Abstract
Purpose
The purpose of this study is to investigate the mechanism of cellular proliferation of electromagnetic field (EMF) on human intervertebral disc (IVD) cells.
Materials and Methods
Human IVD cells were cultured three-dimensionally in alginate beads. EMF was exposed to IVD cells with 650Ω, 1.8 millitesla magnetic flux density, 60 Hz sinusoidal wave. Cultures were divided into a control and EMF group. Cytotoxicity, DNA synthesis and proteoglycan synthesis were measured by MTT assay, [3H]-thymidine, and [35S]-sulfate incorporation. To detect phenotypical expression, reverse transcription-polymerase chain reactions (RT-PCR) were performed for aggrecan, collagen type I, and type II mRNA expression. To assess action mechanism of EMF, IVD cells were exposed to EMF with NG-Monomethyl-L-arginine (NMMA) and acetylsalicylic acid (ASA).
Results
There was no cytotoxicity in IVD cells with the EMF group in MTT assay. Cellular proliferation was observed in the EMF group (p < 0.05). There was no difference in newly synthesized proteoglycan normalized by DNA synthesis between the EMF group and the control. Cultures with EMF showed no significant change in the expression of aggrecan, type I, and type II collagen mRNA compared to the control group. Cultures with NMMA (blocker of nitric oxide) or ASA (blocker of prostaglandin E2) exposed to EMF demonstrated decreased DNA synthesis compared to control cultures without NMMA or ASA (p < 0.05).
Conclusion
EMF stimulated DNA synthesis in human IVD cells while no significant effect on proteoglycan synthesis and chondrogenic phenotype expressions. DNA synthesis was partially mediated by nitric oxide and prostaglandin E2. EMF can be utilized to stimulate proliferation of IVD cells, which may provide efficient cell amplification in cell therapy to degenerative disc disease.
Pulsed electromagnetic fields (EMF) are widely used to facilitate fracture healing of the osteotomy site, un-united fractures, and spinal fusion in humans.1-4 It has been shown that EMF have a significant effect on bone formation and fracture healing through stimulating osteoblast proliferation and mineralization,5 endothelial cell proliferation, and capillary formation.6 Those proliferative and synthetic effects of EMF were mediated by complex molecular mechanisms, i.e., insulin receptor substrate-1 (IRS-1), the S6 ribosomal subunit kinase, the endothelial nitric oxide synthase (eNOS),7 c-myc and c-fos gene expression,8 and an insulin-like growth factor and its receptor.9,10 Furthermore, EMF enhances chondrogenesis and endochondral ossification, which appears to be dependent on stimulation of the progenitor cell pool.11
Intervertebral disc (IVD) is a fibrocartilagenous structure connecting the upper and lower vertebral body, providing mobility and stability of vertebral column.12 Degeneration of IVD caused by various genetic and environmental factors renders significant health care problems and increases socioeconomic cost.13 IVD is composed of the outer annulus fibrosus and inner nucleus pulposus. Cells from the nucleus pulposus exhibit chondrogenic phenotype expressing aggrecan type I collagen,14 with a less mitogenic effect under the influence of a growth factor compared to those from annulus fibrosus.15,16 Various therapeutic approaches have been tried to regenerate IVD and/or prevent degeneration of IVD, including cell therapy, gene therapy, and tissue engineering.17-20
Despite numerous studies of EMF application to fracture healing, spinal fusion, and cartilage healing, the effect of EMF on IVD has not yet been investigated. Furthermore, the biologic effect of EMF on IVD cells in terms of cytotoxicity, cellular proliferation, and matrix synthesis chondrogenic phenotype expression still remains unexplored.
Accordingly, in this experimental study, firstly, we applied lower energy, and pulsed sinusoidal EMF on human IVD cells, and analyzed the biologic effects, i.e. cellular proliferation, matrix synthesis, and phenotypical expression. Secondly, we investigated the possible role of nitric oxide (NO) and prostaglandin E2 (PGE2) in biologic effect of EMF to human IVD cells.
All of the experimental protocols were approved by the human subjects Institutional Review Board of our institution.
Lumbar IVD tissues were obtained from eight patients (age range: 37 to 54 years) during surgical disc procedures, including discectomy alone and discectomy during posterior lumbar interbody fusion. Classification of the IVD of each patient as a grade of degeneration was performed based on magnetic resonance images of each disc, which is based on histological change according to IVD degeneration.21 Grade III and IV degenerations were included in this study to minimize the effect of degeneration grades on the expression of phenotype and matrix synthesis. An attempt was made by the operating surgeon (SHM, HML) to carefully obtain tissue from the central aspect of the disc to optimize harvest of only the nucleus pulposus and transitional zone. Herniated disc material was strictly excluded from the current study. Disc tissue specimens were washed with Hank's balanced salt solution (HBSS) (Gibco-BRL, Grand Island, NY, USA) to remove blood and bodily fluid contaminants, and were then transported in sterile HBSS to the laboratory, less than 20 minutes following surgical removal.
Any obvious granulation tissue, dense outer annulus, and cartilaginous endplate were carefully removed from the disc tissue specimens. Disc cells were then isolated from the inner annulus and nucleus pulposus as described before.22 Briefly, the dissected specimens were minced with a scalpel into pieces of approximately two cubic millimeters in volume. Disc tissues from the nucleus pulposus and inner annulus were digested for 60 minutes at 37℃ under gentle agitation in a medium composed of equal parts of Dulbecco's Modified Eagle Medium and Ham's F-12 medium (DMEM/F12, Gibco-BRL Carlsbad, CA, USA) containing 5% heat-inactivated fetal bovine serum (FBS) (Gibco-BRL) with 0.4% pronase (Sigma, St. Louis, MO, USA) and 0.004% deoxyribonuclease II type IV (DNase, Sigma, St. Louis, MO, USA). The tissue was then washed 2 times with DMEM/ F12 and digested overnight under the same conditions, except that the pronase was replaced with bacterial 0.025% collagenase type II (Worthington Biochemical Corp., Lakewood, NJ, USA). Cells were filtered through a sterile nylon mesh filter (pore size: 70 um), counted in a haemocytometer, and plated in 24 well plates (Falcon, Franklin Lakes, NJ, USA) at a density of approximately 6×104 cells/mL. Primary cultures were sustained for 2 to 3 weeks in DMEM/F12 containing 10% FBS, 1% v/v penicillin, streptomycin, and nystatin (all antibiotics from Gibco-BRL) in a 5% CO2 incubator with humidity. The culture medium was changed twice a week. Cell viability was determined by the trypan blue exclusion test. Secondary cultures after trypsinization of primary cultures were exclusively utilized to minimize the effect of subculture on the expression of phenotype.
The preparation of IVD cells in alginate beads was performed as described elsewhere.23 Briefly, isolated cells from the primary culture with trypsinization were resuspended in sterile 0.15M NaCl containing 1.2% low-viscosity alginate (Sigma, St. Louis, MO, USA) at a density of two million cells per milliliter, then slowly expressed through a 22 gauge needle in a drop-wise fashion into 102 mM CaCl2 solutions. After gelation, the beads were allowed to polymerize further for a period of 10 minutes in the CaCl2 solution. After a wash in 10 volumes of 0.15M NaCl and 3 washes in 10 volumes of DMEM/F12 medium, the beads were finally placed in a complete culture medium. Ten beads were cultured in each well of a 24-well plate. To remove the cells from the alginate bead, wells were rinsed twice with 0.15M NaCl with gentle pipetting into the well. The rinse solution was incubated for 1 minute and was aspirated off. Three times of the volume of alginate in the dissolving buffer (55 mM sodium citrate and 0.15M NaCl) was added to the wells and the plates were incubated at 37℃ for 10 minutes with shaking.
EMF is designed similarly as reported previously.24 Tightly winded with copper lining, the cylinder of the polyethylene tube with a diameter of 12 cm and a height of 30 cm was connected to an electric power supply. The cultured cell was placed in the central position in the cylinder shaped tool. The copper lining was designed for resistance of 650 ohme and the output of 1.8 militelsa (mT) magnetic flux density-measuring by Walker mode MG2A Gaussmeter, when the source of electrical power was connected. The wave generation was designed to generate the sinusoidal wave of 30 Hz and connected with the electric power supply that was designed for generating the sinusoidal of 30 Hz in the EMF of 1.8 mT. Cultures were divided into a control and EMF group with or without Monomethyl-L-arginine (NMMA)(300 Um) and acetylsalicylic acid (ASA)(200 ug/mL). EMF exposure to cultures was set for 72 hours.
To investigate the effect of EMF on human IVD cell cultures, IVD cells were subjected to EMF exposure for 72 hours and then cells were analyzed for proliferation, proteoglycan synthesis, mRNA expression of aggrecan, and type I, II collagen. To elucidate the mechanism of EMF action on IVD cell proliferation, IVD cells were treated with NMMA as an inhibitor of NO or ASA as an inhibitor of prostaglandin E2, and then exposed to EMF for 72 hours.
After incubation for 48 hours, the viability of LF (ligament flavum) cells in each well was assessed using the MTT assay. Assays were done in triplicate; 200 µL MTT reagent (2 mg/mL culture medium) was added to each well and incubated for three hours in 5% CO2 at 37℃ with humidity. The supernatant was discarded and replaced with DMSO to dissolve the formazan product, which was measured at 550 nm in a spectrophotometric plate reader.
Total cellular RNA was eluted by selective binding to a silica gel-based membrane using an RNeasy mini kit. Reverse transcription of RNA into cDNA was performed by incubating 1 µL of RNA in a reaction mixture containing 0.5 mg/mL cDNA reaction product and was used as the template to co-amplify β-actin, aggrecan, and collagen type I and II. PCR was performed using a DNA thermal cycler. The same reaction profile was used for all primer sets: an initial denaturation at 94℃ for 1 minute, followed by 25-40 cycles of 94℃ for 5 seconds, 47-50℃ for 5 seconds, and 72℃ for 30 seconds, and an additional 2 min extension step at 72℃ after the last cycle. Amplification reactions specific for the following cDNAs were performed: β-actin, aggrecan, collagen type I, and collagen type II. PCR products (5 µL) were analyzed by electrophoresis in 2% agarose gels, and detected by staining with ethidium bromide. The intensity of the products was quantified using the BioImage Visage 110 system (BioRad, Hercules, CA, USA).
DNA synthesis was measured by the [3H]-thymidine incorporation. 5 µCi/mL of [3H]-thymidine (Amersham Biosciences; 25 Ci/mmol specific activity) was added to the control and treated cultures for the 24 hours. The medium was then discarded and the cells were trypsinized with trypsin/EDTA. The trypsinized cells were filtered onto glass fiber filters (Whatman GF/C; Maidstone, England), and transferred to a scintillation vial. Filters were dried and counted in 3 mL of scintillation cocktail solution (Beckman Coulter Inc., Brea, CA, USA) in a Packard scintillation counter. The results of each experiment, expressed as cpm/well, are the means of three parallel cultures.
5 µCi/mL of [35S]-sulfate (Amersham Biosciences; 25 Ci/mmol specific activity) was added to control and treated cultures for the 24 hours. At the end of the culture the medium was collected and the beads were dissolved with 28 mM EDTA/0.15M NaCl. The cells were then placed in an extraction media (8M guanidine HCl solution, 5 mM sodium acetate (pH5.8), proteinase inhibitor) at 4℃ for 48 hours. Aliquots (200 µL) of the cell extracts were eluted on Sephadex G-25M in PD-10 columns under dissociative conditions. Fractions (1 mL) were collected in scintillation vial and mixed with 6 mL scintillation cocktail solution (Beckman Coulter Inc., Brea, CA, USA). Five fractions were collected per sample, and three meddle fractions were counted in a Packard liquid scintillation counter.
Human IVD cell cultures with 72 hour EMF exposure showed no cytotoxicity compared to the control, as measured by an MTT assay. Each culture demonstrated uniform increase in optical density in the MTT assay (data not shown), implying cellular proliferation without recognizable cytotoxicity.
Human IVD cell cultures in three dimensional alginate beads were exposed to EMF for 72 hours. Cellular proliferation as measured by 3H-thymidine incorporation significantly increased (53%) in the IVD cell culture with EMF, compared to cultures without EMF exposure (p < 0.05). In the EMF exposure group, human IVD cell culture with NMMA demonstrated a 27% decrease in DNA synthesis compared to cultures without NMMA (p < 0.05). Also in the EMF group, human IVD cell culture with ASA demonstrated a 30% decrease in DNA synthesis compared to cultures without ASA (p < 0.05) (Fig. 1).
Human IVD cell culture in three dimensional alginate beads were exposed to EMF for 72 hours. Newly synthesized proteoglycan as measured by [35S]-sulfate incorporation demonstrated no difference in new synthesized proteoglycan compared to control cultures. In the EMF exposure group, human IVD cell culture with NMMA demonstrated an 83% increase in newly proteoglycan synthesis compared to cultures without NMMA (p < 0.05). Also in the EMF group, human IVD cell culture with ASA demonstrated a 60% increase in newly proteoglycan synthesis compared to cultures without ASA (p < 0.05) (Fig. 2).
In densitometric assay of reverse transcription-polymerase chain reaction, human IVD cells culture in three dimensional alginate beads with 72 hours exposure of EMF revealed no significant changes in the expression of aggrecan, type I, and type II collagen mRNA expression (Fig. 3).
Biological reaction of human tissue to EMF is widely divided into two categories: First, in a hazardous way, focusing on a possibility of tumorogenesis by cellular proliferations; secondly, in beneficial way, applying EMF as proliferating cells and stimulating matrix synthesis for regenerating tissues. EMF induces cellular proliferation of articular cartilage cells and nasal cartilage cells and stimulates synthesis of extracellular matrix and GAG.25 Furthermore, EMF affects osseous tissue in proliferation, differentiation, and expression of osteogenic phenotypes. Hence, these results have a significant implication in effective fracture healing with EMF.2,4,5 Although there are numerous studies of EMF on various musculoskeletal tissue, there has been no such study for validating EMF effects on IVD cells in vitro. Therefore, this study systematically examined the biological effect of EMF on IVD cells, i.e., cytotoxicity, DNA synthesis, matrix synthesis, and expression of chondrogenic phenotypes. Moreover, the mechanism of the EMF effect on IVD was examined.
In this study, there was no recognizable cytotoxicity in IVD cells under the influence of EMF as evidenced by maintained and increased cellular proliferation in MTT assay. Significant cellular proliferation (53%), as measured by DNA synthesis, is noted with a 72-hour exposure of EMF compared to the control culture without EMF. In proteoglycan synthesis normalized by DNA synthesis, human IVD cells with 72-hour EMF exposure revealed a slight decrease in proteoglycan synthesis, compared to the control group without EMF exposure; however, such a finding was statistically not significant. A slight decrease in proteoglycan synthesis in IVD cells with EMF can be explained, in part, by the increased DNA synthesis in IVD cells with the exposure of EMF. Cellular proliferation might hinder matrix synthesis of IVD cells. In addition, we analyzed phenotypical expressions of aggrecan, collagen type 1, and collagen type 2 mRNA. The mRNA expression of aggrecan in the culture with 72-hour exposure of EMF showed no significant changes between the EMF group and the control. Thus, these results of proteoglycan synthesis, mRNA expression of aggrecan, and collagen type II denote that EMF appears to render significant cellular proliferation of IVD cells, without significant effect on IVD in terms of chondrogenic matrix synthesis and its phenotypical expressions.
As a second part of the study, human IVD cell cultures were treated with NMMA, an inhibitor of NO, ASA as an inhibitor of PGE2, and then exposed to EMF to validate the mechanism of EMF on IVD in terms of cellular proliferation. IVD cells with EMF and NMMA demonstrated a statistically significant 27% decrease in DNA synthesis (p < 0.05). IVD cells with EMF and ASA revealed a statistically significant 30% decrease in DNA synthesis. (p < 0.05) These findings denote cellular proliferation in IVD with EMF, in part, mediated by second messengers such as NO and PGE2. It has been reported that cellular proliferation and matrix synthesis are mediated by eNOS,7 which is compatible to the current study. In addition to NO action, we can suggest that PGE2 mediated cellular proliferation can be a novel mechanism of EMF on cells. IVD cells with EMF, NMMA, or ASA exhibited increased proteoglycan synthesis, which could be explained by the fact that inhibition of cellular proliferation by NMMA or ASA might provide a suitable condition for matrix synthesis.
The characteristics of IVD cells which were utilized in this experiment can be debated. To minimize the effect of degeneration and dedifferentiation of IVD cells during the culture period, primary IVD cell cultures from IVD of grade III and IV degeneration were exclusively utilized.
The important point of this study is that EMF was applied to the IVD cell culture and the biological effect, especially cellular proliferation, of EMF on the IVD cell culture was demonstrated. Furthermore, we validated NO and PGE2 mediated cellular proliferation in IVD cells with EMF. With these results, the authors suggest a possible implication of EMF in IVD tissue. Firstly, EMF could be utilized in stimulating cellular proliferation as an amplification process in autogenous disc cell therapy to regenerate disc tissue. In disc tissue engineering, initial cellular amplification is of prime importance since excessive harvesting of autogenous disc tissue seemed to be clinically infeasible. With the proliferative effect of EMF in IVD cell cultures, a relatively small amount of disc tissue might be sufficient for autogenous tissue engineering.
One possible side effect with using the electromagnetic field could be excessive proliferation of cells and forming a tumor. It was impossible to address any metaplastic change of IVD cells under the influence of EMF with such a short duration (72 hours). However, the authors thought that EMF might render no significant metaplastic change in IVD cells since EMF was applied in vitro and with such a short period of time.
In conclusion, EMF stimulates cellular proliferation in human IVD cells while no significant changes in proteoglycan synthesis and the expression of chondrogenic phenotypes. Cellular proliferation by EMF is, in part, mediated by NO and PGE2.
Figures and Tables
Fig. 1
Percent control of DNA synthesis measured by 3H-thymidine incorporation (CPM). Control: the cultures without EMF exposure; EMF: the cultures with EMF exposure for 72 hours. EMF+NMMA: the cultures with EMF (72 hours) and Monomethyl-L-arginine. EMF+ASA: the cultures with EMF (72 hours) and acetylsalicylic acid. *p < 0.05. CPM, counts per minute; EMF, electromagnetic field; NMMA, NG-Monomethyl-L-arginine; ASA, acetylsalicylic acid.
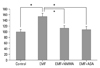
Fig. 2
Percent control of proteoglycan synthesis measured by 35S-Sulfate incorporation (CPM). Control: the cultures without EMF exposure; EMF: the cultures with EMF exposure for 72 hours. EMF+NMMA: the cultures with EMF (72 hours) and Monomethyl-L-arginine. EMF+ASA: the cultures with EMF (72 hours) and acetylsalicylic acid. *p < 0.05, CPM, counts per minute; EMF, electromagnetic field; NMMA, NG-Monomethyl-L-arginine; ASA, acetylsalicylic acid; NS, not-significant.
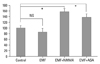
ACKNOWLEDGEMENTS
This research is supported, in part, by Brain Korea 21 Project for Medical Science and Yuhan CMB, Yonsei University College of Medicine.
References
1. Colson DJ, Browett JP, Fiddian NJ, Watson B. Treatment of delayed- and non-union of fractures using pulsed electromagnetic fields. J Biomed Eng. 1988. 10:301–304.


2. Foley KT, Mroz TE, Arnold PM, Chandler HC Jr, Dixon RA, Girasole GJ, et al. Randomized, prospective, and controlled clinical trial of pulsed electromagnetic field stimulation for cervical fusion. Spine J. 2008. 8:436–442.
3. Holmes GB Jr. Treatment of delayed unions and nonunions of the proximal fifth metatarsal with pulsed electromagnetic fields. Foot Ankle Int. 1994. 15:552–556.


4. Borsalino G, Bagnacani M, Bettati E, Fornaciari F, Rocchi R, Uluhogian S, et al. Electrical stimulation of human femoral intertrochanteric osteotomies. Double-blind study. Clin Orthop Relat Res. 1988. (237):256–263.
5. Lin HY, Lu KH. Repairing large bone fractures with low frequency electromagnetic fields. J Orthop Res. 2010. 28:265–270.
6. Yen-Patton GP, Patton WF, Beer DM, Jacobson BS. Endothelial cell response to pulsed electromagnetic fields: stimulation of growth rate and angiogenesis in vitro. J Cell Physiol. 1988. 134:37–46.


7. Schnoke M, Midura RJ. Pulsed electromagnetic fields rapidly modulate intracellular signaling events in osteoblastic cells: comparison to parathyroid hormone and insulin. J Orthop Res. 2007. 25:933–940.


8. De Mattei M, Gagliano N, Moscheni C, Dellavia C, Calastrini C, Pellati A, et al. Changes in polyamines, c-myc and c-fos gene expression in osteoblast-like cells exposed to pulsed electromagnetic fields. Bioelectromagnetics. 2005. 26:207–214.
9. Fitzsimmons RJ, Ryaby JT, Magee FP, Baylink DJ. IGF-II receptor number is increased in TE-85 osteosarcoma cells by combined magnetic fields. J Bone Miner Res. 1995. 10:812–819.


10. Fitzsimmons RJ, Baylink DJ. Growth factors and electromagnetic fields in bone. Clin Plast Surg. 1994. 21:401–406.


11. Frazer A, Bunning RA, Thavarajah M, Seid JM, Russell RG. Studies on type II collagen and aggrecan production in human articular chondrocytes in vitro and effects of transforming growth factor-beta and interleukin-1beta. Osteoarthritis Cartilage. 1994. 2:235–245.


12. Roberts S, Evans H, Trivedi J, Menage J. Histology and pathology of the human intervertebral disc. J Bone Joint Surg. 2006. 88:Suppl 2. 10–14.


13. Katz JN. Lumbar disc disorders and low-back pain: socioeconomic factors and consequences. J Bone Joint Surg. 2006. 88:Suppl 2. 21–24.


14. Hoogendoorn R, Doulabi BZ, Huang CL, Wuisman PI, Bank RA, Helder MN. Molecular changes in the degenerated goat intervertebral disc. Spine (Phila Pa 1976). 2008. 33:1714–1721.


15. Kim H, Lee JU, Moon SH, Kim HC, Kwon UH, Seol NH, et al. Zonal responsiveness of the human intervertebral disc to bone morphogenetic protein-2. Spine (Phila Pa 1976). 2009. 34:1834–1838.


16. Kim DJ, Moon SH, Kim H, Kwon UH, Park MS, Han KJ, et al. Bone morphogenetic protein-2 facilitates expression of chondrogenic, not osteogenic, phenotype of human intervertebral disc cells. Spine (Phila Pa 1976). 2003. 28:2679–2684.


17. Hiyama A, Mochida J, Iwashina T, Omi H, Watanabe T, Serigano K, et al. Transplantation of mesenchymal stem cells in a canine disc degeneration model. J Orthop Res. 2008. 26:589–600.
18. Moon SH, Nishida K, Gilbertson LG, Lee HM, Kim H, Hall RA, et al. Biologic response of human intervertebral disc cells to gene therapy cocktail. Spine (Phila Pa 1976). 2008. 33:1850–1855.


19. Nishida K, Kang JD, Gilbertson LG, Moon SH, Suh JK, Vogt MT, et al. Modulation of the biologic activity of the rabbit intervertebral disc by gene therapy: an in vivo study of adenovirus-mediated transfer of the human transforming growth factor beta 1 encoding gene. Spine (Phila Pa 1976). 1999. 24:2419–2425.
20. Yang X, Li X. Nucleus pulposus tissue engineering: a brief review. Eur Spine J. 2009. 18:1564–1572.
21. Thompson JP, Pearce RH, Schechter MT, Adams ME, Tsang IK, Bishop PB. Preliminary evaluation of a scheme for grading the gross morphology of the human intervertebral disc. Spine (Phila Pa 1976). 1990. 15:411–415.


22. Chelberg MK, Banks GM, Geiger DF, Oegema TR Jr. Identification of heterogeneous cell populations in normal human intervertebral disc. J Anat. 1995. 186:43–53.
23. Maldonado BA, Oegema TR Jr. Initial characterization of the metabolism of intervertebral disc cells encapsulated in microspheres. J Orthop Res. 1992. 10:677–690.