Abstract
Purpose
The phosphodiesterase 5 inhibitor sildenafil has antinociceptive effects, mediated by an increase in cGMP. This study examined the role of spinal adenosine and serotonin receptors played in the antinociceptive effects of intrathecal sildenafil.
Materials and Methods
Intrathecal catheters were inserted into the subarachnoid space of Sprague-Dawley male rats as a drug delivery device. Pain was induced by injecting formalin into the plantar surface of rats and observing nociceptive behavior (flinching response) for 60 mininutes. Then, the effects of intrathecal adenosine and serotonin receptor antagonists on the antinociceptive activity of intrathecal sildenafil were examined.
Sildenafil (Viagra®; Pfizer US Pharmaceutical Group, New York, NY, USA) is a selective, potent inhibitor of cGMP-specific phosphodiesterase 5, which increases the cGMP concentration by inhibiting the hydrolysis of cGMP to GMP.1 It has been shown to have antinociceptive effects in the formalin test in rats and in the writhing response induced by acetic acid and zymosan in mice,2-8 which is mediated through the nitric oxide (NO)-cGMP-potassium channel pathway.4,6,8,9 Recently, intrathecal sildenafil was reported to produce antinociceptive effects, mediated through GABAB and opioid receptors.5,10
Experimental data indicate that adenosine and serotonin act by modulating nociceptive transmission at the spinal level, mediated through the respective receptors.11-17 Recently, it was reported that sildenafil inhibited the anticonvulsant effect of adenosine.18 Additionally, dipyridamole, another type of phosphodiesterase 5 inhibitor, inhibited vasoconstriction induced by serotonin.19 These observations suggest that the effects of the cGMP-specific phosphodiesterase inhibitor are affected by both the adenosine and serotonin systems. However, the roles of spinal adenosine and serotonin receptors in the antinociceptive effect of intrathecal sildenafil remain to be determined.
Thus, this study investigated the effects of intrathecal sildenafil in a formalin-induced nociception model of rat and then determined the roles of adenosine and serotonin receptors played in the effects of sildenafil at the spinal level.
The Institutional Animal Care Committee, Research Institute of Medical Science of Chonnam National University, approved these experimental procedures.
Sprague-Dawley adult male rats (8-weeks-old, weighing 250-300 g) were used in these experiments. The rats were housed in a vivarium maintained at 20-23℃ with 12-hour light/dark cycle and were given food and water ad libitum.
For spinal drug administration, a polyethylene-10 tube was catheterized and inserted into the subarachnoid space under sevoflurane anesthesia and aseptic surgical conditions, as described previously.20 Following catheter implantation, rats were individually housed. Those rats with post-surgical neurological deficits were excluded from the study and sacrificed immediately with an overdose of volatile anesthetic. All testing was performed 5 days after intrathecal catheterization.
The rats were divided into the six following groups:
group 1: 20% dimethylsulfoxide (DMSO, control); n = 7.
group 2: sildenafil 3, 10, and 30 µg, respectively; n = 7 each.
group 3: CGS 15943 0.03 µg (adenosine-receptor antagonist); n = 6.
group 4: dihydroergocristine methanesulfonate 3 µg (serotonin-receptor antagonist); n = 6.
group 5: sildenafil 30 µg with CGS 15943 0.03 µg; n = 6.
group 6: sildenafil 30 µg with dihydroergocristine methanesulfonate 3 µg; n = 6.
The following drugs were used in this study: sildenafil, CGS 15943 (Sigma Aldrich, St. Louis, MO, USA), and dihydroergocristine methanesulfonate (Sigma). The sildenafil was provided by Pfizer Korea. All drugs were dissolved in 20% DMSO. Intrathecal administration of these agents was performed using a hand-driven, gear-operated syringe pump. All drugs were delivered in a 10-µL volume, followed by an additional 10 µL of normal saline to flush the catheter.
The formalin test was used as a nociceptive test.5 The formalin test was used as a nociceptive test.5 The plantar surface of the hind paw was injected with 50 µL of 5% formalin solution subcutaneously using a 30-gauge needle. Formalin injection produces characteristic pain behavior, that is, a rapid, brief flexion of the injected paw, which was defined as flinching. The total number of flinches was recorded over 5 min intervals. Rats were observed for a total period of 60 min. Formalin-induced flinches were observed in a characteristic biphasic response. The initial phase 1 (0-9 min) was followed by a relatively short quiescent period, which was then followed by a late phase 2 (10-60 min). Immediately following the completion of the formalin test, the rats were sacrificed by a volatile anesthetic overdose.
Four to five days after intrathecal catheterization, the rats were placed in a restraining cylinder for the experiments. After a 20-min habituation period, the rats were then randomly assigned to one of the drug treatment groups. Experiments were carried out in a blinded fashion. Intrathecal drugs were injected 10 min before formalin injection. The control consisted of intrathecal DMSO. The rats were used only once for the formalin test.
To investigate the antinociceptive effects of intrathecal sildenafil (3, 10, 30 µg), the flinching response was examined during phases 1 and 2 in the formalin test for 60 min.
Next, rats were pretreated with adenosine and serotonin receptor antagonists to determine which receptor affected sildenafil activity. These antagonists were administered intrathecally 10 min before delivering the intrathecal sildenafil (30 µg), and the formalin was injected 10 min later. The doses of the adenosine and serotonin receptor antagonists were based on pilot experiments and a previous study,21 as the maximum dosage that did not affect the control formalin response. The receptor antagonists used were the adenosine receptor antagonist CGS 15943 (0.03 µg) and the serotonin receptor antagonist dihydroergocristine methanesulfonate (3 µg).
Animals were tested only once. The researcher who tested the drugs was blinded to the drug given to each animal.
In order to evaluate the behavioral changes of sildenafil, CGS 15943, and dihydroergocristine methanesulfonate, additional rats received the highest doses of agents used, and were examined after intrathecal administration. Motor function was assessed using the placing-stepping reflex and the righting reflex.22
The former was evoked by drawing the dorsum of hind paw across the edge of the table. Normally, rats try to put their paws forward into a position for walking. The latter was evaluated by placing the rat horizontally with its back on the table; healthy rats produce immediate, coordinated twisting of the body into an upright position. The pinna and corneal reflexes were evoked by stimulating the ear canal or cornea with string, respectively.22 The healthy rats spontaneously shook their heads or blinked. Normality of behavior was judged as present or absent.
Data are expressed as means ± SEM. The time-response data are presented as the number of flinches. The dose-response data are presented as the total sum of flinches in each phase. The dose-response data for sildenafil were analyzed using the Jonckheere test. Comparison of antagonism of the effect of sildenafil was carried out using the unpaired t-test. p values < 0.05 were deemed to indicate statistical significance.
A subcutaneous injection of formalin into the hindpaw resulted in a biphasic flinching response by the injected paw. Fig. 1 shows the time course of intrathecal sildenafil, administered 10 min before the formalin injection. In group 2, which received sildenafil, the flinching response during phases 1 and 2 in the formalin test was significantly lower than group 1 (Fig. 1). Intrathecal sildenafil produced dose-dependent suppression of the flinching response during phases 1 and 2 in the formalin test (Fig. 2).
In both groups 3 and 4, intrathecal CGS 15943 and dihydroergocristine per se had little or no effect on the formalin-induced flinching response, compared with group 1 (Fig. 3).
In both groups 5 and 6, the flinching response was significantly higher, compared with group 2, indicating that intrathecal CGS 15943 and dihydroergocristine reversed the antinociceptive effect of sildenafil during phases 1 and 2 in the formalin test (Fig. 3).
In the formalin test, the characteristic biphasic pain behavior observed following formalin injection reflects a distinct phasic response which corresponds to essentially different processes. The phase 1 response is believed to represent a direct effect of formalin on sensory C fibers of primary afferent, thus the phase 1 of the formalin test reflects acute pain. On the other hand, the phase 2 response appears to be prominent and represents an intensified pain state in spite of a reduced level of afferent input, thus the phase 2 of the formalin test reflects a facilitated state.
In this study, intrathecal administration of sildenafil, a specific phosphodiesterase 5 inhibitor, dose-dependently suppressed flinching during both phase 1 and phase 2 of the formalin test, consistent with previous observations,4,5,9 suggesting that sildenafil is active against acute pain and facilitated state at the spinal level.
Phosphodiesterases occur widely in biological systems,23 and are involved in the hydrolysis of cGMP. Eleven subfamilies of phosphodiesterase isoenzymes have been identified, based on their functional characteristics.24 It has been reported that phosphodiestrase 5, 6 and 9 are specific for cGMP hydorolysis and, in particular, phosphodiesterase 5 seem to be the most relevant enzyme.25 Guanylyl cyclase catalyzes the formation of cGMP from guanosine triphosphate (GTP), leading to the synthesis of cGMP, whereas cGMP-specific phosphodiesterase catalyzes the hydrolysis of cGMP to GMP.25 Thus, the intracellular cGMP concentration is regulated by the action of guanylyl cyclase and the rate of degradation by cGMP-specific phosphodiesterase.25,26
It has been proposed that cGMP may play a critical role in the antinociceptive mechanism. Locally injected dibutyryl-cGMP showed antinociception in inflammatory hyperalgesia model.27 Intrathecally administered 8-bromo-cGMP reduced the mechanical allodynia in a neuropathic model.28,29 Thus, our observations suggest that intrathecal sildenafil produces an antinociceptive effect via the accumulation of cGMP, through the inhibition of phosphodiesterase 5.
Adenosine is an endogenous substance in the central nervous system (CNS), where it is an important modulator of neurotransmission and has been implicated in many physiological functions, such as the regulation of arousal and sleep, anxiety, cognition, and memory.11,12 Adenosine may play an important role in the modulation of nociceptive inputs through adenosine A1 and adenosine A2 receptors, which have been identified in the dorsal horn of the spinal cord.30 On the other hand, the adenosine A3 receptor has not yet been identified. Recently, however, it was suggested that the adenosine A3 receptor plays an important role in the control of the facilitated state at the spinal level.14
In addition to the above, the neurotransmitter serotonin [5-hydroxytryptamine, (5-HT)] plays an important role in the modulation of nociceptive transmission, and the major site of action of 5-HT is the spinal cord.13 Although multiple 5-HT receptor subtypes have been defined in the CNS,31 at least seven types of 5-HT receptor (including 5-HT1, 5-HT2, 5HT3, and 5-HT4) and several subtypes have so far been identified in the spinal cord.15,17
In this study, the antinociceptive effect of intrathecal sildenafil was found to be reversed by intrathecal non-specific adenosine and serotonin receptor antagonists. These findings suggest that the increased cGMP with sildenafil activates adenosine and serotonin receptors at the spinal level. However, the antinociceptive effect of intrathecal sildenafil was not completely reversed by the intrathecal adenosine and serotonin receptor antagonists. These results suggest that other mechanisms may also contribute to the antinociceptive effect of sildenafil at the spinal level. Previous work indicates that the NO-cGMP-PKG-potassium channel pathway, GABAB receptor, and opioid receptors are involved in sildenafil antinociception in the formalin model.4-6,8-10
In summary, sildenafil, an inhibitor of phosphodiesterase 5, increased the cGMP level in the spinal cord, thereby resulting in its antinociceptive effect in the formalin test. Both adenosine and serotonin receptors may be involved in the antinociceptive effects of sildenafil at the spinal level. However, it is not known what effects the increased cGMP, caused by sildenafil, exert on which subtypes of adenosine and serotonin receptors. Further research including a receptor-binding study will be necessary.
Figures and Tables
Fig. 1
Time-effect curve of sildenafil on flinching in the formalin test. Sildenafil was administered intrathecally at time - 10 min and the formalin was injected subcutaneously at time 0. Data are presented as the number of flinches. Each line represents mean ± SEM.
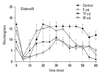
Fig. 2
Dose-response curve of intrathecal sildenafil on the flinching during phases 1 (A) and 2 (B) in the formalin test. Data are presented as the sum of the number of flinches in each phase. Intrathecal sildenafil produced dose-dependent suppression of flinching in both phases. Each line represents mean ± SEM. Compared with the control [dimethylsulfoxid (DMSO)]. *p < 0.05.
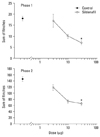
Fig. 3
The antagonistic effects of intrathecal CGS 15943 (adenosine receptor antagonist, 0.03 µg) and dihydroergocristine (HEC, serotonin receptor antagonist, 3 µg) on the antinociceptive effects of intrathecal sildenafil (30 µg) during phases 1 (A) and 2 (B) in the formalin test. The two antagonists and sildenafil were given 20 or 10 min respectively before injecting formalin. The data are presented as the sum of the number of flinches in each phase. Each bar represents mean ± SEM. Compared with sildenafil (30 µg): *p < 0.05, †p < 0.01.
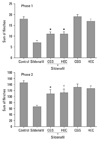
References
1. Gibson A. Phosphodiesterase 5 inhibitors and nitrergic transmission-from zaprinast to sildenafil. Eur J Pharmacol. 2001. 411:1–10.


2. Mixcoatl-Zecuatl T, Aguirre-Bañuelos P, Granados-Soto V. Sildenafil produces antinociception and increases morphine antinociception in the formalin test. Eur J Pharmacol. 2000. 400:81–87.


3. Asomoza-Espinosa R, Alonso-López R, Mixcoatl-Zecuatl T, Aguirre-Bañuelos P, Torres-López JE, Granados-Soto V. Sildenafil increases diclofenac antinociception in the formalin test. Eur J Pharmacol. 2001. 418:195–200.


4. Araiza-Saldaña CI, Reyes-García G, Bermúdez-Ocaña DY, Pérez-Severiano F, Granados-Soto V. Effect of diabetes on the mechanisms of intrathecal antinociception of sildenafil in rats. Eur J Pharmacol. 2005. 527:60–70.
5. Yoon MH, Kim WM, Lee HG, Kim YO, Huang LJ, An TH. Roles of opioid receptor subtypes on the antinociceptive effect of intrathecal sildenafil in the formalin test of rats. Neurosci Lett. 2008. 441:125–128.


6. Jain NK, Patil CS, Singh A, Kulkarni SK. Sildenafil-induced peripheral analgesia and activation of the nitric oxide-cyclic GMP pathway. Brain Res. 2001. 909:170–178.


7. Jain NK, Patil CS, Singh A, Kulkarni SK. Sildenafil, a phosphodiesterase-5 inhibitor, enhances the antinociceptive effect of morphine. Pharmacology. 2003. 67:150–156.


8. Vale ML, Rolim DE, Cavalcante IF, Ribeiro RA, Souza MH. Role of NO/cGMP/KATP pathway in antinociceptive effect of sildenafil in zymosan writhing response in mice. Inflamm Res. 2007. 56:83–88.


9. Patil CS, Singh VP, Kulkarni SK. Peripheral and central activation of nitric oxide-cyclic GMP pathway by sildenafil. Inflammopharmacology. 2005. 13:467–478.


10. Kim WM, Yoon MH, Lee HG, Han YG, Kim YO, Huang LJ, et al. GABAB receptor modulation on the antinociception of intrathecal sildenafil in the rat formalin test. Korean J Pain. 2007. 20:106–110.
11. Sawynok J, Liu XJ. Adenosine in the spinal cord and periphery: release and regulation of pain. Prog Neurobiol. 2003. 69:313–340.


12. Jacobson KA, Gao ZG. Adenosine receptors as therapeutic targets. Nat Rev Drug Discov. 2006. 5:247–264.


13. Fürst S. Transmitters involved in antinociception in the spinal cord. Brain Res Bull. 1999. 48:129–141.


14. Yoon MH, Bae HB, Choi JI. Antinociception of intrathecal adenosine receptor subtype agonists in rat formalin test. Anesth Analg. 2005. 101:1417–1421.


15. Jeong CY, Choi JI, Yoon MH. Roles of serotonin receptor subtypes for the antinociception of 5-HT in the spinal cord of rats. Eur J Pharmacol. 2004. 502:205–211.


16. Zahn PK, Straub H, Wenk M, Pogatzki-Zahn EM. Adenosine A1 but not A2a receptor agonist reduces hyperalgesia caused by a surgical incision in rats: a pertussis toxin-sensitive G protein-dependent process. Anesthesiology. 2007. 107:797–806.


17. Qu CL, Huo FQ, Huang FS, Li YQ, Tang JS, Jia H. The role of 5-HT receptor subtypes in the ventrolateral orbital cortex of 5-HT-induced antinociception in the rat. Neuroscience. 2008. 152:487–494.
18. Akula KK, Dhir A, Kulkarni SK. Nitric oxide signaling pathway in the anti-convulsant effect of adenosine against pentylenetetrazol-induced seizure threshold in mice. Eur J Pharmacol. 2008. 587:129–134.


19. Santos-Silva AJ, Cairrão E, Morgado M, Alvarez E, Verde I. PDE4 and PDE5 regulate cyclic nucleotides relaxing effects in human umbilical arteries. Eur J Pharmacol. 2008. 582:102–109.


20. Yaksh TL, Rudy TA. Chronic catheterization of the spinal subarachnoid space. Physiol Behav. 1976. 17:1031–1036.


21. Yoon MH, Choi JI, Jeong SW. Spinal gabapentin and antinociception: mechanisms of action. J Korean Med Sci. 2003. 18:255–261.
22. Yoon MH, Choi JI, Jeong SW. Antinociception of intrathecal cholinesterase inhibitors and cholinergic receptors in rats. Acta Anaesthesiol Scand. 2003. 47:1079–1084.


23. Beavo JA, Reifsnyder DH. Primary sequence of cyclic nucleotide phosphodiesterase isozymes and the design of selective inhibitors. Trends Pharmacol Sci. 1990. 11:150–155.
24. Uckert S, Hedlund P, Andersson KE, Truss MC, Jonas U, Stief CG. Update on phosphodiesterase (PDE) isoenzymes as pharmacologic targets in urology: present and future. Eur Urol. 2006. 50:1194–1207.
25. Pyne NJ, Arshavsky V, Lochhead A. cGMP signal termination. Biochem Soc Trans. 1996. 24:1019–1022.


26. Beavo JA. Cyclic nucleotide phosphodiesterases: functional implications of multiple isoforms. Physiol Rev. 1995. 75:725–748.


27. Ferreira SH, Nakamura M. I - Prostaglandin hyperalgesia, a cAMP/Ca2+ dependent process. Prostaglandins. 1979. 18:179–190.


28. Sousa AM, Prado WA. The dual effect of a nitric oxide donor in nociception. Brain Res. 2001. 897:9–19.


29. Tegeder I, Schmidtko A, Niederberger E, Ruth P, Geisslinger G. Dual effects of spinally delivered 8-bromo-cyclic guanosine monophosphate (8-bromo-cGMP) in formalin-induced nociception in rats. Neurosci Lett. 2002. 332:146–150.


30. Choca JI, Green RD, Proudfit HK. Adenosine A1 and A2 receptors of the substantia gelatinosa are located predominantly on intrinsic neurons: an autoradiography study. J Pharmacol Exp Ther. 1988. 247:757–764.