Abstract
Purpose
Lithium-pilocarpine induced status epilepticus (LPSE) causes selective and age-dependent neuronal death, although the mechanism of maturation-related injury has not yet been clarified. The activating transcription factor-2 (ATF-2) protein is essential for the normal development of mammalian brain and is activated by c-Jun N-terminal kinase (JNK). It induces the expression of the c-jun gene and modulates the function of the c-Jun protein, a mediator of neuronal death and survival. Therefore, we investigated the expression of c-Jun and ATF-2 protein in the immature and adult rat hippocampus to understand their roles in LPSE-induced neuronal death.
Materials and Methods
Lithium chloride was administrated to P10 and adult rats followed by pilocarpine. Neuronal injury was assessed by silver and cresyl violet staining, performed 72 hours after status epilepticus. For evaluation of the expression of ATF-2 and c-Jun by immunohistochemical method and Western blot, animals were sacrificed at 0, 4, 24, and 72 hours after the initiation of seizure.
Results
Neuronal injury and expression of c-Jun were maturation-dependently increased by LPSE, whereas ATF-2 immunoreactivity decreased in the mature brain. Since both c-Jun and ATF-2 are activated by JNK, and targets and competitors in the same signal transduction cascade, we could speculate that ATF-2 may compete with c-Jun for JNK phosphorylation.
It is well established that the severity of neuronal injury caused by status epilepticus varies according to the injured area and age.1-4 In young rats, seizure duration is shorter, mortality rate is lower, neuronal injury is barely detectable, and the spontaneous recurrence of seizure hardly appears. In adult rats, on the other hand, seizures usually last for a longer time followed by recurrence, mortality rate is higher, and severe neuronal injury can be detected in selected area of the hippocampus, piriform cortex, and entorhinal cortex. The mechanism of maturation-related injury has not been yet clarified.
As the activating transcription factor-2 (ATF-2) and c-Jun are activated by c-Jun N-terminal kinase (JNK), these 2 proteins may be competitors in signal transduction cascades.5,6 ATF-2 is a crucial transcription factor for the normal development of the brain in mammals.7-9 c-Jun was reported to be involved in cell survival, and it is known to be fundamentally involved in apoptosis.10-15 Furthermore, ATF-2 and c-Jun are reported to counteract with each other: c-Jun suppresses the transcription of human gastric inhibitory peptide that is increased by ATF-2.16 Thus, expression of ATF-2 and c-Jun is inversely related, and ATF-2 expression is decreased rapidly in injured neurons while c-Jun expression is increased.17
We, therefore, speculated that the expression of these 2 proteins in injured neurons play an important role in the determination of the survival and death of cells, and examined the role of these 2 proteins in injured neurons by comparing the expression of ATF-2 and c-Jun at various ages in the lithium-pilocarpine model of status epilepticus.
Sprague-Dawley white adult rats, weighing 250-400 gm, were kept under the same conditions for over 6 weeks and fed with commercial solid food (Jeil animal food Co., Daejeon, Korea). P10 rats, 10 day-old rats, were litters and nursed by their mother. Forty-eight rats were used for immunohistochemical assessment of the expression of c-Jun and ATF-2 (24 per age group). Twenty-four rats were studied with Western blot (12 rats per age group), and 12 rats were evaluated with silver staining or Cresyl violet staining (6 rats per age group).
Lithium, pilocarpine, valium, phenobarbital, methylscopolamine, urethane, phosphate buffer, paraformaldehyde, FD Neuro-Silver™ Kit I (FD NeuroTechnologies, Ellicott, MD, USA), cresyl violet, DAKO LSAB® Kit (DAKO Corporation, Carpinteria, CA, USA), normal goat serum, phosphate buffered saline (PBS)-TX, ATF-2 polyclonal antibody (Santa Cruz Biotechnology, Santa Cruz, CA, USA), c-Jun monoclonal antibody (BD Transduction Lab, San Jose, CA, USA), biotinylated anti-mouse immunoglobulin (Ig)-G, peroxidase-labelled streptavidin, 3,3'-diaminobenzidine-tetra-hydrochloride (Sigma, St. Louis, MO, USA), 0.5 mol/L NaCl, 5 mmol/L Tris, 1% Nonidet P-40, glycerol, sodium dodecyl sulfate, protease inhibitor cocktail tablets (Roche Diagnostics, Indianapolis, IN, USA), and β-actin were used.
Lithium chloride (3 mEq/kg) was injected i.p., followed by pilocarpine (35 mg/kg) i.p. 24 hours later. Status epilepticus were staged every 30 minutes for 4 hours. To prevent the death due to status epilepticus in adult animals, valium (10 mg/kg) and phenobarbital (30 mg/kg) were injected i.p. hours after the onset of status epilepticus. Control animals were treated with saline instead of lithium and pilocarpine.
Neuronal injury was assessed by silver and cresyl violet staining, performed 72 hours after status epilepticus. For evaluation of the expression of ATF-2 and c-Jun by immunohistochemical method and Western blot, animals were sacrificed at 0, 4, 24, and 72 hours after the initiation of seizure.
Experimental animals were anesthetized with urethane, cardioperfused with 300 mL of saline, and fixed with 300 mL of 4% paraformaldehyde in 0.1 M phosphate buffer (pH 7.4), and the brain was removed. Brains were fixed overnight in the same fixative solution at 4℃, incubated in 0.1M phosphate buffer (pH 7.4) containing 30% sucrose until the brain sedimented, frozen rapidly in liquid nitrogen, and stored at -70℃ Coronal slices, 15 µm and 40 µm frozen, were then prepared (Frigomobil, Reichert Jung, Germany). To distinguish normal neuronal cells with well-maintained phospholipid of cellular membrane from injured neuronal cells with damaged membrane showing degenerative changes, 40 µm thick sections were treated with silver staining using FD NeuroSilver™ Kit I (FD Neuro-Technologies, Ellicott, MD, USA) and cresyl violet, and sections were examined by light microscopy (BX-50F, Olympus, Tokyo, Japan).
The hippocampus was divided into 3 areas, and the damage of each area was staged from 0 to 3 (Fig. 1): 0 indicating no damage, 1 damage in less than 1/3 areas, 2 damage between 1/3 and 2/3 areas, and 3 damage in more than 2/3 areas. Four to 8 sections per animal were evaluated. All sections were evaluated, and results were obtained from the average of analyzed sections. Data were analyzed for normality of variance, and samples with same variances were compared statistically by 1-way analysis of variance(ANOVA) applying Student-Newman-Keuls method.
Immunohistochemical analysis was performed on 60 µm thick free-floating tissue sections by the standard peroxidase-labeled streptavidin-biotin detection method {DAKO LSAB® Kit (DAKO Corporation, Carpinteria, CA, USA)}. Thus, sections were incubated in blocking solution (normal goat serum, 1 : 50) for 1 hour at room temperature, and then incubated overnight with the primary antibody ATF-2 (Santa Cruz Biotechnology, Santa Cruz, CA, USA), or c-Jun (BD Transduction Lab, San Jose, CA, USA) diluted with PBS-TX (1 : 1000) at room temperature. Then, prepared sections were incubated with the secondary antibody biotinylated anti-mouse IgG and peroxidase-labeled streptavidin for 2 hours at room temperature, stained with the mixture of 0.05% 3, 3'-diaminobenzidine-tetrahydrochloride (Sigma, St. Louis, MO, USA) and 0.01% H2O2.
To isolate proteins, the hippocampus was homogenized in homogenization buffer (1 : 1 wt/vol) containing 0.5 mol/L NaCl, 5 mmol/L Tris (pH 8.0), 1% Nonidet P-40, 10% glycerol, 0.1% sodium dodecyl sulfate, protease inhibitor cocktail tablets (Roche Diagnostics Corporation, Indianapolis, IN, USA). Proteins were resolved by sodium dodecyl sulfate-polyacrylamide gel electrophoresis and transferred to a nitrocellulose membrane. The membrane was incubated for 1 hour at room temperature with ATF-2 antiserum {1 : 1000, tris buffered saline (TBS) buffer} or c-Jun antiserum (1 : 500, TBS buffer). The blot was rinsed with TBS/Tween, incubated with secondary antibody horseradish peroxidase-conjugated rabbit antibody (1 : 5000 dilution) at room temperature, and protein band was examined by chemiluminescense (Amersham, Arlington Heights, IL, UK). To evaluate proteins in each well, the membrane was stained for β-actin and exposed to X-ray films (X-Omat, Kodak, Rochester, NY, USA). The intensity of band was determined (ScanJet 6300C, Hewlett-Packard, Paloalto, CA, USA), and the ratio with actin was calculated by Scion Image software (Scion Corp, Frederick, Maryland, USA). Statistical analysis was performed for between group comparison, using the Kruskall-Wallis and Mann-Whitney test.
The augmentation of neuronal injury was prominent in the dentate gyrus and the CA1 area, confirming that these are the most vulnerable sites of the hippocampus in lithium-pilocarpine induced status epilepticus (LPSE).
The expression of ATF-2 was increased slightly in the dentate gyrus, CA1 and CA3 4 hours after seizure, and returned to the basal level 24 hours after seizure in both age groups. After 72 hours of seizure, the expression of ATF-2 in CA1 and CA3 was decreased in adult rats, however, it remained at basal level in P10 rats (Fig. 4).
At baseline (0 hour of the seizure), the expression of c-Jun in the CA1 and CA3 was weaker in P10 rats than in adult rats. Four hours later, the expression was detected in the dentate gyrus, CA1 and CA3 of both P10 and adult rats. Twenty-four hours later, the expression was stronger in adult rats: the expression in P10 rats was undetected, whereas it was weakly detected in adult rats (Fig. 5).
Four hours after seizure, the immunoreactivity of ATF-2 and c-Jun was increased in both age groups. Twenty-four hours after seizure, the expression returned to basal level. At 0, 4, and 24 hours after seizure, the expression of ATF-2 protein in adult rats was lower than that in P10 rats. The expression of the c-Jun protein was higher in adult rats than in P10 rats (p = 0.05) (Figs. 6 and 7). The difference was statistically significant for both proteins at all times.
It is well established in animal studies that status epilepticus induces neuronal injury and the damaged level varies depending on the age of animals, type and duration of seizure, the causality of seizure, and genetic factors.18 During seizure, not only decreased oxygen delivery, but also the excitation due to the excess secretion of glutamate result in increases of intracellular calcium. This generates reactive oxygen species by mediating the activation of nitric oxide synthase, oxidative phosphorylation of mitochondria, and activation of lipase, protease, endonuclease and other enzymes which are harmful to cellular metabolism, resulting in neuronal cell injury and reorganization of the neuronal synaptic network.19
Several studies revealed that the immature brain is less vulnerable to morphological and physiological change after status epilepticus. This may be due to low density of the active synaptic network in the developing brain, to low energy consumption, and to relatively immature biochemical process, thus leading to cell death in children.20,21 In addition, age-dependent injury has been reported to be due to increase of the density of the muscarinic receptor and D2 receptor in the developing brain or difference in the expression of immediate early genes.1,2 Nevertheless, the mechanism of the age-dependent neuronal injury caused by status epilepticus has not yet been fully elucidated.
The ATF-2 expression in our experiment is in concordance with previous finding, showing initial increase after injury and a regression to basal level at 24 hours. We showed in this study that this finding was reduced with age.6,7,22,23
It has been shown that the expression of ATF-2 in the neuronal cells of the posterior ganglion in mice with transient focal ischemia, neurotmesis, or physical injury of the neuronal parenchyma, is suppressed for 1-4 hours and returns to the basal level a few days later. During degenerative process, AFT-2 is not detected in the area adjacent to physical injury, therefore, the down-regulation of ATF-2 correlating with the long-lasting stress to neuronal cells. The re-expression of ATF-2 indicates the normal transcription of genes in neuronal cells after neuronal injury.6 In quinolinic acid-induced seizure models, over-expression of JNK and c-Jun preceded cell death, whereas over-expression of ATF-2 correlated to cell survival.7 Experimental destruction of the ATF-2 locus caused the degeneration of the central nervous system and neurological abnormality.22 ATF-2 expression was increased after partial injury of retinal ganglion that resulted in survival, but decreased in the complete injury of retinal ganglion that resulted in death.23
In our experiment the c-Jun expression was increased in the mature brain: it increased 4 hours after seizure and returned to basal level 24 hours after. These results are similar to previous results showing that the c-Jun expression increases gradually for the initial 4 hours and returns to basal level 24 hours later,24-26 and that c-Jun expression was increased from 14 days after birth as the brain matures.27 The role of c-Jun in neuronal injury remains contradictory, because this protein acts on two distinct paths; neuroprotection28,29 or excitotoxicity-induced neuronal death.7,12,30-34 Yang et al.30 reported that the severity of the seizure induced by the glutamate receptor analog (kainic acid) is reduced and the apoptosis of neuronal cells in the hippocampus is prevented in mice with disturbed Jnk3 encoding gene. Furthermore, Lee et al.31 reported that the increase of c-Jun expression correlates statistically with the increase of the in situ end labeling positive apoptotic neurons during 4-8 weeks after kainic acid induced seizure. In addition, it is well known that the phosphorylation of c-Jun by JNK is involved in cell death; 1) in the nerve growth factor (NGF)-deprived sympathetic ganglion, neuronal apoptosis and excessive expression of c-Jun were detected,32 and programmed cell death of sympathetic neurons was prevented in c-Jun domain negative mutant,12 2) in the mouse nervous system, ischemia and plaque formation in Alzheimer, radiation, or other degenerative diseases were correlated to the expression of c-Jun, 3) the phosphorylation of c-Jun by JNK induced by injection of quinolinic acid to the brain cortex was detected prior to DNA fragmentation and cell death,7 4) in postmortem brain tissue of patients with Alzheimer disease, amyotrophic lateral sclerosis or multiple sclerosis, the c-Jun expression was found to be increased.33,34 In our present study, the c-Jun level was higher in adult rats than in P10 rats, therefore, we speculated that phosphorylated c-Jun may be increased and correlated to neuronal injury in the hippocampus.
We showed in the present study that the ATF-2 expression was higher in P10 rats, whereas the c-Jun expression was higher in adult rats. This is in agreement with the result of Reimold et al.22 that the ATF-2 expression decreases and the c-Jun expression increases with maturation of brain. The neuronal injury in hippocampus of adult rats can occur by increased phosphorylated c-Jun, because ATF-2 and c-Jun are target proteins of JNK.
In conclusion, the neuronal injury of immature rats was not severe compared to that of adult rats, ATF-2 expression decreased while c-Jun expression increased with brain maturation, and ATF-2 and c-Jun compete with each other for the phosphorylation by JNK. These results suggest a neuroprotective role of ATF-2 in the maturation-related neuronal cell death due to status epilepticus.
Figures and Tables
Fig. 1
Division of the hippocampus into 3 sectors for damage analysis. (A) CA1+CA2, (B) CA3, (C) CA3 + dentate gyrus (DG).
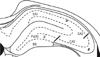
Fig. 2
Cresyl violet stain and silver stain are shown in P10 and adult rats 72 hours after the lithium-pilocarpine induced status epilepticus. CA1 pyramidal layer shows no evidence of neuronal drop out or degeneration in P10 and severe neuronal drop out and degeneration in adult rat (×400, scale bar: 250 µm).
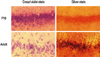
Fig. 3
Neuronal injury was quantitified, on the scale of 0-3 as described in the methods (0, no damage; 1, mild; 2, moderate; 3, severe). Neuronal damage score was 1.25 ± 0.27 in adult rats and 0 in P10 rats. There was significant difference between P10 and adult rats (p < 0.001, Mann-Whitney method).
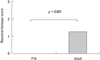
Fig. 4
Immunohistochemistry for ATF-2 was performed on slices obtained from P10 and adult rat, 0, 4, and 72 hours after the lithium-pilocarpine induced status epilepticus. There is increased expression of ATF-2 at 4 hours in P10 and adult rats, followed by return to baseline in P10 rats, while it decreased below baseline level in adult rats by 72 hours (×100, scale bar: 100 µm). ATF-2, activating transcription factor-2.
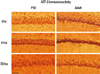
Fig. 5
Immunohistochemistry for c-Jun was performed on slices obtained from P10 and adult rat, 0, 4, and 72 hours after the lithium-pilocarpine induced status epilepticus. At 0 hour, there was weak expression in adult rat. There was increased expression in P10 and adult rats at 4 hours, followed by decreased below baseline level in P10 and adult rats at 72 hours (×100, scale bar: 100 µm).
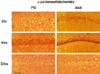
Fig. 6
Hippocampi were harvested from animals (n = 3 each age) at 0, 4 and 24 hours after the lithium-pilocarpine induced status epilepticus. Extracted proteins were run on SDS-PAGE and blotted for ATF-2 immunostaining. Actin immunoreactivity was used to standardize the optical density for bar graph representation. P10 has high expression. There is an induction at 4 hours in each age group. The difference between P10 and Adult was statistically significant (*p < 0.05). SDS-PAGE, sodium dodecyl sulfate polyacrylamide gel-electrophoresis; ATF-2, activating transciption factor.
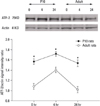
Fig. 7
Hippocampi were harvested from animals (n=3 each age) at 0, 4 and 24 hours after the lithium-pilocarpine induced status epilepticus. Extracted proteins were run on SDS-PAGE and blotted for c-Jun immunostaining. Actin immunoreactivity was used to standardize the optical density for bar graph representation. P10 has low expression. The difference between P10 and adult was statistically significant (*p < 0.05). SDS-PAGE, sodium dodecyl sulfate polyacrylamide gel-electrophoresis.

ACKNOWLEDGEMENTS
This study was supported by a grant from the Korea Health 21 R&D Project (A060093), Ministry of Health & Welfare, Republic of Korea.
References
1. Dubé C, André V, Covolan L, Ferrandon A, Marescaux C, Nehlig A. C-Fos, Jun D and HSP72 immunoreactivity, and neuronal injury following lithium-pilocarpine induced status epilepticus in immature and adult rats. Brain Res Mol Brain Res. 1998. 63:139–154.


2. De Bruin VM, Marinho MM, De Sousa FC, Viana GS. Behavioral and neurochemical alterations after lithium-pilocarpine administration in young and adult rats: a comparative study. Pharmacol Biochem Behav. 2000. 65:547–551.


3. Cavalheiro EA, Silva DF, Turski WA, Calderazzo-Filho LS, Bortolotto ZA, Turski L. The susceptibility of rats to pilocarpine-induced seizures is age-dependent. Brain Res. 1987. 465:43–58.


4. Priel MR, dos Santos NF, Cavalheiro EA. Developmental aspects of the pilocarpine model of epilepsy. Epilepsy Res. 1996. 26:115–121.


5. Ham J, Babij C, Whitfield J, Pfarr CM, Lallemand D, Yaniv M, et al. A c-Jun dominant negative mutant protects sympathetic neurons against programmed cell death. Neuron. 1995. 14:927–939.


6. Eilers A, Whitfield J, Babij C, Rubin LL, Ham J. Role of the Jun kinase pathway in the regulation of c-Jun expression and apoptosis in sympathetic neurons. J Neurosci. 1998. 18:1713–1724.


7. van Dam H, Wilhelm D, Herr I, Steffen A, Herrlich P, Angel P. ATF-2 is preferentially activated by stress-activated protein kinases to mediate c-jun induction in response to genotixic agents. EMBO J. 1995. 14:1798–1811.


8. Gupta S, Campbell D, Dérijard B, Davis RJ. Transcription factor ATF-2 regulation by the JNK signal transduction pathway. Science. 1995. 267:389–393.


9. Robinson GA. Changes in the expression of transcription factors ATF-2 and Fra-2 after axotomy and during regeneration in rat retinal ganglion cells. Brain Res Mol Brain Res. 1996. 41:57–64.


10. Martin-Villalba A, Winter C, Brecht S, Buschmann T, Zimmermann M, Herdegen T. Rapid and long-lasting suppression of the ATF-2 transcription factor is a common response to neuronal injury. Brain Res Mol Brain Res. 1998. 62:158–166.


11. Ferrer I, Blanco R, Carmona M. Differential expression of active, phosphorylation-dependent MAP kinases, MAPK/ERK, SAPK/JNK and p38, and specific transcription factor substrates following quinolinic acid excitotoxicity in the rat. Brain Res Mol Brain Res. 2001. 94:48–58.


12. Cheung NS, Carroll FY, Larm JA, Beart PM, Giardina SF. Kainate-induced apoptosis correlates with c-Jun activation in cultured cerebellar granular cells. J Neurosci Res. 1998. 52:69–82.


13. Kitayama T, Ogita K, Yoneda Y. Sustained potentiation of AP1 DNA binding is not always associated with neuronal death following systemic administration of kainic acid in murine hippocampus. Neurochem Int. 1999. 35:453–462.


14. Dragunow M, Preston K. The role of inducible transcription factors in apoptotic nerve cell death. Brain Res Brain Res Rev. 1995. 21:1–28.


15. Gass P, Herdegen T. Neuronal expression of AP-1 proteins in excitotoxic-neurodegenerative disorders and following nerve fiber lesions. Prog Neurobiol. 1995. 47:257–290.


16. Someya Y, Inagaki N, Maekawa T, Seino Y, Ishii S. Two 3', 5' - cyclic-adenosine monophosphate response elements in the promoter region of the human gastric inhibitory polypeptide gene. FEBS Lett. 1993. 317:67–73.


17. Takeda J, Maekawa T, Sudo T, Seino Y, Imura H, Seito N, et al. Expression of the CRE-BP1 transcriptional regulator binding to the cyclic AMP response element in central nervous system, regenerating liver, and human tumors. Oncogene. 1991. 6:1009–1014.
19. Marks JD, Friedman JE, Haddad GG. Vulnerability of CA1 neurons to glutamate is developmentally regulated. Brain Res Dev Brain Res. 1996. 97:194–206.


20. Berger ML, Tremblay E, Nitecka L, Ben-Ari Y. Maturation of kainic acid seizure-brain damage syndrome in the rat. III. Postnatal development of kainic acid binding sites in the limbic system. Neuroscience. 1984. 13:1095–1104.


21. Sankar R, Shin DH, Wasterlain CG. GABA metabolism during status epilepticus in the developing rat brain. Brain Res Dev Brain Res. 1997. 98:60–64.


22. Reimold AM, Grusby MJ, Kosaras B, Fries JW, Mori R, Maniwa S, et al. Chondrodysplasia and neurological abnormalities in ATF-2 deficient mice. Nature. 1996. 379:262–265.


23. Kreutz MR, Bien A, Vorwerk CK, Böckers TM, Seidenbecher CI, Tischmeyer W, et al. Co-expression of c-Jun and ATF-2 characterizes the surviving retinal ganglion cells which maintain axonal connections after partial optic nerve injury. Brain Res Mol Brain Res. 1999. 69:232–241.


24. Lee JK, Kang SS, Lee MC. Stress protein expression in kainate-induced experimental temporal lobe epilepsy in rats. J Korean Neurosurg Soc. 1998. 27:1641–1652.
25. Williams MB, Jope RS. Distinctive rat brain immediate early gene responses to seizures induced by lithium plus pilocarpine. Brain Res Mol Brain Res. 1994. 25:80–89.


26. Rikhter TY, Hsu FC, Coulter DA. Gene expression alterations in CA1 area of the rat hippocampus before the onset of epilepsy: A microchip study. 2003. In : AES Proceedings Annual Meeting of the American Epilepsy Society; 2003 Dec 5-10; Boston, Massachusetts. American Epilepsy Society.
27. Kim JH, Jung HY, Roh MS, Ahn YM, Kang UG, Kim YS, et al. Developmental Changes in the activation of signal transduction pathway via JNK in rat hippocampus after kainic acid-induced seizure. J Korean Neuropsychiatr Assoc. 2001. 40:971–980.
28. Behrens A, Sibilia M, Wagner EF. Amino-terminal phosphorylation of c-Jun regulates stress-induced apoptosis and cellular proliferation. Nat Genet. 1999. 21:326–329.


29. Schauwecker PE. Seizure-induced neuronal death is associated with induction of c-Jun N-terminal kinase and is dependent on genetic background. Brain Res. 2000. 884:116–128.


30. Yang DD, Kuan CY, Whitmarsh AJ, Rincón M, Zheng TS, Davis RJ, et al. Absence of excitotoxicity-induced apoptosis in the hippocampus of mice lacking the Jnk3 gene. Nature. 1997. 389:865–870.


31. Lee MC, Rho JL, Kim MK, Woo YJ, Kim JH, Nam SC, et al. c-JUN expression and apoptotic cell death in kainate-induced temporal lobe epilepsy. J Korean Med Sci. 2001. 16:649–656.


32. Estus S, Zaks WJ, Freeman RS, Gruda M, Bravo R, Johnso EM Jr. Altered gene expression in neurons during programmed cell death: identification of c-jun as necessary for neuronal apoptosis. J Cell Biol. 1994. 127(6 Pt 1):1717–1727.

