Abstract
Purpose
In cardiac 2-[F-18]fluoro-2-deoxy-D-glucose (FDG)-positron emission tomography (PET) examination, interpretation of myocardial viability in the low uptake region (LUR) has been difficult without additional perfusion imaging. We evaluated distribution patterns of FDG at the border zone of the LUR in the cardiac FDG-PET and established a novel parameter for diagnosing myocardial viability and for discriminating the LUR of normal variants.
Materials and Methods
Cardiac FDG-PET was performed in patients with a myocardial ischemic event (n = 22) and in healthy volunteers (n = 22). Whether the myocardium was not a viable myocardium (not-VM) or an ischemic but viable myocardium (isch-VM) was defined by an echocardiogram under a low dose of dobutamine infusion as the gold standard. FDG images were displayed as gray scaled-bull's eye mappings. FDG-plot profiles for LUR (= true ischemic region in the patients or normal variant region in healthy subjects) were calculated. Maximal values of FDG change at the LUR border zone (a steepness index; Smax scale/pixel) were compared among not-VM, isch-VM, and normal myocardium.
Results
Smax was significantly higher for n-VM compared to those with isch-VM or normal myocardium (ANOVA). A cut-off value of 0.30 in Smax demonstrated 100% sensitivity and 83% specificity for diagnosing n-VM and isch-VM. Smax less than 0.23 discriminated LUR in normal myocardium from the LUR in patients with both n-VM and isch-VM with a 94% sensitivity and a 93% specificity.
Cardiac 2-[F-18]fluoro-2-deoxy-D-glucose (FDG)-positron emission tomography (PET) has been regarded as the gold standard for interpreting myocardial viability,1,2 and it is an especially important tool for predicting improvements in cardiac function after revascularization therapies for patients with myocardial ischemic events.3 A sustained uptake of FDG accompanied by reduced perfusion (metabolism-perfusion mismatch) is considered to be an indicator of ischemic but viable myocardium. Low uptake of FDG suggests a decrease in glucose utilization due to ischemic insult and is considered to be an indicator of either viable or not viable myocardium depending on the severity the of defect in FDG uptake. For example, a myocardial FDG uptake of less than 50% of the normal uptake value is one of the criteria to indicate a not viable myocardium.4-6 The extent of lower uptake of FDG is qualitatively expressed as mild, moderate, or severe compared to background and other myocardial regions.7,8 However, it has been difficult to interpret whether the myocardium is viable or not viable without information from perfusion imaging.2 Another limitation of cardiac FDG-PET is the spatial and temporal heterogeneity of the uptake distributions in normal myocardium9 that are recognized as normal variants.10,11 Whether a low uptake region indicates a physiological modification or a pathological perturbation is one, it needs to be differentiated in order to avoid false-positive results. Therefore, it is necessary to establish a novel diagnostic parameter to interpret low uptake regions of cardiac FDG without additional perfusion imaging in order to discriminate the three different myocardial conditions: normal variant, ischemic but viable myocardium, and not viable myocardium.
In the present study, we hypothesized that distribution of the FDG at the border zone of a low uptake region demonstrated characteristics specific to the normal myocardium, ischemic but viable myocardium, and not viable myocardium conditions that can be used to discriminate one from another. To verify our hypothesis, we examined cardiac FDG-PET in healthy volunteers and in patients with myocardial ischemic event. In the latter, viability of the myocardium at the culprit lesion was defined by an echocardiogram at rest and under low dose-dobutamine stress. Patient population was further divided into two groups: those with ischemic but viable myocardium, and those with not viable myocardium. By converting FDG-PET data to a gray scale bull's eye mapping, we were able to evaluate a novel index expressing the steepness of FDG distribution at the border zone of the low uptake region (Smax). We report in the present study that cut-off values of Smax can be used to discriminate normal myocardium, ischemic but viable myocardium, and not viable myocardium with high sensitivity and high specificity.
The study population was comprised of 22 patients with myocardial ischemic events (patient group; range, 53-80 years, mean ± SD: 71.1 ± 7.5 years, 18 males, 4 females) and 22 healthy volunteers (healthy group: range, 19-22 years, mean ± SD: 20.5 ± 0.7 years, 10 males, 12 females). Patients with myocardial ischemic events who were hospitalized and had cardiac catheterization were also enrolled. The present study began more than one month after acute on-set of the ischemic event or the last revascularization therapy. In the healthy group, the inclusion criteria included normal physical examination, normal resting electrocardiogram, normal echocardiogram, and lack of a past history of cardiac disease or any chronic diseases. Detailed information of the patients and healthy volunteers are shown in Tables 1 and 2, respectively.
Both the control and the patient groups were further divided into two subgroups: those under fasting conditions and those under glucose loading conditions before FDG administration. In the former, the fasting condition was maintained 6 to 7 hours after meals (two rice balls and plum pickles and green tea). In the latter, 6 to 7 hours of the fasting was maintained followed by an oral administration of glucose (75 g) solution 60 minutes before a FDG injection.
This study was approved by the Research Ethics Committee of Kawasaki Medical School (No. 295). Each subject gave written consent before participating in the study.
18F-FDG of 185MBq (FDGscan In jectable, Nihon Mediphysics, Tokyo, Japan) was administered intravenously. At 115 minutes after FDG administration, a transmission scan was performed using a Discovery ST Elite (GE Healthcare, Piscataway, NJ, USA) under the following conditions: 5 minutes data acquisition, 128×128 matrix size, 3.3 mm slice width, 47 slices, and attenuation correction using CT.
Acquired FDG images were reconstructed using 3D-OSEM (VEU point) under the following conditions: 3.59 mm post filter, 3.59 mm FWHM, 28 subset, and 2 iterations. Short axis view of the heart was converted to DICOM data using Daemon Research Image Processor (version 1.0.3.4, Fuji Film Pharma, Co. Ltd., Tokyo, Japan) and displayed as colored bull's eye mapping using CardioBull (version 3.0, Fuji Film Pharma). The identical bull's eye mapping was redisplayed with 256-gray scale. Referring to the colored bull's eye mapping and patient database (electrocardiogram, echocardiogram, and cardiac catheterization), the location of the culprit region in the gray scale bull's eye mapping was confirmed. Including the culprit region (area of ischemic event) and neighboring non-culprit region, the plot profile (a gray scale-slope) along the optional line was scanned to find the point where the gray scale slope was the steepest (Scion Image, ALPHA4.0.3.2, Scion Corporation, Frederick, Maryland, USA). The steepness of the gray scale slope at the optional pixel point was analyzed by the change in gray scale per pixel. Maximal steepness was analyzed by the absolute value of change in gray scale per pixel (Smax; scale/pixel) which was manually detected since automatic detection algorithm has not developed yet. Almost of all of the maximal values (Smax point) were found around the line connecting the minimal FDG count (in the culprit lesion) and neighboring high FDG count in the bull's eye mapping. In the healthy group, the Smax was determined by the same procedure. The lowest FDG uptake region was included instead of an area of the ischemic event. This analysis was performed by two independent investigators.
Since the total amount of cardiac FDG uptake is known to be critical for interpreting the cardiac FDG-PET findings,12 FDG count of the left ventricle was evaluated by comparing it to the background. One ROI was placed to include the whole left ventricle and another ROI in the right lung field (as back ground) in the serial transaxial images (Daemon Research Image Processor, version 1.0.3.4, Fuji Film Pharma). Maximal count in the left ventricle divided by maximal count in the lung (Hmax/Lmax) was calculated. We assumed in the present study that sufficient cardiac uptake was defined by Hmax/Lmax ≥ 10.0, and insufficient if HHmax/Lmax < 10.0.
Whether the area demonstrating low uptake region in FDG-PET (area of interest) was not viable myocardium or ischemic but viable myocardium was defined by echocardiogram (SONOS 7500, Phillips, Amsterdam, The Netherlands) at rest and under low-dobutamine stress. If the area of interest showed normal or hypokinetic wall motion at rest, this region was defined as ischemic but viable. If the area of interest was akinetic or showed dyskinetic wall motion at rest, echocardiogram was evaluated under low dose (5 to 10 µg/kg/minute) dobutamine infusion. If improvement in the wall motion was observed, this region was defined as ischemic but viable myocardium.13 If not, then it was defined as not viable myocardium. The viability was diagnosed by agreement from two echo-cardiologists.
All data is expressed as the mean ± SD. The differences in values were evaluated by one way-ANOVA, followed by Fisher's PLSD. The sensitivity and specificity of the cut-off value of Smax was evaluated by qui-square test. The inter-observer agreement was assessed by determining the mean and SD of the between observation difference. A p value less than 0.05 was considered statistically significant.
Echocardiographic study defined ten patients with ischemic but viable myocardium and 12 patients with not viable myocardium. Twenty FDG sets of data were evaluated as normal myocardium in the healthy group. Two healthy volunteers (under fasting condition) were not included in the final analysis as they showed FDG uptake levels that were too low to reconstruct images. The same failure occurred in one patient with not viable myocardium. Thus, 11 sets of FDG data were evaluated as not viable myocardium. One patient with ischemic but viable myocardium showed extremely high FDG uptake (typical case of metabolism-perfusion mismatch). This case was also excluded because we only focused on evaluating the clinical significance of low FDG uptake in the present study. Thus, nine FDG patients were evaluated as ischemic but viable myocardium.
Representative images of bull's eye mapping of FDG-PET are shown in Fig. 1A. Both the patients with myocardial ischemic events as well as the healthy volunteers showed low uptake regions. Almost all of the healthy volunteers showed heterogeneous distribution. Single or multiple low uptake region(s) were observed in 89% of subjects under glucose loading and 92% of subjects under fasting (Table 2). Thus, the finding of low uptake region per se is not likely to be a diagnostic parameter.
Fig. 1B demonstrates the plot profile of the FDG distribution using the gray scale mapping in Fig. 1A. Steepness of the FDG-gray scale change (Fig. 1C) was higher in the ischemic patient group (absolute values of Smax are 0.58 and 0.53 scale/pixel) than that in the healthy volunteers (absolute values of Smax are 0.22 and 0.30 scale/pixel).
Hmax/Lmax, the parameter for global myocardial FDG uptake, is high (assumed sufficient in the present study) in patient #15 (15.4) and healthy volunteer #20 (15.0), but low in patient #20 (6.3) and in healthy volunteer #16 (8.0, Fig. 1D). Thus, the Smax is feasible for use in interpretations for patient #15 and healthy volunteer #20, but not for patient #20 and for healthy volunteer #16. A patient with ischemic but viable myocardium, patient #17, showed high enough Hmax/Lmax (18.1) and a low absolute value of Smax(0.19).
The Smax values from subjects with Hmax/Lmax ≥ 10 were selected and analyzed. The Smax was significantly different between three myocardial conditions (p < 0.0001, ANOVA) (Fig. 2). The Smax in the not viable myocardium (0.42 ± 0.10 scale/pixel) was significantly higher than that in the normal myocardium (0.19 ± 0.04 scale/pixel, p < 0.0001) as well as that in the ischemic but viable myocardium (0.25 ± 0.05 scale/pixel, p < 0.0001). The Smax in the ischemic but viable myocardium was higher than that in normal myocardium (p = 0.03). In the patient group, a cut-off value of 0.30 scale/pixel differentiated not viable myocardium from ischemic but viable myocardium with 100% sensitivity and 71% specificity. This indicated that 0.30 scale/pixel in the Smax is a useful parameter to estimate myocardial viability. Overall, a cut-off value of 0.23 scale/pixel differentiated the patient group (not viable myocardium + ischemic but viable myocardium) from the healthy group with 88% sensitivity and 93% specificity. This cut-off value was also useful in detecting patients with myocardial ischemic event (not viable myocardium or ischemic but viable myocardium) in an optional population. However, this sensitivity and specificity were slightly decreased to 85% and 85%, respectively, if the subjects' data with Hmax/Lmax less than 10 were included in the analysis.
Inter-observer difference was low for measurement of Smax (Fig. 3). The correlation coefficient was high for measurements by two different observers (r = 0.97).
Diagnosis of myocardial viability and discrimination of normal variants in FDG-PET examination have previously been considered difficult without additional information from perfusion imaging. The major reason is the spatial and temporal heterogeneity in FDG distribution along with the low uptake regions that are observed in patients with ischemic but viable myocardium and even in healthy subjects. In the present study, we established a novel and simple numerical parameter of cardiac FDG-PET to diagnose not viable myocardium as well as ischemic but viable myocardium, and also to discriminate normal variants. In the selected subjects with sufficient uptake of myocardial FDG (Hmax/Lmax ≥ 10), the index of maximal steepness of FDG uptake gray scale slope at the border zone of the low uptake region in the bull's eye mapping (Smax) was significantly different for these three conditions. The Smax in the not viable myocardium (defined by echocardiogram at rest and under low dose dobutamine stress) was distributed at a higher range (0.42 ± 0.10 scale/pixel). The Smax in the ischemic but viable myocardium distributed at an intermediate range (0.25 ± 0.05 scale/pixel). The Smax in the normal variant distributed at a lower range (0.19 ± 0.04 scale/pixel). In the patient group with myocardial ischemic events, the cut-off value of 0.30 scale/pixel differentiated not viable myocardium from ischemic but viable myocardium with 100% sensitivity and 71% specificity, indicating the usefulness of Smax to diagnose myocardial viability after the myocardial ischemic event. Overall, a cut-off value of 0.23 scale/pixel differentiated the patient group (≥ 0.23) from the normal group (< 0.23) with 88% sensitivity and 93% specificity, indicating the usefulness of Smax to detect patients with a myocardial ischemic event (myocardium is viable or not viable) within an optional population. We concluded that the Smax in the FDG-PET is a novel parameter to diagnose myocardial viability without additional information from perfusion imaging and also a tool to discriminate normal variants of FDG distribution.
We attributed the significant differences in Smax values among not viable myocardium, ischemic but viable myocardium, and normal variant populations to partial volume effect as limited by spatial resolution of the PET (voxel size of the scanner: 3.3×3.3×3.3 mm3 in the present study). Optional point in the bull's eye mapping (X-Y axes plane) is converted to display the mean FDG count along the transmural direction (Z-axis; ~10 mm depth). We considered that the real distribution of FDG along transmural wall is different for these three conditions, as shown in the schematic diagram in Fig. 4. After a myocardial ischemic event, a partition of glucose utilization increases, but eventually disappears depending on the severity of the ischemic insult. If the ischemic insult is severe, the not viable myocardium (lack of FDG uptake) occupies the wall transmurally with a relatively clear border. Thus, the Smax would be high in this case. If the ischemic insult is mild, the not viable myocardium is limited to the subendocardial layer. Also, small clusters of the ischemic area14 heterogeneously distributed in the transmural wall15 may merge together.16 The borderline may be relatively unclear, and thus the Smax would be lower than that in the not viable myocardium. Glucose utilization in these myocardial conditions is pathologically disrupted, and the presence of these conditions must lead to different glucose utilization in physiological modification in normal subjects (normal variant). Although the mechanisms of physiological modification of glucose utilization (its control unit size of and mechanism of spatial/temporal heterogeneity etc.) are still unclear,17 we believe that the appearance of a decreased glucose utilization along the transmural wall as a normal variant is different from that in pathological disruption of glucose utilization due to ischemic insults. This speculation may be supported by the lowest Smax value in the normal variant. Further investigation is required to confirm this hypothesis.
A perfusion image (i.e., 201Tl-Cl and 13N ammonia scintigram etc.) is compared to metabolic assessment by myocardial FDG-PET to interpret myocardial viability. American Society of Nuclear Cardiology Practice Guidelines in 2003 noted that interpretation of FDG images without perfusion imaging information is considered to be difficult and unreliable for distinguishing normal myocardium from irreversibly injured myocardium.2 However, the present study showed that the myocardial FDG-PET enabled us to distinguish these myocardial conditions without additional perfusion imaging by analyzing the Smax in FDG-PET. Using this method, we were able to diagnose not viable myocardium (≥ 0.30 scale/pixel) and ischemic but viable myocardium (< 0.30 scale/pixel) in the patient group and also to discriminate normal myocardium (normal variant, < 0.23 scale/pixel) from the patient group with high sensitivity and high specificity if there was sufficient global cardiac FDG uptake (Hmax/Lmax ≥ 10.0). We do not, however, believe that a perfusion image is unnecessary in evaluating myocardial viability. One case showed extremely high uptake of FDG at the ischemic area and was readily interpreted as viable myocardium with an additional 201Tl-Cl perfusion imaging (but this case was excluded from the present study because we primarily focused on the significance of Smax in cases with low uptake region of FDG).
Glucose loading of the subjects has been previously recommended18 to increase unitization of glucose by the myocardium and to improve diagnostic accuracy of cardiac FDG-PET.12 However, it was insufficient to erase the heterogeneities in FDG distribution in the present study as 89% of the healthy group under glucose loading had assumed heterogeneous distribution of FDG (Table 2). Nonetheless, glucose loading was effective in increasing global FDG uptake into the myocardium19 (increases in probability to satisfy the condition of Hmax/Lmax ≥ 10.0) and helped improve validity of Smax. Note that fasting conditions allowed interpretation of myocardial viability by Smax as long as the subject showed sufficient global myocardial FDG uptake (Hmax/Lmax ≥ 10.0, 46% of the healthy volunteer and 86% of the patients satisfy this criteria under fasting conditions in the present study). Data selection according to the criteria of Hmax/Lmax ≥ 10.0 assisted in evaluating Smax despite glycemic conditioning. Our recommended strategies to diagnose myocardial viability using FDG-PET and Smax, as well as discrimination of normal variant are shown in Fig. 5.
In terms of study limitations, the number of the patients with identical glucose conditioning is still small in the present study. A strict criteria of ischemic conditions may be further required. Additional perfusion imaging study may help the conclusion. A larger scale longitudinal study on whether Smax predicts the extent of functional recovery by revascularization therapy as well as the patient prognosis is required. To do this, an automatic program to calculate the Smax would be needed.
In conclusion, the Smax in cardiac FDG-PET bull's eye mapping is a simple and useful numerical parameter with high sensitivity and high specificity to diagnose myocardial viability (not viable myocardium if cut-off value ≥ 0.30 scale/pixel) and to discriminate normal variants (if cut-off value < 0.23 scale/pixel). Additional perfusion imaging is supportive, but not indispensable, if the Smax is evaluated under the condition of Hmax/Lmax ≥ 10. Glucose loading is recommended to obtain higher probability of Hmax/Lmax ≥ 10, but not necessary as long as the patients satisfy the criteria of Hmax/Lmax ≥ 10 when fasting.
Figures and Tables
Fig. 1
Representative images of cardiac FDG-PET and Smax in patients with myocardial ischemic event as well as healthy volunteers. Myocardium in patient #17 was defined as viable by an echocardiogram under low dose of dobutamine stress. Myocardium in patient #15 and #20 were defined as not viable. (A) FDG bull's eye mapping (colored and gray scaled). Low uptake regions were observed in both patients (#17, #15, and #20) and healthy volunteers (#20 and #16). (B) FDG plot profile along white and blue lines in the panel A. (C) Changing rate of FDG gray scale per pixel along the line. Absolute values (red) indicate Smax values. The pink dots in the panels A, B, and C indicate the location of Smax. (D) Hmax/Lmax values are shown. FDG, cardiac 2-[F-18] fluoro-2-deoxy-D-glucose; PET, positron emission tomegraphy.
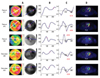
Fig. 2
Difference of Smax in three myocardial conditions. The Smax is the highest in the not viable myocardium (red circles), intermediate in ischemic but viable myocardium (brown circles), and the lowest in normal myocardium (blue circles). Closed circles and solid lines (mean ± SD): data from the subjects with Hmax/Lmax ≥10. Open circles: data of the subjects with Hmax/Lmax < 10. Dashed lines (mean ± SD) were analyzed by including all data (both Hmax/Lmax≥10 and <10).
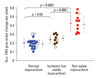
Fig. 4
Schematic diagram of real FDG uptake in the myocardial wall and FDG-PET count. Gray area: positive FDG uptake. White area: negative FDG uptake. Voxel size of the PET scanner in the present study was 3.3×3.3×3.3 mm3. FDG count is displayed as its average of that in the transmural wall. Steepness of the FDG slope at the border zone of the low uptake region is different for not viable myocardium, ischemic but viable myocardium, and normal variant (speculated). FDG, cardiac 2-[F-18] fluoro-2-deoxy-D-glucose; PET, positron emission tomegraphy.
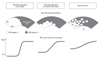
Table 1
Patient Profile and Findings of Echocardiogram and FDG-PET
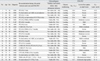
AK, akinetic wall motion; AMI, acute myocardial infarction; CABG, coronary arterial bypass graft; CTO, chronic total obstruction; DK, dyskinetic wall motion; HK, hypokinetic wall motion; Hmax / Lmax, heart / lung ratio; NA, not available for dubutaimine infusion; NL, normal wall motion; OMI, old myocardial infarction; PCI, percutaneous coronary intervention; POBA, percutaneous old balloon angioplasty; SH, severely hypokinetic wall motion; Smax, steepness index; SVG, saphenous vein graft; UAP, unstable angina.
#; segment number of coronary arterial branch.
References
1. Baumgartner H, Porenta G, Lau YK, Wutte M, Klaar U, Mehrabi M, et al. Assessment of myocardial viability by dobutamine echocardiography, positron emission tomography and thallium-201 SPECT: correlation with histopathology in explanted hearts. J Am Coll Cardiol. 1998. 32:1701–1708.


2. Schelbert HR, Beanlands R, Bengel F, Knuuti J, Dicarli M, Machac J, et al. PET myocardial perfusion and glucose metabolism imaging: Part 2-Guidelines for interpretation and reporting. J Nucl Cardiol. 2003. 10:557–571.


3. Bax JJ, Fath-Ordoubadi F, Boersma E, Wijns W, Camici PG. Accuracy of PET in predicting functional recovery after revascularisation in patients with chronic ischaemic dysfunction: head-to-head comparison between blood flow, glucose utilisation and water-perfusable tissue fraction. Eur J Nucl Med Mol Imaging. 2002. 29:721–727.


4. Knuuti MJ, Nuutila P, Ruotsalainen U, Teräs M, Saraste M, Härkönen R, et al. The value of quantitative analysis of glucose utilization in detection of myocardial viability by PET. J Nucl Med. 1993. 34:2068–2075.
5. Baer FM, Voth E, Deutsch HJ, Schneider CA, Horst M, de Vivie ER, et al. Predictive value of low dose dobutamine transesophageal echocardiography and fluorine-18 fluorodeoxyglucose positron emission tomography for recovery of regional left ventricular function after successful revascularization. J Am Coll Cardiol. 1996. 28:60–69.


6. Altehoefer C, Kaiser HJ, Dörr R, Feinendegen C, Beilin I, Uebis R, et al. Fluorine-18 deoxyglucose PET for assessment of viable myocardium in perfusion defects in 99mTc-MIBI SPET: a comparative study in patients with coronary artery disease. Eur J Nucl Med. 1992. 19:334–342.


7. Porenta G, Kuhle W, Czernin J, Ratib O, Brunken RC, Phelps ME, et al. Semiquantitative assessment of myocardial blood flow and viability using polar map displays of cardiac PET images. J Nucl Med. 1992. 33:1628–1636.
8. Nekolla SG, Miethaner C, Nguyen N, Ziegler SI, Schwaiger M. Reproducibility of polar map generation and assessment of defect severity and extent assessment in myocardial perfusion imaging using positron emission tomography. Eur J Nucl Med. 1998. 25:1313–1321.
9. Inglese E, Leva L, Matheoud R, Sacchetti G, Secco C, Gandolfo P, et al. Spatial and temporal heterogeneity of regional myocardial uptake in patients without heart disease under fasting conditions on repeated whole-body 18F-FDG PET/CT. J Nucl Med. 2007. 48:1662–1669.


10. Choi Y, Brunken RC, Hawkins RA, Huang SC, Buxton DB, Hoh CK, et al. Factors affecting myocardial 2-[F-18]fluoro-2-deoxy-D-glucose uptake in positron emission tomography studies of normal humans. Eur J Nucl Med. 1993. 20:308–318.


11. Gropler RJ, Siegel BA, Lee KJ, Moerlein SM, Perry DJ, Bergmann SR, et al. Nonuniformity in myocardial accumulation of fluorine-18-fluorodeoxyglucose in normal fasted humans. J Nucl Med. 1990. 31:1749–1756.
12. Schelbert HR. 18F-deoxyglucose and the assessment of myocardial viability. Semin Nucl Med. 2002. 32:60–69.
13. Sawada S, Elsner G, Segar DS, O'Shaughnessy M, Khouri S, Foltz J, et al. Evaluation of patterns of perfusion and metabolism in dobutamine-responsive myocardium. J Am Coll Cardiol. 1997. 29:55–61.
14. Ince C, Ashruf JF, Avontuur JA, Wieringa PA, Spaan JA, Bruining HA. Heterogeneity of the hypoxic state in rat heart is determined at capillary level. Am J Physiol. 1993. 264:H294–H301.


15. Matsumoto T, Goto M, Tachibana H, Ogasawara Y, Tsujioka K, Kajiya F. Microheterogeneity of myocardial blood flow in rabbit hearts during normoxic and hypoxic states. Am J Physiol. 1996. 270:H435–H441.
16. Watanabe N, Akasaka T, Toyota E, Fujimoto K, Kajita T, Shigeto F, et al. Three-dimensional microstructural abnormality of the coronary capillary network after myocardial reperfusion--comparison between 'reflow' and 'no-reflow'. Circ J. 2004. 68:868–872.


17. Deussen A. Local myocardial glucose uptake is proportional to, but not dependent on blood flow. Pflugers Arch. 1997. 433:488–496.


18. Bacharach SL, Bax JJ, Case J, Delbeke D, Kurdziel KA, Martin WH, et al. PET myocardial glucose metabolism and perfusion imaging: Part 1-Guidelines for data acquisition and patient preparation. J Nucl Cardiol. 2003. 10:543–556.
19. Bax JJ, Veening MA, Visser FC, van Lingen A, Heine RJ, Cornel JH, et al. Optimal metabolic conditions during fluorine-18 fluorodeoxyglucose imaging; a comparative study using different protocols. Eur J Nucl Med. 1997. 24:35–41.