Abstract
Purpose
Dexmedetomidine, a full agonist of α2B-adrenoceptors, is used for analgesia and sedation in the intensive care units. Dexmedetomidine produces an initial transient hypertension due to the activation of post-junctional α2B-adrenoceptors on vascular smooth muscle cells (SMCs). The aims of this in vitro study were to identify mitogen-activated protein kinase (MAPK) isoforms that are primarily involved in full, α2B-adrenoceptor agonist, dexmedetomidine-induced contraction of isolated rat aortic SMCs.
Materials and Methods
Rat thoracic aortic rings without endothelium were isolated and suspended for isometric tension recording. Cumulative dexmedetomidine (10-9 to 10-6 M) dose-response curves were generated in the presence or absence of extracellular signal-regulated kinase (ERK) inhibitor PD 98059, p38 MAPK inhibitor SB 203580, c-Jun NH2-terminal kinase (JNK) inhibitor SP 600125, L-type calcium channel blocker (verapamil and nifedipine), and α2-adrenoceptor inhibitor atipamezole. Dexmedetomidine-induced phosphorylation of ERK, JNK, and p38 MAPK in rat aortic SMCs was detected using Western blotting.
Results
SP 600125 (10-6 to 10-5 M) attenuated dexmedetomidine-evoked contraction in a concentration-dependent manner, whereas PD 98059 had no effect on dexmedetomidine-induced contraction. SB 203580 (10-5 M) attenuated dexmedetomidine-induced contraction. Dexmedetomidine-evoked contractions were both abolished by atipamezole and attenuated by verapamil and nifedipine. Dexmedetomidine induced phosphorylation of JNK and p38 MAPK in rat aortic SMCs, but did not induce phosphorylation of ERK.
The highly selective α2-adrenergic agonist dexmedetomidine has a relative α2/α1 selectivity ratio of 1,620, which is 5-10 times greater than that of other compounds (e.g., α2/α1 selectivity ratio: clonidine, 220; UK 14304, 300).1 Dexmedetomidine is a full agonist of α2B-adrenoceptors and a partial agonist of α2A- and α2C-adrenoceptors.2 In humans and mammals, dexmedetomidine produces an initial transient increase in blood pressure after intravenous injection.3-8 In addition, intravenous doses of dexmedetomidine produce transient systemic and coronary vasoconstriction.6-8 Activation of post-junctional α2B-adrenoceptors on vascular smooth muscle is responsible for the initial transient hypertension.9,10
In response to receptor stimulation, Ca2+ is mobilized from intracellular stores and/or the extracellular space to increase cytosolic Ca2+ concentrations in vascular smooth muscle cells (SMCs), leading to the activation of the Ca2+-calmodulin-myosin light chain kinase (MLCK) pathway.11 In addition to increased cytosolic Ca2+-induced contraction, contraction mediated by protein kinases involving Rho-kinase, protein kinase C (PKC), mitogen-activated protein kinase (MAPK), and receptor tyrosine kinase is believed to play important roles in agonist-induced contraction.11,12 Such protein kinase-mediated contraction leads to the phosphorylation of 20-kDa myosin regulatory light chain (MLC20) and inhibition of myosin light chain phosphatase (MLCP).11,12 MAPKs are serine-threonine protein kinases that consist of three isoforms: extracellular signal-regulated kinase (ERK), p38 MAPK, and c-Jun NH2-terminal kinase (JNK).13 A growing body of evidence demonstrates that MAPK pathway activation via G protein-coupled receptors is involved in the modulation of contraction in vascular smooth muscle.14,15 ERK contributes to contractions induced by the partial α2B-adrenoceptor agonist UK 14304 in blood vessles.16,17 As peripheral vasoconstriction in response to α2-agonists primarily involves the α2B-adrenoceptor, MAPK isoforms activated by α2-adrenergic agonists may differ for each α2-adrenoceptor agonist, depending on the agonist's affinity for the α2B-adrenoceptor subtype.2,9,10 However, from the best knowledge available to the authors, the MAPK isoform principally involved in the dexmedetomidine-induced contraction in isolated rat aortic SMCs has not been previously identified. The goal of the current in vitro study was to identify the MAPK isoform that is mainly involved in the dexmedetomidine-induced contraction of isolated rat aortic SMCs.
All experimental procedures and protocols were approved by the Institutional Animal Care and Use Committee at Gyeongsang National University.
Male Sprague Dawley rats weighing 250-350 g each were anesthetized by intraperitoneal administration of pentobarbital sodium (50 mg/kg). The aortic rings for tension measurement were prepared according to the method described in the previous studies.18,19 The descending thoracic aorta was dissected free, and surrounding connective tissues and fat were removed under microscopic guidance while aorta was bathed in Krebs solution composed of following components: 118 mM NaCl, 4.7 mM KCl, 1.2 mM MgSO4, 1.2 mM KH2PO4, 2.4 mM CaCl2, 25 mM NaHCO3, 11 mM glucose, and 0.03 mM EDTA. The aorta was then cut into 2.5-mm rings and suspended on Grass isometric transducers (FT-03, Grass Instrument, Quincy, MA, USA) under a 3.0-g resting tension in a 10-mL Krebs bath at 37℃, aerated continuously with 95% O2 and 5% CO2 to maintain pH values within the 7.35-7.45 range. The rings were equilibrated at a 3.0-g resting tension for 120 min, during which time bathing solution was changed every 30 min. In all aortic rings, the endothelium was intentionally removed from the aortic rings by inserting a 25-gauge needle into the lumen of the rings and gently rubbing the ring for a few seconds. When the contraction in response to phenylephrine (10-7 M) had stabilized, endothelial removal was confirmed by the absence of a relaxation response to acetylcholine (10-5 M). The contractile response evoked by isotonic 30 mM KCl was measured for all aortic rings and used as a reference value. The isotonic 30 mM KCl solution was prepared by replacing the NaCl in the Krebs solution with an equimolar amount of KCl. After washing out the KCl from the organ bath and allowing the isometric tension to return to baseline, a cumulative concentration-response curve to either dexmedetomidine or levomedetomidine was obtained as described below. A single ring was used for each concentration-response curve. The cyclooxygenase inhibitor indomethacin (10-5 M) and the nitric oxide synthase inhibitor NW-nitro-L-arginine methyl ester (L-NAME, 10-5 M) were also included in the Krebs solution to prevent the release of endogenous prostaglandins and nitric oxide, respectively, from any residual endothelium.
The first series of experiments assessed the cumulative concentration-response curves to the medetomidine enantiomers dexmedetomidine or levomedetomidine (10-9 to 10-6 M) in the endothelium-denuded rings. A subsequent concentration of dexmedetomidine was added after the previous concentration elicited a sustained and stable contraction for 5 min.
The second series of experiments was designed to determine which isoform of MAPK is functionally important in mediating dexmedetomidine-induced contraction. The effect of MAPK inhibitors (ERK inhibitor: 10-5 M PD 98059, p38 MAPK inhibitor: 10-6 and 10-5 M SB 203580, JNK inhibitor: 10-6, 3×10-6, and 10-5 M SP 600125) on the dexmedetomidine concentration-response curve was assessed by comparing each dexmedetomidine-induced contraction in the presence or absence of each MAPK inhibitor. The incubation period for each MAPK inhibitor was 20 min prior to addition of dexmedetomidine. Inhibitor concentrations were chosen based on the reported 50% inhibitory concentration.12,20
The third series of experiments was designed to examine the effect of dexmedetomidine on the voltage-operated calcium channel-induced contraction. The initial baseline response induced by isotonic 30 mM KCl was recorded in the endothelium-denuded aorta. After washing out KCl from the organ bath and allowing it to return to the baseline resting tension, the second isotonic 30 mM KCl-induced contraction in the same aorta was determined by adding 30 mM KCl solution containing dexmedetomidine (0, 3×10-8, 10-7, 3×10-7, or 10-6 M) to the organ bath.
Finally, the effects of the L-type calcium channel blocker (10-5 M verapamil and 5×10-6 M nifedipine) and the α2-adrenoceptor antagonist atipamezole (10-4 M) on the dexmedetomidine concentration-response curve were assessed by comparing cumulative dexmedetomidine concentration-response curves in the presence and absence of each inhibitor. Each inhibitor was added to the organ bath 20 min prior to the addition of dexmedetomidine.
The cells were isolated from the rat thoracic aorta by enzymatic dissociation and grown in Dulbecco's modified eagle medium (DMEM) supplemented with 10% heat-inactivated fetal bovine serum (FBS), 2 mM L-glutamine, 100 U/mL penicillin, and 100 µg/mL streptomycin. The cells were subcultured twice a week by harvesting with trypsin/EDTA and seeding in 7.5×105 /mm2 flasks. For experiments, the cells between passage numbers 2 and 10 were seeded into dishes (2 cells/mm2), fed every other day, and used at confluence (6-7 days). The cells were deprived of serum overnight prior to treatment.
Western blot analysis was performed according to the method described in the previous study.21 In brief, the cells were lysed in PRO-PREP protein extract solution for total cell lysates, and the lysates were centrifuged at 100,000×g for 20 min at 4℃. Protein concentration was determined using the Bradford method. For preparation of sample loading, equal volumes of 2×sodium dodecyl sulfate (SDS) sample buffer (0.1 mol/L Tris-HCI, 20% glycerol, 4% SDS, and 0.01% bromophenol blue) and supernatant fractions from the lysates were mixed. Proteins (60 microgram) were separated by 10% SDS-polyacrylamide gel electrophoresis for 90 min at 110 V. The separated proteins were transferred to polyvinylidene difluoride (PVDF) membranes for 2 h at 20 mA using SD Semi-dry Transfer Cells (Bio-Rad Laboratories, Hercules, CA, USA). After blocking the membranes using 5% nonfat milk in Tris-buffed saline (TBS, pH 7.0), the membranes were incubated overnight at 4℃ with specific antibodies at a dilution of 1 : 500 in 5% skim milk in TBS-T. Bound antibody was detected by horseradish peroxidase (HRP)-conjugated anti-goat or anti-rabbit IgG. The membranes were washed and developed using a Western Blotting Luminol Reagent System (iNtRON Biotechnology, Houston, TX, USA) and autoradiography.
All drugs were of the highest purity available commercially: acetylcholine, L-NAME, indomethacin, verapamil, nifedipine, PD 98059, SB 203580, and SP 600125 were obtained from Sigma-Aldrich (St. Louis, MO, USA). Dexmedetomidine, levomedetomidine, and atipamezole were kind gifts from Orion Pharma (Turku, Finland). All concentrations are expressed as the final molar concentration in the organ bath. DMEM, FBS, penicillin, streptomycin, trypsin/EDTA, and glutamine were supplied by Gibco BRL (Rockville, MD, USA). PRO-PREP protein extract solution and electrochemiluminescence (ECL) Western blotting detection reagents were supplied by iNtRON Biotechnology (Houston, TX, USA). Anti-phospho-ERK and anti-ERK antibodies were purchased from Santa Cruz Biotechnology (Santa Cruz, CA, USA). Anti-phospho-JNK, anti-JNK, anti-phospho-p38 MAPK, and anti-p38 MAPK antibodies were obtained from Cell Signaling Technology (Beverly, MA, USA). Nifedipine, PD 98059, SB 203580, and SP 600125 were dissolved in dimethyl sulfoxide (DMSO) (final organ bath concentration: 0.1% DMSO) as was indomethacin. Unless stated otherwise, all other drugs were dissolved and diluted in distilled water.
Values are expressed as the mean±SD of n rats from which descending thoracic aortic rings were obtained. Contractile responses to dexmedetomidine and levomedetomidine are expressed as a percentage of the maximum contraction to 30 mM isotonic KCl. Maximal contraction was defined as the contractile response to 10-6 M dexmedetomidine. Statistical analysis for the comparison of maximal contractions between the no-drug and inhibitor-treated groups was performed using the Student's t-test for paired samples or a one-way analysis of variance (ANOVA) by Tukey's multiple comparison test. Band intensities from the Western blot analyses were assessed by scanning densitometry, and statistical analysis was performed using the Mann-Whitney U test. Scanning densitometry was performed using an Image Master® VSD (Pharmacia Biotech Inc., San Francisco, CA, USA). The effect of dexmedetomidine on the 30 mM KCl-induced contraction was analyzed by an unpaired Student's t-test. Responses to each concentration of dexmedetomidine were compared using repeated measures ANOVA followed by a Tukey's multiple comparison test. A p value of less than 0.05 was considered statistically significant.
Dexmedetomidine produced concentration-dependent contractions in endothelium-denuded rings (p<0.001: 10-7 to 10-6 M versus 10-9 M), whereas levomedetomidine produced no contractile response (maximal contraction: p<0.001 versus dexmedetomidine) (Fig. 1).
The ERK inhibitor PD 98059 (10-5 M), p38 MAPK inhibitor SB 203580 (10-5 M), and JNK inhibitor SP 600125 (10-5 M) did not significantly affect the baseline resting tension. PD 98059 (10-5 M) had no effect on dexmedetomidine-induced contraction (Fig. 2A). SB 203580 (10-5 M) attenuated dexmedetomidine-induced maximal contractions (maximal contraction: p<0.001 versus no drug) (Fig. 2B). SP 600125 (10-6, 3×10-6, and 10-5 M) attenuated dexmedetomidine-induced maximal contractions in a concentration-dependent manner (maximal contraction: p<0.001 versus no drug) (Fig. 2C).
Dexmedetomidine (3×10-8 to 10-6 M) increased 30 mM KCl-induced contraction (3×10-8 and 3×10-7 M: p=0.002 versus no drug; 10-7 M: p=0.001 versus no drug; 10-6 M: p=0.006 versus no drug) (Fig. 3A). The maximum contractile effect of dexmedetomidine in endothelium-denuded rings was markedly attenuated by verapamil (10-5 M) and nifedipine (5×10-6 M) relative to responses in the absence of each inhibitor (maximal contraction: p<0.001 versus no drug) (Fig. 3B, C). Dexmedetomidine-induced contractions were completely abolished by atipamezole (10-4 M) (maximal contraction: p<0.001 versus no drug) (Fig. 3B).
The above results (Fig. 2) indicate the involvement of JNK and p38 MAPK in dexmedetomidine-induced contractions. Thus, we examined whether dexmedetomidine (10-7 and 10-6 M) induces activation of JNK and p38 MAPK pathways in rat aortic SMCs (Fig. 4A). JNK phosphorylation was induced at both 5 minutes and 10 minutes by dexmedetomidine (10-6 M) (Fig. 4A). p38 MAPK phosphorylation was induced at 10, 30, and 60 minutes by dexmedetomidine (10-6 M) (Fig. 4A). As expected, the ERK1/2 pathway was not activated at 5, 10, 30 and 60 minutes by dexmedetomidine (10-7 or 10-6 M) (Fig. 4A). Fig. 4B shows that dexmedetomidine (10-6 M) treatment induced phosphorylation of p38 and JNK, but not ERK1/2, at 10 minutes compared to control (0 minute).
Despite the increased use of dexmedetomidine for analgesia and sedation in intensive care units, we believe that the present study is the first to investigate the specific MAPK isoforms involved in dexmedetomidine-induced contractions of rat aortic SMCs.22 The major findings of the present study are as follows: 1) SP 600125 and SB 203580 attenuated dexmedetomidine-induced contractions, whereas PD 98059 had no effect on dexmedetomidine-induced contractions; 2) dexmedetomidine induced phosphorylation of JNK and p38 MAPK, whereas dexmedetomidine had no effect on ERK phosphorylation; 3) dexmedetomidine-induced contractions were attenuated by the L-type calcium channel antagonist (verapamil and nifedipine), indicating a role for extracellular Ca2+; these contractions were virtually abolished by a high concentration of the α2-adrenoceptor antagonist atipamezole.
The L-isomer of medetomidine has no effect on hemodynamics.3 In agreement with a previous study by Schmeling, et al.,3 levomedetomidine failed to contract endothelium-denuded rings, suggesting that the effect of dexmedetomidine is stereoselective.
Protein kinases, including Rho-kinase, PKC, and MAPK, mediate agonist-induced contractions.11,12 Three major MAPK families have been identified (i.e., ERK, JNK, and p38 MAPK) in vascular SMCs.13 Several lines of evidence suggest that the ERK-dependent MAPK pathway is activated by receptor agonists such as phenylephrine, angiotensin II, and 5-hydroxytryptamine, producing contraction of vascular smooth muscle.23-25 PD 98059 has been shown to attenuate contractions in response to UK 14304 in isolated endothelium-denuded rat aorta and porcine palmar lateral veins, suggesting that UK 14304-induced contractions involve ERK pathway activation.16,17 In contrast with previous reports, PD 98059 did not alter the dexmedetomidine concentration-response curve in this study, while dexmedetomidine-induced contractions were attenuated by SP 600125 and SB 203580, suggesting that the JNK and p38 MAPK pathways are involved in dexmedetomidine-induced contractions.16,17 In addition, both postsynaptic α2A- and α2B-adrenoceptors are present in rat aortas.26 This finding may be due to differences in α2-adrenoceptor subtype affinities, α2-adrenoceptor subtype distributions, incubation period and α2/α1 selectivity ratios, as well as the fact that the α2B-adrenoceptor is the main α2-adrenoceptor subtype involved in vascular smooth muscle contraction.1,2,9,10,27 However, as SP 600125 also non-specifically inhibits MLCK, which is involved in vascular smooth muscle contraction, we cannot rule out the possibility that the inhibition of MLCK induced by SP 600125 may attenuate dexmedetomidine-induced contraction.28 Thus, we should be very cautious about interpreting data using SP 600125 as inhibitor of JNK. If a potent and specific inhibitor that is selective for JNK can be developed, further study using this selective inhibitor will be needed.
Dexmedetomidine and clonidine enhance high potassium-induced contractions in isolated endothelium-denuded vessels.29,30 In accordance with previous studies, dexmedetomidine augmented 30 mM KCl-induced contractions in this in vitro study, suggesting that dexmedetomidine has a synergistic effect on voltage-operated calcium channel-induced contraction.29,30 Verapamil and nifedipine attenuated contractile responses to dexmedetomidine, suggesting that dexmedetomidine-induced contraction involves L-type calcium channels activation. These results indicate that the major signaling mechanism for dexmedetomidine-induced contractions is associated with the Ca2+-calmodulin-MLCK pathway. However, although verapamil attenuated dexmedetomidine-induced contraction, we could not rule out the possibility that the inhibition of α2-adrenoceptors induced by verapamil may attenuate dexmedetomidine-induced contraction because verapamil can also bind to α2-adrenoceptors.31 Another L-type calcium channel blocker, nifedipine, which does not compete for [3H] yohimbine binding to alpha-2 adrenoceptors, attenuated dexmedetomidine-induced contraction, indicating a significant role for extracellular Ca2+ entry through L-type calcium channels in the calcium-dependent mechanism for dexmedetomidine-induced contraction.31 Dexmedetomidine-induced contractions of rat aortas involve activation of the lipoxygenase pathway.32 Metabolites generated by the lipoxygenase pathway greatly accelerate calcium entry, favoring smooth muscle contraction.33 Taken together, these results suggest that activation of the lipoxygenase pathway involved in dexmedetomidine-induced contractions may be associated with L-type calcium channel-induced contractions in aortic smooth muscle.
To confirm whether JNK and p38 MAPK mediate dexmedetomidine-induced contractions, Western blot analyses were performed. In agreement with results from isometric tension measurements in this in vitro study, dexmedetomidine was shown to induce phosphorylation of both JNK and p38 MAPK in rat aortic SMCs, while dexmedetomidine did not induce phosphorylation of ERK. UK 14304-induced contraction increases ERK phosphorylation in porcine palmar lateral veins.17 Treatment of the renal tubular cell line LLC-PK1-α2B with dexmedetomidine activates ERK via a pathway involving arachidonic acid metabolism.34 Dexmedetomidine is a full agonist of α2B-adrenoceptors, whereas UK 14304 is a partial agonist of α2B-adrenoceptors.2 Thus, this finding may be due to differences in α2-adrenoceptor subtype affinities and species.2 As SP 600125 attenuated dexmedetomidine-induced maximal contractions in a concentration-dependent manner, these results suggest that dexmedetomidine-induced contraction is primarily mediated by activation of a JNK-mediated pathway and, in part, by activation of a p38 MAPK-mediated pathway. There are several cross talk points among the signal transduction pathways of protein kinase-mediated contraction.12 Therefore, it is very difficult to fully elucidate the signaling pathways involving JNK and p38 MAPK that are responsible for dexmedetomidine-induced contractions.
Dexmedetomidine produces an initial transient increase in blood pressure, followed by a long-lasting reduction in blood pressure in both humans and animals.3-8,35 This initial, transient increase in blood pressure may be ascribed to the time differences between the direct binding of dexmedetomidine on α2B-adrenoceptors of vascular SMCs and the diffusion of dexmedetomidine into the central nervous system to decrease sympathetic activity; these time differences might be unavoidable effects of dexmedetomidine and may be associated with both dosage and intravenous injection speed.4,5 Oral and buccal administration of dexmedetomidine in humans did not produce initial transient hypertension, suggesting that these time differences were eliminated.36,37 In the current study, a dexmedetomidine concentration (10-8 M) that corresponds approximately to the plasma concentration (2×10-9 to 8×10-9 M) of dexmedetomidine used for sedation in humans did not produce any contractile responses, but supraclinical doses (10-7 to 10-6 M) of dexmedetomidine did so.38 However, any clinical implication of dexmedetomidine on regional hemodynamics must be tempered by the fact that the aorta was used in this in vitro experiment, whereas organ blood flow is controlled by changes in the diameters of arterioles with diameters less than 150 µm. Despite this limitation, taking into consideration results from previous studies, as well as the current results, these findings suggest that dexmedetomidine-induced JNK- and p38 MAPK-mediated contraction may contribute to the initial transient increase in blood pressure after dexmedetomidine infusion.3-8,35 Dexmedetomidine-induced contractions in vascular smooth muscle are primarily attenuated by endothelial nitric oxide produced via stimulation of endothelial α2-adrenoceptors by dexmedetomidine.29,32,39 Taken together, these results suggest that patients, with compromised endothelial integrity, such as those with diabetes, hypertension, and atherosclerosis, may exhibit extremely high blood pressures due to exaggerated dexmedetomidine-induced vasoconstriction.
In conclusion, these results indicate that contraction induced by dexmedetomidine involves both JNK- and p38 MAPK-mediated pathways downstream of α2-adrenoceptor stimulation in rat aortic SMCs. In addition, dexmedetomidine-induced contractions are primarily dependent on calcium influx via L-type calcium channels.
Figures and Tables
Fig. 1
Medetomidine enantiomers concentration-response curves in endothelium-denuded rings. Data represent the mean±SD and are expressed as the percentage of the maximal contraction induced by isotonic 30 mM KCl (isotonic 30 mM KCl-induced contraction: 100%=2.30±0.60 g [n=8] and 100%=1.90±0.20 g [n=8] for endothelium-denuded rings with dexmedetomidine and levomedetomidine, respectively). *p<0.001 compared with 10-9 M dexmedetomidine. Maximal contraction: †p<0.001 compared with levomedetomidine.
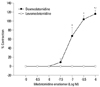
Fig. 2
Effects of PD 98059 (10-5 M), SB 203580 (10-6, 10-5 M), and SP 600125 (10-6, 3×10-6, and 10-5 M) on dexmedetomidine concentration-response curves in endothelium-denuded rings. All data represent the mean±SD and are expressed as the percentage of the maximal contraction induced by isotonic 30 mM KCl. (A) Isotonic 30 mM KCl-induced contraction: 100%=2.42±0.63 g (n=6) and 100%=2.51±0.21 g (n=6) for endothelium-denuded rings with no drug and 10-5 M PD 98059-pretreated endothelium-denuded rings, respectively. (B) Isotonic 30 mM KCl-induced contraction: 100%=2.57±0.29 g (n=7), 100%=2.81±0.30 g (n=6), and 100%=2.21±0.52 g (n=7) for endothelium-denuded rings with no drug and SB 203580 (10-6 M)- and (10-5 M)-pretreated endothelium-denuded rings, respectively. Maximal contraction: *p<0.001 compared with no drug. (C) Isotonic 30 mM KCl-induced contraction: 100%=2.50±0.42 g (n=7), 100%=2.43±0.42 g (n=6), 100%=2.34±0.29 g (n=7) and 100%=2.50±0.31 g (n=5) for endothelium-denuded rings with no drug and SP 600125 (10-6 M)-, (3×10-6 M)-, and (10-5 M)-pretreated endothelium-denuded rings, respectively. Maximal contraction: *p<0.001 compared with no drug, †p<0.001 compared with 10-6 M SP 600125, ‡p<0.001 compared with 3×10-6 M SP 600125.
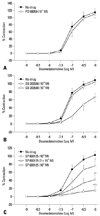
Fig. 3
(A) Effect of dexmedetomidine (DMT, 3×10-8, 10-7, 3×10-7, 10-6 M) on 30 mM potassium chloride (KCl)-induced contraction in endothelium-denuded aorta. Data represent the mean±SD and are expressed as the relative percentage of the maximal contraction induced by initial 30 mM KCl (100%=2.75±0.48 g [n=6], 100%=2.71±0.78 g [n=6], 100%=2.83±0.47 g [n=6], 100%=2.68±0.45 g [n=6] and 100%=2.41±0.28 g [n=5] for endothelium-denuded aorta with no drug, 3×10-8 DMT, 10-7 DMT, 3×10-7 DMT and 10-6 M DMT, respectively). *p=0.002, †p=0.001, ‡p=0.002 and §p=0.006 compared with no drug. (B) Effects of verapamil and atipamezole on dexmedetomidine concentration- response curves in endothelium-denuded rings. Data represent the mean±SD and are expressed as the percentage of the maximal contraction induced by isotonic 30 mM KCl (isotonic 30 mM KCl-induced contraction: 100%=2.37±0.53 g [n=6], 100%=2.64±0.59 g [n=5] and 100%=1.47±0.24 g [n=5] for endothelium-denuded rings with no drug, the verapamil (10-5 M)- and atipamezole (10-4 M)-pretreated endothelium-denuded rings, respectively). Maximal contraction: *p<0.001 compared with no drug. (C) Effects of nifedipine on dexmedetomidine concentration-response curves in endothelium-denuded rings. Data represent the mean±SD and are expressed as the percentage of the maximal contraction induced by isotonic 30 mM KCl (isotonic 30 mM KCl-induced contraction: 100%=2.44±0.51 g [n=6] and 100%=2.45±0.48 g [n=6] for endothelium-denuded rings with no drug and the nifedipine (5×10-6 M)-pretreated endothelium-denuded rings, respectively). Maximal contraction: *p<0.001 compared with no drug.
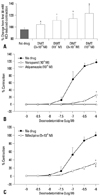
Fig. 4
Effect of dexmedetomidine (DMT) on the activation of exracellular signal-regulated kinase (ERK), p38 mitogen-activated protein kinase (MAPK), and c-Jun NH2-terminal kinase (JNK) in rat aortic vascular smooth muscle cells (VSMCs). VSMCs were treated with DMT (10-7 or 10-6 M) in a time-dependent manner (5, 10, 30, or 60 min). (A) Phosphorylation of ERK, p38 MAPK, and JNK was examined by Western blot analysis as described in the methods. (B) Band intensities at 10 min after 10-6 M dexmedetomidine pretreatment were assessed by scanning densitometry. Data are presented as the mean±SD of three independent experiments. *p=0.039 and †p=0.045 compared with control.
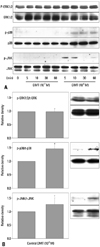
References
1. Virtanen R, Savola JM, Saano V, Nyman L. Characterization of the selectivity, specificity and potency of medetomidine as an alpha 2-adrenoceptor agonist. Eur J Pharmacol. 1988. 150:9–14.


2. Peltonen JM, Pihlavisto M, Scheinin M. Subtype-specific stimulation of [35S]GTPgammaS binding by recombinant alpha 2-adrenoceptors. Eur J Pharmacol. 1998. 355:275–279.


3. Schmeling WT, Kampine JP, Roerig DL, Warltier DC. The effects of the stereoisomers of the alpha 2-adrenergic agonist medetomidine on systemic and coronary hemodynamics in conscious dogs. Anesthesiology. 1991. 75:499–511.


4. Bloor BC, Ward DS, Belleville JP, Maze M. Effects of intravenous dexmedetomidine in humans. II. Hemodynamic changes. Anesthesiology. 1992. 77:1134–1142.
5. Hall JE, Uhrich TD, Barney JA, Arain SR, Ebert TJ. Sedative, amnestic, and analgesic properties of small-dose dexmedetomidine infusions. Anesth Analg. 2000. 90:699–705.


6. Lawrence CJ, Prinzen FW, de Lange S. Hemodynamic and coronary vascular effects of dexmedetomidine in the anesthetized goat. Acta Anaesthesiol Scand. 1997. 41:830–836.


7. Jalonen J, Halkola L, Kuttila K, Perttilä J, Rajalin A, Savunen T, et al. Effects of dexmedetomidine on coronary hemodynamics and myocardial oxygen balance. J cardiothorac Vasc Anesth. 1995. 9:519–524.


8. Flacke WE, Flacke JW, Bloor BC, Mclntee DF, Sagan M. Effects of dexmedetomidine on systemic and coronary hemodynamics in the anesthetized dog. J Cardiothorac Vasc Anesth. 1993. 7:41–49.


9. Guimaräes S, Moura D. Vascular adrenoceptors: an update. Pharmacol Rev. 2001. 53:319–356.
10. Mizobe T. [Adrenergic receptor and knockout mouse: 2). Alpha adrenergic receptor knockout mouse]. Masui. 2001. 50:12–19.
11. Akata T. General anesthetics and vascular smooth muscle: direct actions of general anesthetics on cellular mechanisms regulating vascular tone. Anesthesiology. 2007. 106:365–391.
12. Abdel-Latif AA. Cross talk between cyclic nucleotides and polyphosphoinositide hydrolysis, protein kinases, and contraction in smooth muscle. Exp Biol Med (Maywood). 2001. 226:153–163.


13. Khan TA, Bianchi C, Ruel M, Voisine P, Sellke FW. Mitogen-activated protein kinase pathways and cardiac surgery. J Thorac Cardiovasc Surg. 2004. 127:806–811.


14. Kim B, Kim J, Bae YM, Cho SI, Kwon SC, Jung JY, et al. p38 mitogen-activated protein kinase contributes to the diminished aortic contraction by endothelin-1 in DOCA-salt hypertensive rats. Hypertension. 2004. 43:1086–1091.


15. Lee YR, Lee CK, Park HJ, Kim H, Kim J, Kim J, et al. C-Jun N-terminal kinase contributes to norepinephrine-induced contraction through phosphorylation of caldesmon in rat aortic smooth muscle. J Pharmacol Sci. 2006. 100:119–125.


16. Carter RW, Kanagy NL. Tyrosine kinases regulate intracellular calcium during alpha (2)-adrenergic contraction in rat aorta. Am J Physiol Heart Circ Physiol. 2002. 283:H1673–H1680.
17. Roberts RE. Role of the extracellular signal-regulated kinase (Erk) signal transduction cascade in alpha (2) adrenoceptor-mediated vasoconstriction in porcine palmar lateral vein. Br J Pharmacol. 2001. 133:859–866.


18. Choi YS, Jeong YS, Ok SH, Shin IW, Lee SH, Park JY, et al. The direct effect of levobupivacaine in isolated rat aorta involves lipoxygenase pathway activation and endothelial nitric oxide release. Anesth Analg. 2010. 110:341–349.


19. Sung HJ, Sohn JT, Park JY, Hwang EM, Baik JS, Ogawa K. Direct effect of ropivacaine involves lipoxygenase pathway activation in rat aortic smooth muscle. Can J Anaesth. 2009. 56:298–306.


20. Klegeris A, Giasson BI, Zhang H, Maguire J, Pelech S, McGeer PL. Alpha-synuclein and its disease-causing mutants induce ICAM-1 and IL-6 in human astrocytes and astrocytoma cells. FASEB J. 2006. 20:2000–2008.


21. Park MK, Kang YJ, Lee HS, Kim HJ, Seo HG, Lee JH, et al. The obligatory role of COX-2 expression for induction of HO-1 in ischemic preconditioned rat brain. Biochem Biophys Res Commun. 2008. 377:1191–1194.


22. Kwak KH. Emergence agitation/delirium: we still don't know. Korean J Anesthesiol. 2010. 59:73–74.


23. Dessy C, Kim I, Sougnez CL, Laporte R, Morgan KG. A role for MAP kinase in differentiated smooth muscle contraction evoked by alpha-adrenoceptor stimulation. Am J Physiol. 1998. 275:C1081–C1086.
24. Touyz RM, EI Mabrouk M, He G, Wu XH, Schiffrin EL. Mitogen-activated protein/extracellular signal-regulated kinase inhibition attenuates angiotensin II-mediated signaling and contraction in spontaneously hypertensive rat vascular smooth muscle cells. Circ Res. 1999. 84:505–515.


25. Watts SW, Yang P, Banes AK, Baez M. Activation of Erk mitogen-activated protein kinase proteins by vascular serotonin receptors. J Cardiovasc Pharmacol. 2001. 38:539–551.


26. Civantos Calzada B, Aleixandre de Artiñano A. Alpha-adrenoceptor subtypes. Pharmacol Res. 2001. 44:195–208.


27. Yildiz O, Ulusoy HB, Seyrek M, Gul H, Yildirim V. Dexmedetomidine produces dual alpha 2-adrenergic agonist and alpha 1-adrenergic antagonist actions on human isolated internal mammary artery. J Cardiothorac Vasc Anesth. 2007. 21:696–700.


28. Bain J, Plater L, Elliott M, Shpiro N, Hastie CJ, McLauchlan H, et al. The selectivity of protein kinase inhibitors: a further update. Biochem J. 2007. 408:297–315.


29. Molin JC, Bendhack LM. Clonidine induces rat aorta relaxation by nitric oxide-dependent and -independent mechanisms. Vascul Pharmacol. 2004. 42:1–6.


30. Hamasaki J, Tsuneyoshi I, Katai R, Hidaka T, Boyle WA, Kanmura Y. Dual alpha (2)-adrenergic agonist and alpha (1)-adrenergic antagonist actions of dexmedetomidine on human isolated endothelium-denuded gastroepiploic arteries. Anesth Analg. 2002. 94:1434–1440.


31. Motulsky HJ, Snavely MD, Hughes RJ, Insel PA. Interaction of verapamil and other calcium channel blockers with alpha 1- and alpha 2-adrenergic receptors. Circ Res. 1983. 52:226–231.


32. Kim HJ, Sohn JT, Jeong YS, Cho MS, Kim HJ, Chang KC, et al. Direct effect of dexmedetomidine on rat isolated aorta involves endothelial nitric oxide synthesis and activation of the lipoxygenase pathway. Clin Exp Pharmacol Physiol. 2009. 36:406–412.


33. Vanhoutte PM. Calcium-entry blockers, vascular smooth muscle and systemic hypertension. Am J Cardiol. 1985. 55:17B–23B.


34. Cussac D, Schaak S, Denis C, Paris H. alpha 2B-adrenergic receptor activates MAPK via a pathway involving arachidonic acid metabolism, matrix metalloproteinases, and epidermal growth factor receptor transactivation. J Biol Chem. 2002. 277:19882–19888.


35. Shah S, Sangari T, Qasim M, Martin T. Severe hypertension and bradycardia after dexmedetomidine for radiology sedation in a patient with acute transverse myelitis. Paediatr Anaesth. 2008. 18:681–682.


36. Zub D, Berkenbosch JW, Tobias JD. Preliminary experience with oral dexmedetomidine for procedural and anesthetic premedication. Paediatr Anaesth. 2005. 15:932–938.


37. Sakurai Y, Obata T, Odaka A, Terui K, Tamura M, Miyao H. Buccal administration of dexmedetomidine as a preanesthetic in children. J Anesth. 2010. 24:49–53.

