells were retrieved from the culture dishes for Oil red O staining on days 3, 10, 21 and 28 before differentiation or were stored in liquid nitrogen using cryotubes for total RNA extraction.
After the culture medium was removed and washed twice with PBS, the differentiated cells were fixed with absolute (100%) propylene glycol for 2 minutes at room temperature. After washing twice with PBS, cells was treated with 0.5% Oil red O (Sigma-Aldrich AG, Chemie Gmbh Munich, Germany) in isopropanol for 1 hour at 37℃ in the incubator. Thereafter, Oil red O was removed, and cells were subsequently treated with 85% propylene glycol at room temperature for 1 minute. After washing twice with PBS, cells were considered positive when bright red dye was observed on the microscope.
On days 3, 10, 21, and 28 before differentiation, total RNA was isolated from cells in both groups, using the RiboPure™ kit (Applied Biosystems/Ambion, Austin, TX, USA). First, after washing the cryotube containing the cells with PBS, 1 mL TRI® reagent (Sigma-Aldrich, Chemie Gmbh Munich, Germany) was added and left at room temperature for 5 minutes. Then, the cryotubes were centrifuged at 12000 g for 10 min at 4℃. After the supernatant was transferred into a new centrifuge tube, 200 µL of chloroform solution was added, left at room temperature for 5 minutes, and centrifuged at 12000 g for 10 min at 4℃. After the supernatant was again transferred to a new centrifuge tube, 200 µL of 100% ethanol was added, transferred to a filter cartridge, and centrifuged for 1 minute at 10000 g. After adding 500 µL of washing solution, the filter cartridge was centrifuged for 1 minute at 10000 g. Then 50 µL of elution buffer was added to the filter cartridge and the total RNA was obtained after 1 minute of centrifugation at 10000 g. Total RNA was determined by measuring the OD value on a spectrophotometer Nanodrop (Thermo scientific, Wilmington, DE, USA). The total RNA was kept at minus 70℃ in the freezer.
To perform cDNA synthesis, 3 µg of total RNA, 1 µL of random primer (Bionics, Seoul, Korea) and 3.2 µL of 2.5 mM deoxyribonucleotide triphosphate (Rexgene biotech, Cheongwon, Korea) were mixed into a polymerase chain reaction (PCR) tube, reacted at 70℃ for 5 minutes, and chilled on ice. Then, 4 µL of reverse transcriptase (RT) buffer (Rexgene biotech), 0.5 µL of molony murine leukemia virus RT (Rexgene biotech) and 0.5 µL of RNase inhibitor (Invitrogen, Carlsbad, CA, USA) were mixed in, adding RNase-free water to a total volume of 20 µL. After the solution was well mixed, cDNA was synthesized after reacting at 42℃ for 1.5 hours and at 70℃ for 15 minutes.
Probes for CCAAT-enhancer binding protein α (C/EBPα), inperoxisome proliferator-activated receptor γ (PPARγ), fatty acid binding protein 4 (FABP4) and Glycerol-3-phosphate dehydrogenase (GPD2) gene were purchased from Applied Biosystems (Foster City, CA, USA). RT-PCR was monitored using the 7900 HT Fast Real-Time PCR System (Applied Biosystems) with a 10 µL solution comprising 2 µL of cDNA, 5 µL of TaqMan 2X Universal PCR Master Mix, 0.5 µL of primer and 2.5 µL of DW. For PCR progression, pre-denaturation was performed at 50℃ for 2 minutes and at 95℃ for 10 min; this was followed by denaturation at 95℃ for 15 seconds; annealing and elongation were both performed at 60℃ for 1 minute, and this process was repeated 40 times. For all samples, experiments were repeated three times under the same conditions. We used the primer specific to C/EBPα, PPARγ, FABP4, GPD2 and β-actin in order to analyze differentiation patterns for the induced cells and controls. The primers used in this experiment were based on the following cDNA: CEBPA (GenBank Accession No. NM_004364.2), PPARG (GenBank Accession No. NM_015869.4), GPD2 (GenBank Accession No. NM_000408.3), and FABP4 (GenBank Accession No. NM_001442.2).
The primer sequences were as follows: CEBPA, Forward 5'-GCA AAC TCA CCG CTC CAA TG-3', Reverse 5'-TTA GGT TCC AAG CCC CAA GTC-3'; PPAR-γ, Forward 5'-GAT ACA CTG TCT GCA AAC ATA TCA CAA-3', Reverse 5'-CCA CGG AGC TGA TCC CAA-3'; GPD2, Forward 5'-ATC GAT TAG TGG GGA GAG TTT CGT GCA-3', Reverse 5'-ATC GAT GCG TTC GCT TAA GGA ATG T-3'; FABP4, Forward 5'-GCG TGG AAT TCG ATG AAA TCA-3', Reverse 5'-CCC GCC ATC TAG GGT TAT GA-3'; and β-actin, Forward 5'-GCA CTC TTC CAG CCT TCC TTC C-3', Reverse 5'-TCA CCT TCA CCG TTC CAG TTT TT-3'.
For cells obtained at various times from both groups, RT-qPCR for adipocyte marker genes was performed three times, and gene expression was analyzed with the Sequence Detector Software version 2.1 (Applied Biosystems) and the Delta Ct method.19 The Delta Ct method expressed the RT-qPCR results for two variables as fold changes by the relative quantification method. In this study, fold changes in gene expression at each time point after differentiation, normalized to the control group and relative to the expression at time zero, was calculated using the Delta Ct method. These data were presented as mean±standard deviation, and the significance between subsequent time points was analyzed by paired t-test using the SPSS software (version 12.0; SPSS, Inc., Chicago, IL, USA). p-values <0.05 were considered statistically significant.
From cells isolated from three chorion samples, eight subcultures were successfully performed. The three passages exhibited the spindle-shaped morphology similar with fibroblasts (Fig. 1). In flow cytometry, these cells were negative for hematopoietic cell markers, such as CD45, CD34, and HLA-DR antigens, but positive for mesenchymal cell markers, such as CD73, CD105, and CD166 antigens (Fig. 2). Based upon their morphology and surface marker profile, the cultured cells derived from the chorions were confirmed as mesenchymal stem cells.
Adipogenic differentiation was induced for four weeks in the cell lines (n=3) after the seventh subculture. The phenotypic changes in Oil Red staining appeared on day 3 after in duction. As differentiation progressed, small lipid granules, observed in a scattered pattern in the initial period, grew further and combined to larger granules, transforming to ovoid or round shapes (Fig. 3). Generation of lipid granules was not observed in the control group, even after 4 weeks.
The mRNA levels of C/EBPα, PPARγ, GPD2, and FABP4 were assessed. The relative expression of the transcripts at various time points was further normalized to day 0 control values to represent a relative fold change of expression. On day 3, C/EBPα expression increased 4.6 (±0.68)-fold and continuously increased to 9.6 (±0.88)-fold on day 21 and 16.2 (±1.56)-fold on day 28. PPARγ began to be expressed on day 3 and increased to 2.9 (±0.55)-fold on day 21. GPD2 increased 1.53 (±0.95)-fold on day 3 and 3.0 (±0.95)-fold on day 21, reaching its peak expression at 3.5 (±0.57)-fold on day 28. FABP4 expression remained relatively low until day 10, increasing to 4.7 (±0.59)-fold on day 21 and to 5.1 (±0.40)-fold on day 28. Comparing the changes in transcripts between serial time points, the expressions of C/EBPα and PPARγ were found significantly elevated around days 0-3 and days 10-21. GPD2 exhibited continuous, significant change from the initial period to day 28; moreover, FABP4 was the last to exhibit a significant increase in expression, observed on day 21 (Fig. 4).
Our study is significant in that it is the first study to sequentially observe morphological changes and expression patterns of adipocyte maker genes in human chorion-derived cells during the process of adipogenic differentiation. To observe the molecular changes that occur during the process of adipogenic differentiation, this study investigated the expression patterns of C/EBPα for the early genes, PPARγ for the regulators of adipocyte marker genes, and FABP4 and GDP2 for the late genes associated with the lipid metabolism of mature adipocyte marker genes.
C/EBPα, a member of the CCAAT/enhancer binding protein family, is an early gene involved in initiating the adipogenic differentiation process. In addition, promoters of various adipocyte-related genes contain a regulatory C/EBP consensus sequence to control the activity and expression of genes.16 Hormone-mediated activation of C/EBP expressing adipoblasts were shown to cause an abrupt cessation of cell growth and accentuate terminal differentiation by increasing FABP4 expression.20
PPARγ controls various genes involved in fatty acid generation, combination and movement within a cell.21 Through extensive experiments concerning the specific roles of C/EBPα and PPARγ, PPARγ was discovered to be mainly involved in fat formation, while C/EBPα increased PPARγ expression and controlled the insulin sensitivity of the adipocyte.22-24 In the adipogenic differentiation of the C/EBPα-/- fibroblasts, less accumulation of lipids and no expression of endogenous PPARγ was observed; after inducing PPARγ expression by retroviral vector, lipid accumulation and the expression of adipocyte marker genes increased, but insulin sensitivity was not recovered.24 In contrast, lipid granules were generated in PPARγ-/- fibroblasts by addition of synthetic PPARγ ligand, but C/EBPα expression via the retrovirus did not seem to create fat.23
FABP4 and GDP are genes expressed in the late adipogenic differentiation process.25 FABP4 is involved in the accumulation of body fat, and FABP4 concentrations are known to be proportional to obesity severity and serum concentrations of triglycerides in type II diabetes. When treated with thiazolidinedione, a PPARγ ligand, FABP4 gene expression was also increased together with PPARγ. GDP2 is an enzyme located in the mitochondrial membrane that forms the glycerol phosphate shuttle and engages in the glycerol-phospholipid metabolism of mature adipocytes, to catalyze the transition of glycerol-3-phosphate into dihydroxyacetone phosphate.25,26
In the present study, increased C/EBPα expression was observed in the early periods of differentiation. PPARγ, known as a regulator of transcription for several genes, showed continuous increased expression from the initial stages of differentiation to day 21. The expression of GPD2, which is involved in fat formation, gradually increased in the early periods and was prominently elevated at day 21. FABP4 showed no change in expression over the first two weeks, but started to increase in expression level at day 21 and continued to increase thereafter. According to these results, the gene expression pattern for chorion-derived mesenchymal stem cells during the differentiation process was consistent with the known sequence for the expression of adipocyte marker genes.
In the literature, the duration of adipogenic induction according to the source of mesenchymal stem cell is a matter of debate. After 5 weeks of adipogenic induction in human umbilical cord stroma-derived mesenchymal stem cells, the expression levels of specific marker genes were found to reach a plateau after day 14 or 21, and the phenotypic response to adipogenesis significantly increased until day 35 with production of adipose specific hormones for 3-5 weeks.28 Similarly, in this study, it was assumed that phenotypic changes followed essential transcriptional changes, since the expressions of genes peaked at week 2-3 and the lipid contents upon cell staining exhibited bigger and rounder patterns after three weeks. In previous studies of chorion derived stem cells, adipogenic differentiation was usually induced for 2-3 weeks,15,18 but we continued the induction process until 4 weeks. As a result, the amount of lipid granules gradually increased to day 28, and the expression of FABP4, the late gene, was also observed to increase continuously to day 28. Therefore, we discerned that additional studies are needed to investigate the cell morphology and biochemical changes of human chorion-derived mesenchymal stem cells, which are differentiated over 4 weeks.
The most important feature of stem cells is the differentiation potential into a variety of cells. Depending on the tissue source of the cells, mesenchymal stem cells have demonstrated different levels of differentiation when they are induced into specific cells. In other studies, chorion-derived mesenchymal stem cells from 3rd trimester pregnancies were better induced into chondrocytes, osteocytes, myocytes, and neurons than amnion-derived stem cells.15 In a comparative study of mesenchymal stem cells derived from bone marrow and cord blood, in regards to osteogenic differentiation, the cells from cord blood showed stronger osteogenic potential but a lower capacity for adipogenic differentiation than those from bone marrow.27 In the adipogenic differentiation of umbilical cord stroma-derived mesenchymal stem cells, PPARγ and C/EBPβ were expressed more than 27 fold and 13 fold, respectively, in the first week after induction, and the genes of specific enzymes of mature adipocytes showed an approximately 7 to 140 fold increase in their expression levels.28 In bone marrow derived mesenchymal stem cells, PPARγ and FABP4 were expressed more than 14 and 1000 times, respectively, in the first week after induction,29 and the fat derived stem cells exhibited a higher capacity for adipogenic differentiation than amniotic fluid derived stem cells.30 Mesenchymal cells from human chorions showed poor potency for adipogenic differentiation and only 5-10% of those were able to form mature adipocytes.15 In this study, the expression of adipocyte marker genes was observed to be less than and slower than stem cells derived from other tissues. Therefore, adipogenic potential of chorion-derived stem cells is considered lower than that of stem cells derived from other tissues.
Although the rate of differentiation of chorion-derived mesenchymal stem cells into adipocytes was not assessed in this research, it was observed to be different according to the medium used for inducing adipogenic differentiation, as reported previously. Future specific studies on how differentiation media and additives to the culture medium may affect differentiation are needed.
Terminal differentiation to mature adipocytes is characterized by the acquirement of endocrine function by production of adipocyte-specific hormones such as leptin and adiponectin, which promote insulin sensitivity and lipid accumulation. Periodic changes in both cell morphology and gene expression were observed after adipogenic induction of human chorion derived cells; however, studies concerning insulin sensitivity in differentiated cells were not conducted. Therefore, we could not determine whether the chorion-derived stem cells differentiated into mature adipocytes with complete and unique characteristics. Future studies on hormone secretion and specific gene expression associated with insulin sensitivity may increase the understanding of adipogenic differentiation in chorion-derived mesenchymal stem cells.
In this study, mesenchymal stem cells derived from the chorions of the 3rd trimester pregnancies were established, and both lipid granule formation and adipocyte marker gene expression were sequentially observed. From our results, a culture period of four weeks or more would be more appropriate for inducing adipogenic differentiation in chorion-derived mesenchymal stem cells, although their differentiation level would remain lower than that of mesenchymal stem cells derived from other tissues. These results may constitute the basis for understanding the characteristics of chorion-derived mesenchymal stem cells and may prove helpful for planning future studies.
Figures and Tables
Fig. 1
Morphology of cultured cells isolated from chorions observed by optical microscopy at 100-fold magnitude. Cells isolated from the chorions showed the typical morphology of fibroblast-like cells, which was maintained for eight passages of culture (A: passage 3, B: passage 4, C: passage 6).
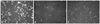
Fig. 2
Immunophenotypic characterization of the chorion derived cells. Fluorescent-activated cell sorting analysis demonstrated that cells were positive for the markers of bone marrow derived mesenchymal stem cell, such as CD73, CD166, and CD105, but negative for hematopoietic markers (CD34 and CD45) and HLA-DR.
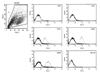
Fig. 3
Oil red stain (A-F, ×100) of the chorion derived mesenchymal stem cells during adipogenic differentiation for 4 weeks. A significant increase in lipid granule size was noticed in induced cells, compared with controls, from day 3 until day 28 of induction, as indicated by the appearance of oil red O-positive lipid granules (A and B: Control at day 0 and day 28. C at day 3, D at day 10, E at day 21, F at day 28 from adipogenic induction).
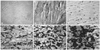
Fig. 4
The mRNA levels of C/EBPα (A), PPARγ (B), GPD2 (C) and FABP4 (D) were assessed in induced (▪) and non-induced (•) samples using real time quantitative PCR. The relative expression of the transcripts at various time points was further normalized to day 0 control values, to represent the relative fold change in expression. Comparing changes in transcripts between serial time points, the C/EBPα and PPARγ were found significantly elevated around days 0-3 (p<0.001, p=0.007, respectively) and days 10-21 (p<0.001, p<0.001 respectively). GPD2 exhibited continuous, significant changes at days 3, 21 and 28 (p=0.002, p<0.001 and p<0.001, respectively). The expression of FABP4 remained relatively low for the first 2 weeks but, significantly increased on day 21 (p<0.001). *The change in transcripts between serial time points was significant on paired t-test. p<0.05. C/EBPα, CCAAT/enhancer binding protein α; GPD2, glycerol 3-phosphate dehydrogenase; PPARγ, peroxisome proliferator-activated receptor γ; FABP4, fatty acid binding protein 4.
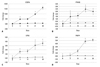
References
1. Pittenger MF, Mackay AM, Beck SC, Jaiswal RK, Douglas R, Mosca JD, et al. Multilineage potential of adult human mesenchymal stem cells. Science. 1999. 284:143–147.


2. Ferrari G, Cusella-De Angelis G, Coletta M, Paolucci E, Stornaiuolo A, Cossu G, et al. Muscle regeneration by bone marrow-derived myogenic progenitors. Science. 1998. 279:1528–1530.


3. Sanchez-Ramos J, Song S, Cardozo-Pelaez F, Hazzi C, Stedeford T, Willing A, et al. Adult bone marrow stromal cells differentiate into neural cells in vitro. Exp Neurol. 2000. 164:247–256.


4. Beyer Nardi N, da Silva Meirelles L. Mesenchymal stem cells: isolation, in vitro expansion and characterization. Handb Exp Pharmacol. 2006. 249–282.


5. Dvash T, Mayshar Y, Darr H, McElhaney M, Barker D, Yanuka O, et al. Temporal gene expression during differentiation of human embryonic stem cells and embryoid bodies. Hum Reprod. 2004. 19:2875–2883.


6. Yamamoto Y, Banas A, Murata S, Ishikawa M, Lim CR, Teratani T, et al. A comparative analysis of the transcriptome and signal pathways in hepatic differentiation of human adipose mesenchymal stem cells. FEBS J. 2008. 275:1260–1273.


7. Wang Q, Yu JH, Zhai HH, Zhao QT, Chen JW, Shu L, et al. Temporal expression of estrogen receptor alpha in rat bone marrow mesenchymal stem cells. Biochem Biophys Res Commun. 2006. 347:117–123.


8. Hu Y, Liao L, Wang Q, Ma L, Ma G, Jiang X, et al. Isolation and identification of mesenchymal stem cells from human fetal pancreas. J Lab Clin Med. 2003. 141:342–349.


9. in 't Anker PS, Noort WA, Scherjon SA, Kleijburg-van der Keur C, Kruisselbrink AB, van Bezooijen RL, et al. Mesenchymal stem cells in human second-trimester bone marrow, liver, lung, and spleen exhibit a similar immunophenotype but a heterogeneous multilineage differentiation potential. Haematologica. 2003. 88:845–852.
10. Campagnoli C, Roberts IA, Kumar S, Bennett PR, Bellantuono I, Fisk NM. Identification of mesenchymal stem/progenitor cells in human first-trimester fetal blood, liver, and bone marrow. Blood. 2001. 98:2396–2402.


11. Battula VL, Bareiss PM, Treml S, Conrad S, Albert I, Hojak S, et al. Human placenta and bone marrow derived MSC cultured in serum-free, b-FGF-containing medium express cell surface frizzled-9 and SSEA-4 and give rise to multilineage differentiation. Differentiation. 2007. 75:279–291.


12. In't Anker PS, Scherjon SA, Kleijburg-van der, de Groot-Swings GM, Claas FH, Fibbe WE, et al. Isolation of mesenchymal stem cells of fetal or maternal origin from human placenta. Stem Cells. 2004. 22:1338–1345.
13. Miki T, Lehmann T, Cai H, Stolz DB, Strom SC. Stem cell characteristics of amniotic epithelial cells. Stem Cells. 2005. 23:1549–1559.


14. Mitchell KE, Weiss ML, Mitchell BM, Martin P, Davis D, Morales L, et al. Matrix cells from Wharton's jelly form neurons and glia. Stem Cells. 2003. 21:50–60.


15. Portmann-Lanz CB, Schoeberlein A, Huber A, Sager R, Malek A, Holzgreve W, et al. Placental mesenchymal stem cells as potential autologous graft for pre- and perinatal neuroregeneration. Am J Obstet Gynecol. 2006. 194:664–673.


16. Otto TC, Lane MD. Adipose development: from stem cell to adipocyte. Crit Rev Biochem Mol Biol. 2005. 40:229–242.


17. Hutley LJ, Newell FM, Joyner JM, Suchting SJ, Herington AC, Cameron DP, et al. Effects of rosiglitazone and linoleic acid on human preadipocyte differentiation. Eur J Clin Invest. 2003. 33:574–581.


18. Soncini M, Vertua E, Gibelli L, Zorzi F, Denegri M, Albertini A, et al. Isolation and characterization of mesenchymal cells from human fetal membranes. J Tissue Eng Regen Med. 2007. 1:296–305.


19. Livak KJ, Schmittgen TD. Analysis of relative gene expression data using real-time quantitative PCR and the 2(-Delta Delta C(T)) Method. Methods. 2001. 25:402–408.


20. Umek RM, Friedman AD, McKnight SL. CCAAT-enhancer binding protein: a component of a differentiation switch. Science. 1991. 251:288–292.


21. Tontonoz P, Hu E, Graves RA, Budavari AI, Spiegelman BM. mPPAR gamma 2: tissue-specific regulator of an adipocyte enhancer. Genes Dev. 1994. 8:1224–1234.


22. Rosen ED, Hsu CH, Wang X, Sakai S, Freeman MW, Gonzalez FJ, et al. C/EBPalpha induces adipogenesis through PPARgamma: a unified pathway. Genes Dev. 2002. 16:22–26.
23. Shao D, Lazar MA. Peroxisome proliferator activated receptor gamma, CCAAT/enhancer-binding protein alpha, and cell cycle status regulate the commitment to adipocyte differentiation. J Biol Chem. 1997. 272:21473–21478.


24. Wu Z, Rosen ED, Brun R, Hauser S, Adelmant G, Troy AE, et al. Cross-regulation of C/EBP alpha and PPAR gamma controls the transcriptional pathway of adipogenesis and insulin sensitivity. Mol Cell. 1999. 3:151–158.


25. Auwerx J, Martin G, Guerre-Millo M, Staels B. Transcription, adipocyte differentiation, and obesity. J Mol Med (Berl). 1996. 74:347–352.


26. Shin DW, Kim SN, Lee SM, Lee W, Song MJ, Park SM, et al. (-)-Catechin promotes adipocyte differentiation in human bone marrow mesenchymal stem cells through PPAR gamma transactivation. Biochem Pharmacol. 2009. 77:125–133.


27. Chang YJ, Shih DT, Tseng CP, Hsieh TB, Lee DC, Hwang SM. Disparate mesenchyme-lineage tendencies in mesenchymal stem cells from human bone marrow and umbilical cord blood. Stem Cells. 2006. 24:679–685.


28. Karahuseyinoglu S, Kocaefe C, Balci D, Erdemli E, Can A. Functional structure of adipocytes differentiated from human umbilical cord stroma-derived stem cells. Stem Cells. 2008. 26:682–691.


29. Sekiya I, Larson BL, Vuoristo JT, Cui JG, Prockop DJ. Adipogenic differentiation of human adult stem cells from bone marrow stroma (MSCs). J Bone Miner Res. 2004. 19:256–264.


30. Kim HK, Im EH, Park H, Cho JA, Yang DY, Kim KH, et al. Characterization and differentiation into adipocytes of mesenchymal stem cells (MSCs) from human adipose tissue and amniotic fluid. Korean J Obstet Gynecol. 2009. 52:447–455.