Abstract
Purpose
Tumor necrosis factor-alpha (TNF-α) is a proinflammatory cytokine that has been implicated in many aspects of the airway pathology in asthma. TNF-α blocking strategies are now being tried in asthma patients. This study investigated whether TNF-α blocking therapy inhibits airway inflammation and airway hyperresponsiveness (AHR) in a mouse model of asthma. We also evaluated the effect of TNF-α blocking therapy on cytokine production and adhesion molecule expression.
Materials and Methods
Ovalbumin (OVA) sensitized BALB/c female mice were exposed to intranasal OVA administration on days 31, 33, 35, and 37. Mice were treated intraperitoneally with soluble TNF-α receptor (sTNFR) during the OVA challenge.
Results
There were statistically significant decreases in the numbers of total cell and eosinophil in bronchoalveolar lavage fluid (BALF) in the sTNFR treated group compared with the OVA group. However, sTNFR-treatment did not significantly decrease AHR. Anti-inflammatory effect of sTNFR was accompanied with reduction of T helper 2 cytokine levels including interleukin (IL)-4, IL-5 and IL-13 in BALF and vascular cell adhesion molecule 1 expression in lung tissue.
Asthma is a T helper 2 (Th2)-mediated inflammatory airway disease, characterized by airway hyperresponsiveness (AHR), chronic eosinophilic inflammation, episode of reversible bronchoconstriction, and mucus hypersecretion. In these responsies, several cytokines are considered to take part in a pivotal role. Although Th2 cytokines, including interleukin (IL)-4, IL-5 and IL-13, are important in asthma,1 tumor necrosis factor (TNF)-α has been implicated in the inflammatory response, seen in asthma.2 TNF-α is a multifunctional proinflammatory cytokine, and a chemoattractant for neutrophils and eosinophils.3 It increases the cytotoxic effect of eosinophils on endothelial cells,4 epithelial expression of adhesion molecules, such as intercellular adhesion molecule 1 (ICAM-1) and vascular cell adhesion molecule 1 (VCAM-1),6 and the contractile function of smooth muscles,7 and is involved in the activation of T cells.5 Howarth et al.8 reported that TNF-α concentration in bronchoalveolar lavage fluid (BALF) and TNF-α protein and messenger RNA (mRNA) expression in bronchial biopsy specimens were increased in patients with severe asthma compared those with mild disease.
In general, the mainstay treatment of asthma is an inhalation of corticosteroids alone or in combination with long-acting β2-agonist. Current therapies for asthma are focused on optimal control of symptoms, however, there is a significant population of severe asthmatics that do not respond well to standard therapies.9 Therefore, there is a need for innovative therapies which are aimed to prevent structural changes in the airways. Based on the current understanding of the pathophysiology of asthma, the development of anti-cytokine therapeutics could be useful. Recently, TNF-α blocking strategies are now being tried in asthma, and show improvement in symptom score, lung function and quality of life.8,10,11 However, despite an apparent clinical efficacy observed, the mechanism of action of TNF-α blocking therapies remains unclear.
The aim of this study was to determine whether TNF-α blocking therapy inhibits the airway inflammation and AHR in murine asthma model. We also investigated the effect of TNF-α blocking therapy on cytokine production and adhesion molecule expression.
BALB/c female mice (n = 16 mice/group; The Dae-Han Experimental Animal Center, Daejeon, Korea), 7 week of age, were immunized by subcutaneous injection on days 0, 7, 14, and 21 with 25 µg of ovalbumin (OVA, grade V; Sigma-Aldrich, St. Louis, MO, USA), which was adsorbed to 1 mg of alum (Sigma-Aldrich) in 200 µL of normal saline. Intranasal OVA challenges [20 ng/50 µL in phosphate-buffered saline, PBS] were carried out days 31, 33, 35, and 37 under isoflurane (Vedco, St. Joseph, MO, USA) anesthesia. Age- and gender-matched control mice were treated in the same way with PBS without OVA. All animal experimental protocols were approved by The Catholic University of Korea, Animal Subjects Committees.
Mice were treated with soluble TNF receptor (sTNFR) (Etanercept®, Wyeth, Muenster, Germany), as diluted in sterile normal saline. sTNFR was given by intraperitoneal administration (4.0 mg/kg in 100 µL normal saline, daily) starting at the same time with intranasal OVA challenge.
Twenty four hours after the last challenge, mice were placed in a barometric plethysmographic chamber (OCP 2000; Allmedicus, Anyang, Korea). Methacholine was used at concentrations of 3.125 mg/mL, 6.25 mg/mL, 12.5 mg/mL, 25 mg/mL, and 50 mg/mL. A total of 3 mL of each concentration was inhaled for 3 minutes as an aerosol, prepared by an Ultra-Neb (3650p, DeVilbiss, Pennsylvania, PA, USA), after which time Penh (enhanced pause) value was measured for 3 minutes. Bronchopulmonary resistance was expressed as Penh, which was calculated, according to the manufacturer's protocols. Results are expressed as percentage increases in Penh after challenge with each concentration of methacholine, where the baseline Penh (after saline challenge) was set as 100%.
Mice were killed by CO2 asphyxiation after measuring airway responsiveness. The trachea was exposed and cannulated with silicone tubing attached to a 23-guage needle on an 800 µL tuberculin syringe. After instillation of 1 mL of sterile PBS through the trachea into the lung, BALF was withdrawn. The total cells in BALF were counted using a hemacytometer. The BALF was cytospinned (3 minutes at 1,000 rpm) onto microscope slides and stained with Diff-Quick. The percentages of BALF macrophages, eosinophils, lymphocytes and neutrophils were obtained by counting 400 leukocytes on randomly selected portions of the slide by light microscopy. Supernatants were stored at -70℃.
The concentrations of IL-4, IL-5, IL-10, and IL-13 (R & D system, Minneapolis, MN, USA) in the BALF were measured using enzyme-linked immunosorbent assay (ELISA) kits, according to the manufacturer's instructions.
Six-micron-thick sections of the lung from each paraffin block were deparaffinized with xylene and hydrated in ethanol. For immunohistochemical detection of VCAM-1 (R & D systems, Minneapolis, MN, USA), the lung sections were incubated overnight at 4℃ with either a primary monoclonal antibody directed against VCAM-1, or a negative control mouse serum instead of the primary antibody. Immunoreactivity was detected by sequential incubation of lung sections with a biotinylated secondary antibody, followed by peroxidase reagent and 3-amino-9-ethylcarbazole (AEC) chromogen.
Total protein was isolated from the lung by homogenization in a buffer containing 50 mM 4-(2-hydroxyethyl)-1-piperazineethanesulfonic acid (HEPES) (pH 7.4), 1% Noniodet P-40, 0.5% deoxycholate, 5 mM ethylenediaminetetraacetic acid (EDTA), 1 mM sodium orthovanadate, 5 mM NaF, and phosphatase and protease inhibitor cocktails (Sigma-Aldrich). The lysates were centrifuged at 14,000 g for 15 minutes at 4℃, the supernatants were collected, their total protein content was determined using a conventional method (Pierce Chemical Co., Rockford, IL, USA), and aliquots were stored at -70℃ until assay. Equal amounts of sample proteins were resolved using 10% SDS-PAGE and transferred to a polyvinylidene difluoride membrane by electroblotting in a buffer containing Tris-HCl (25 mM), glycine (192 mM), and methanol (20%, v/v). After transfer, the blots were incubated in 5% powdered milk in Tween/Tris-buffered saline, containing 10 mM Tris-HCl (pH 7.5), 0.15 M NaCl, and 0.1% Tween 20, at room temperature for 1 hour, incubated overnight with anti-VCAM-1 antibodies (R & D systems, Minneapolis, MN, USA) at 4℃, washed with Tween/Tris-buffered saline, and exposed to corresponding horseradish peroxidase-conjugated IgG for 1 hour. The labeled band was detected using enhanced chemiluminescence detection kit and developed with Hyperfilm-enhanced chemiluminescence (Amersham Pharmacia Biotech, Piscataway, NJ, USA).
Results from each groups were compared by ANOVA with the nonparametric Kruskal-Wallis test, followed by posttesting with Dunn's multiple comparison of means. A statistical software package (Graph Pad Prism, San Diego, CA, USA) was used for the analysis. A p value less than 0.05 was considered statistically significant. All results are given as means ± SEM.
The numbers of total cells, macrophages, neutrophils, lymphocytes and eosinophils in BALF were significantly increased in the OVA group compared with those in the control group. Intraperitoneal administration of sTNFR significantly attenuated the increase of total cells and eosinophils in the asthmatic airway lumens, although the sTNFR-treated group still retained airway inflammation compared with the control group (OVA group vs. the sTNFR treated group; 446.25 ± 64.97 vs. 251.50 ± 27.07, 389.46 ± 56.84 vs. 206.21 ± 21.50, respectively) (p < 0.01) (Table 1).
To assess AHR, we performed a bronchial challenge test using methacholine. Fig. 1 shows dose response curve of AHR to methacholine. In the OVA group, AHR to methacholine was increased more than the control group. However, there was no significant difference, although the sTNFR treated group tended to decrease AHR compared with the OVA group (206.68 ± 27.75 vs. 257.55 ± 62.67, 311.83 ± 45.06 vs. 370.52 ± 66.29, 509.29 ± 85.65 vs. 469.36 ± 75.02, 793.74 ± 164.64 vs. 672.50 ± 115.16, 1162.70 ± 205.22 vs. 884.60 ± 226.76, at the respective concentration of methacholine) (p > 0.05).
In BALF, the levels of a Th2 cytokines, including IL-4, IL-5, and IL-13 were significantly decreased in the sTNFR treated group compared with the OVA group (96.01 ± 16.08 vs. 50.67 ± 7.79*, 223.6 ± 40.3 vs. 136.6 ± 21.7*, 203.3 ± 20.1 vs. 116.6 ± 15.5**, respectively ; *p < 0.05, **p < 0.01) (Figs. 2A, B and C).
In addition, IL-10 in BALF was also significantly decreased in the sTNFR treated group compared with the OVA group (29.90 ± 5.41 vs. 14.74 ± 3.54) (p < 0.05) (Fig. 2D).
We demonstrated that sTNFR treatment reduced the eosinophils recruitment in BALF. VCAM-1, which is shown to play important roles in the recruitment of eosinophils, is upregulated by TNF-α.6 Therefore, we investigated the expression patterns of VCAM-1 in the lung following sTNFR administration in order to investigate the mechanism of anti-inflammatory effect of sTNFR. As shown in Figs. 3 and 4, an increase in the expression of VCAM-1 was observed during prolonged allergen challenge, while administration of sTNFR considerably decreased the VCAM-1 expression.
This study demonstrated in a mouse model of acute asthma that sTNFR reduced airway inflammation, which was accompanied with reduction of several cytokines levels and adhesion molecule expression.
TNF-α, described first in macrophages and monocytes,12 is an important cytokine in the innate immune response, which plays a key role in the immediate host defense against invading microorganisms before activation of the adaptive immune system.13 TNF-α has also been implicated in the pathophysiologic mechanism of several chronic inflammatory disease, including Crohn's disease, rheumatoid arthritis, and psoriatic arthritis.14,15 Blockade of TNF-α has been used to treat human diseases of chronic inflammation. The synthesis and activity of TNF-α can be blocked by various ways including monoclonal antibodies to TNF-α, soluble TNF receptors, and TNF-α converting enzyme inhibitor.16,17 In addition, involvement of TNF-α in asthma has been suggested by various animal studies, showing alteration of the contractile properties of the airway smooth muscle18 and regulation of AHR and neutrophilia.19 Furthermore, TNF-α has been known to have several properties that might be relevant to severe asthma, including induction of glucocorticoid resistance,20 myocyte proliferation,21 and stimulation of fibroblast growth and maturation into myofibroblasts by promoting transforming growth factor (TGF)-β expression.22 Although TNF-α has been postulated to play an important role in the pathogenesis of asthma, the role of TNF-α in asthma remains controversial.23,24 There is an increasing evidence to indicate that TNF-α is responsible for inflammatory responses in asthma. Broide et al.23 showed decreased pulmonary inflammation in TNF-α receptor knockout mice using an OVA model, and our study also demonstrated that TNF-α blocking therapy in asthma suppressed the airway inflammation, although the sTNFR-treated group still retained airway inflammation, compared with the control group, rather than AHR.
The inhaled of administration recombinant TNF-α to normal subjects led to the development of AHR.19 A postmortem study of fatal and nonfatal asthma showed a marked increase of mast cell degranulation in the airway smooth muscle (ASM) bundle in both the large and small airways,25 and increased numbers of mast cells are associated with increased ASM shortening in fatal asthma.26 Therefore, it is likely that the close proximity of these cells will facilitate mast cell-derived TNF-α activation of ASM and contribute to the development of AHR. Contrary to expectation, our result didn't show a significant decrease of AHR after sTNFR treatment. In our experiment, mice were treated with 4 times sTNFR during OVA challenge. It was presumed that the dose was inadequate to ASM contractibility, using the value of Penh as the parameter of AHR in murine asthma model. Recently, however, there is a concensus that the value of Penh dose not always correlate with airway resistance.27 Glaab et al.28 reported that the invasive or other noninvasive methods have superior sensitivity compared to the widely used Phen method. This is a potential limitation of our study.
TNF-α is produced by various cell types in response to allergic pulmonary inflammation, including mast cells, macrophages, neutrophils, eosinophils, and epithelial cells.19 Especially, mast cells are a major potential source of TNF-α.29 It is well known that mast cell-derived TNF-α can affect T lymphocyte activity. TNF-α can enhance proliferation, survival, and recruitment of T lymphocyte.30,31 Also, T lymphocyte activated with TNF-α can induce mast cells to secrete metalloproteinase 9.32 Nakae et al.33 reported that mast cell-derived TNF-α is not required for the induction of specific memory T cells in the sensitization phase, but enhances lung Th2 cytokine production in response to antigen challenge in sensitized mice. We have observed significant reduction of BALF IL-4, IL-5, and IL-13 levels in the sTNFR-treated group compared with the OVA group, which is consistent with the results of previous studies. These findings suggest that antiinflammatory effect of sTNFR is associated with inhibition of Th2 cytokine production in asthma.
IL-10, an important immunoregulatory cytokine, is well known to inhibit allergic inflammation. The type 1 regulatory T lymphocyte (Tr1) is the major source of IL-10.34 Naturally occurring forkhead box P3 (Foxp3)+CD4+CD25+ regulatory T (Treg) cells develop in the thymus, and Tr1 cells can also be induced from effector T cells during inflammatory processes in peripheral tissues. Skewing of allergic-specific effector T cells to a Tr1 phenotype appears to be a critical event in successful allergen-specific immunotherapy and glucocorticoids and β2-agonists treatment. Tr1 suppresses Th2 cells and effector cells of allergic inflammation, such as eosinophils, mast cells, and basophils.35 In our studies, however, we found that sTNFR treatment led to paradoxical reduction of IL-10. Further studies are required to confirm the interaction of TNF-α with IL-10 in allergic asthma.
TNF-α stimulates the production of IL-8, regulated upon activation of normal T cells expressed and secreted (RANTES) and granulocyte-macrophage colony-stimulating factor (GM-CSF) by airway epithelial cells, which increase the expression of adhesion molecules such as ICAM-1 and VCAM-1, which are involved in the recruitment of inflammatory cells to tissue. The up-regulation of adhesion molecules on the airway vascular endothelium is important for eosinophil recruitment.6 ASM cells also express ICAM-1 and VCAM-1, and through this mechanism, TNF-α aids in the binding of activated T lymphocytes to ASM.36 TNF-α levels are increased during asthma exacerbations, and this change is associated with increased expression of ICAM-1, VCAM-1, and E-selectin.37 In our present study, we found that the expression of VCAM-1 in the lung tissues was significantly decreased in the sTNFR-treatment group compared with the OVA group, suggesting that anti-inflammatory effect of sTNFR may in part be dependent on down-regulation of vascular adhesion molecules and consequent reduction of cell traffic into airway.
In conclusion, we have found that sTNFR treatment of murine model of acute asthma resulted in reduction of airway inflammation, which was accompanied with the reduction of Th2 cytokine and adhesion molecule. These results suggest that sTNFR treatment can suppress the airway inflammation via regulation of Th2 cytokine production and adhesion molecule expression in bronchial asthma. An understanding of the mechanisms of TNF-α blockade will facilitate optimal therapeutic regimens for bronchial asthma in the clinic.
Figures and Tables
Fig. 1
Effect of sTNFR treatment on airway hyperresponsiveness (AHR) to inhaled methacholine (Mch). AHR was measured 24 hours after the final ovalbumin (OVA) challenge using a Allmedicus system by which mice were exposed to increasing concentrations of methacholine (3.125 - 50 mg/mL). Values are expressed as mean, n = 16 mice/group in three separated experiments. sTNFR, solubel tumor necrosis factor-alpha receptor.
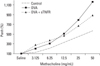
Fig. 2
Effect of sTNFR on IL-4 (A), IL-5 (B), IL-13 (C) and IL-10 (D) levels in bronchoalveolar lavage fluid (BALF). Mice were sacrified 24 hours after the final ovalbumin (OVA) challenge, and BALF were separated and cytokines levels were measured with ELISA, as described in Material and Methods. Values are expressed as mean ± SEM, n = 16 mice/group in three separated experiments, and *p < 0.05, **p < 0.01 in comparison with the OVA group. IL, interleukin; sTNFR, solubel tumor necrosis factor-alpha receptor.
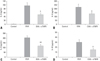
Fig. 3
Effect of sTNFR on VCAM-1. Mice were sacrificed 24 hours after the final ovalbumin challenge. (A) Expression of VCAM-1 in lung tissue was determined by Western blotting. (B) Densimometric analyses are presented as the ratio of VCAM-1 relative to actin, and *p < 0.05 in comparison with the OVA group. OVA, ovalbumin; sTNFR, solubel tumor necrosis factor-alpha receptor; VCAM-1, vascular cell adhesion molecule 1.
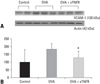
Fig. 4
Photomicrographs showing staining of lung tissue with antibodies to VCAM-1. Mice were sacrificed 24 hours after the final ovalbumin challenge. Paraffin-embeded lung tissue sections were stained with specific antibody to VCAM-1. Immunohistochemical detection of VCAM-1 was measured with monoclonal antibody, as described in Material and Methods. Positive staining is depicted in pink. (A) Control group. (B) OVA group. (C) sTNFR treated group (×200). VCAM-1, vascular cell adhesion molecule 1; OVA, ovalbumin; sTNFR, solubel tumor necrosis factor-alpha receptor.
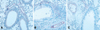
ACKNOWLEDGEMENTS
The authors thank Jung Me Lee and Jin Suk Kim for their technical help throught their experiments.
References
1. Foster PS, Martinez-Moczygemba M, Huston DP, Corry DB. Interleukins-4, -5, and -13: emerging therapeutic targets in allergic disease. Pharmacol Ther. 2002. 94:253–264.


2. Meiler F, Zimmermann M, Blaser K, Akdis CA, Akdis M. T-cell subsets in the pathogenesis of human asthma. Curr Allergy Asthma Rep. 2006. 6:91–96.
3. Lukacs NW, Strieter RM, Chensue SW, Widmer M, Kunkel SL. TNF-alpha mediates recruitment of neutrophils and eosinophils during airway inflammation. J Immunol. 1995. 154:5411–5417.
4. Slungaard A, Vercellotti GM, Walker G, Nelson RD, Jacob HS. Tumor necrosis factor alpha/cachectin stimulates eosinophil oxidant production and toxicity towards human endothelium. J Exp Med. 1990. 171:2025–2041.


5. Scheurich P, Thoma B, Ucer U, Pfizenmaier K. Immunoregulatory activity of recombinant human tumor necrosis factor (TNF)-alpha: induction of TNF receptors on human T cells and TNF-alpha-mediated enhancement of T cell responses. J Immunol. 1987. 138:1786–1790.
6. Lassalle P, Gosset P, Delneste Y, Tsicopoulos A, Capron A, Joseph M, et al. Modulation of adhesion molecule expression on endothelial cells during the late asthmatic reaction: role of macrophage-derived tumour necrosis factor-alpha. Clin Exp Immunol. 1993. 94:105–110.


7. Pennings HJ, Kramer K, Bast A, Buurman WA, Wouters EF. Tumour necrosis factor-alpha induces hyperreactivity in tracheal smooth muscle of the guinea-pig in vitro. Eur Respir J. 1998. 12:45–49.


8. Howarth PH, Babu KS, Arshad HS, Lau L, Buckley M, McConnell W, et al. Tumour necrosis factor (TNFalpha) as a novel therapeutic target in symptomatic corticosteroid dependent asthma. Thorax. 2005. 60:1012–1018.


9. Chanez P, Wenzel SE, Anderson GP, Anto JM, Bel EH, Boulet LP, et al. Severe asthma in adults: what are the important questions? J Allergy Clin Immunol. 2007. 119:1337–1348.


10. Berry MA, Hargadon B, Shelley M, Parker D, Shaw DE, Green RH, et al. Evidence of a role of tumor necrosis factor alpha in refractory asthma. N Engl J Med. 2006. 354:697–708.


11. Erin EM, Leaker BR, Nicholson GC, Tan AJ, Green LM, Neighbour H, et al. The effects of a monoclonal antibody directed against tumor necrosis factor-alpha in asthma. Am J Respir Crit Care Med. 2006. 174:753–762.


12. Pennica D, Nedwin GE, Hayflick JS, Seeburg PH, Derynck R, Palladino MA, et al. Human tumour necrosis factor: precursor structure, expression and homology to lymphotoxin. Nature. 1984. 312:724–729.


14. Choy EH, Panayi GS. Cytokine pathways and joint inflammation in rheumatoid arthritis. N Engl J Med. 2001. 344:907–916.


15. Present DH, Rutgeerts P, Targan S, Hanauer SB, Mayer L, van Hogezand RA, et al. Infliximab for the treatment of fistulas in patients with Crohn's disease. N Engl J Med. 1999. 340:1398–1405.


16. Bryan SA, Leckie MJ, Hansel TT, Barnes PJ. Novel therapy for asthma. Expert Opin Investig Drugs. 2000. 9:25–42.
17. Holgate ST. Cytokine and anti-cytokine therapy for the treatment of asthma and allergic disease. Cytokine. 2004. 28:152–157.
18. Amrani Y, Chen H, Panettieri RA Jr. Activation of tumor necrosis factor receptor 1 in airway smooth muscle: a potential pathway that modulates bronchial hyper-responsiveness in asthma? Respir Res. 2000. 1:49–53.
19. Thomas PS, Yates DH, Barnes PJ. Tumor necrosis factor-alpha increases airway responsiveness and sputum neutrophilia in normal human subjects. Am J Respir Crit Care Med. 1995. 152:76–80.
20. Franchimont D, Martens H, Hagelstein MT, Louis E, Dewe W, Chrousos GP, et al. Tumor necrosis factor alpha decreases, and interleukin-10 increases, the sensitivity of human monocytes to dexamethasone: potential regulation of the glucocorticoid receptor. J Clin Endocrinol Metab. 1999. 84:2834–2839.


21. Amrani Y, Panettieri RA Jr, Frossard N, Bronner C. Activation of the TNF alpha-p55 receptor induces myocyte proliferation and modulates agonist-evoked calcium transients in cultured human tracheal smooth muscle cells. Am J Respir Cell Mol Biol. 1996. 15:55–63.
22. Desmoulière A, Geinoz A, Gabbiani F, Gabbiani G. Transforming growth factor-beta 1 induces alpha-smooth muscle actin expression in granulation tissue myofibroblasts and in quiescent and growing cultured fibroblasts. J Cell Biol. 1993. 122:103–111.


23. Broide DH, Stachnick G, Castaneda D, Nayar J, Sriramarao P. Inhibition of eosinophilic inflammation in allergen-challenged TNF receptor p55/p75--and TNF receptor p55-deficient mice. Am J Respir Cell Mol Biol. 2001. 24:304–311.


24. Rudmann DG, Moore MW, Tepper JS, Aldrich MC, Pfeiffer JW, Hogenesch H, et al. Modulation of allergic inflammation in mice deficient in TNF receptors. Am J Physiol Lung Cell Mol Physiol. 2000. 279:L1047–L1057.


25. Carroll NG, Mutavdzic S, James AL. Distribution and degranulation of airway mast cells in normal and asthmatic subjects. Eur Respir J. 2002. 19:879–885.


26. Chen FH, Samson KT, Miura K, Ueno K, Odajima Y, Shougo T, et al. Airway remodeling: a comparison between fatal and nonfatal asthma. J Asthma. 2004. 41:631–638.


27. Bates J, Irvin C, Brusasco V, Drazen J, Fredberg J, Loring S, et al. The use and misuse of Penh in animal models of lung disease. Am J Respir Cell Mol Biol. 2004. 31:373–374.


28. Glaab T, Ziegert M, Baelder R, Korolewitz R, Braun A, Hohlfeld JM, et al. Invasive versus noninvasive measurement of allergic and cholinergic airway responsiveness in mice. Respir Res. 2005. 6:139.


29. Gordon JR, Galli SJ. Mast cells as a source of both preformed and immunologically inducible TNF-alpha/cachectin. Nature. 1990. 346:274–276.


30. Nakae S, Suto H, Kakurai M, Sedgwick JD, Tsai M, Galli SJ. Mast cells enhance T cell activation: Importance of mast cell-derived TNF. Proc Natl Acad Sci U S A. 2005. 102:6467–6472.


31. Tartaglia LA, Goeddel DV, Reynolds C, Figari IS, Weber RF, Fendly BM, et al. Stimulation of human T-cell proliferation by specific activation of the 75-kDa tumor necrosis factor receptor. J Immunol. 1993. 151:4637–4641.
32. Baram D, Vaday GG, Salamon P, Drucker I, Hershkoviz R, Mekori YA. Human mast cells release metalloproteinase-9 on contact with activated T cells: juxtacrine regulation by TNF-alpha. J Immunol. 2001. 167:4008–4016.


33. Nakae S, Ho LH, Yu M, Monteforte R, Iikura M, Suto H, et al. Mast cell-derived TNF contributes to airway hyperreactivity, inflammation, and TH2 cytokine production in an asthma model in mice. J Allergy Clin Immunol. 2007. 120:48–55.


34. Del Prete G, De Carli M, Almerigogna F, Giudizi MG, Biagiotti R, Romagnani S. Human IL-10 is produced by both type 1 helper (Th1) and type 2 helper (Th2) T cell clones and inhibits their antigen-specific proliferation and cytokine production. J Immunol. 1993. 150:353–360.
35. Wu K, Bi Y, Sun K, Wang C. IL-10-producing type 1 regulatory T cells and allergy. Cell Mol Immunol. 2007. 4:269–275.
36. Panettieri RA Jr, Lazaar AL, Puré E, Albelda SM. Activation of cAMP-dependent pathways in human airway smooth muscle cells inhibits TNF-alpha-induced ICAM-1 and VCAM-1 expression and T lymphocyte adhesion. J Immunol. 1995. 154:2358–2365.
37. Kobayashi T, Hashimoto S, Imai K, Amemiya E, Yamaguchi M, Yachi A, et al. Elevation of serum soluble intercellular adhesion molecule-1 (sICAM-1) and sE-selectin levels in bronchial asthma. Clin Exp Immunol. 1994. 96:110–115.