Abstract
Purpose
Human embryonic stem cells (hESCs) can proliferate for a prolonged period and differentiate into cardiomyocytes in vitro. Recent studies used bone morphogenetic protein 2 (BMP2) to generate cardiomyocytes from hESCs, however, all those studies used early embryoid bodies (EBs) and did not retrieve cardiomyocytes with a high yield. In this study, we treated long-term cultured EBs with BMP2 in order to promote differentiation into cardiomyocytes from hESCs.
Materials and Methods
hESC lines, including SNUhES3 and SNUhES4, were used in this study. Undifferentiated hESC colonies were detached to form EBs and cultured for up to 30 days. These long-term cultured EBs were differentiated into cardiomyocytes in serum-containing media. In our protocol, BMP2 was applied for 5 days after attachment of EBs. Cardiac specific markers, beating of differentiated cells and electron microscopic (EM) ultrastructures were evaluated and analyzed.
Results
Compared to 10-day or 20-day EBs, 30-day EBs showed a higher expression level of cardiac specific markers, Nkx2.5 and α-myosin heavy chain (αMHC). Treatment of BMP2 increased expression of cardiac troponin (cTn) I and α-actinin when evaluated at 20 days after attachment of 30-day EBs. Beating of differentiated cells was observed from 7 to 20 days after attachment. Moreover, EM findings demonstrated fine structures such as Z bands in these differentiated cardiomyocytes. These long-term cultured EBs yielded cardiomyocytes with an efficiency of as high as 73.6% when assessed by FACS.
Human embryonic stem cells, derived from inner cell mass (ICM) of preimplantation embryos, can proliferate for a prolonged period in vitro1,2 and can differentiate into various cell types under suitable environment. Therefore, hESCs are considered as a candidate cell source of cell-based therapies for heart diseases. Since 2001, there have been numerous studies of hESC-derived cardiomyocytes,3-10 and these studies used spontaneous differentiation,3,4,6,7,10 low-serum culture condition8,9 and co-culture with endoderm-like cells.5 However, practical methods using specific signaling molecules that are known to be efficient in differentiation into cardiomyocytes from hESCs are still limited.
Development of uncommitted mesodermal precardiac cells to early cardiomyocytes is regulated by stimulatory signals that are secreted from anterior primitive endoderm.11 Bone morphogenetic proteins (BMPs) signaling is main signaling pathway regulating the cardiomyogenesis. Among BMPs, BMP2 is known to play a crucial role in the induction of heart formation of vertebrate embryos.12-14
In hESCs, BMP2 is known to be as an inducer for mesoderm or cardiac differentiation. Tomescot et al. showed that BMP2 treatment turned on expression of cardiac related genes in hESCs.15 In addition, Pal et al. demonstrated that BMP2 with low serum concentration could induce differentiation into cardiomyocytes in many hESC lines.16 These studies used short-term cultured EBs for differentiation into cardiomyocytes. However, the yield of differentiation was reported to be around 30%.16
In this study, we used long-term cultured EBs from hESC lines, SNUhES3 and SNUhES4, to generate cardiomyocytes with BMP2 treatment in order to enhance in vitro differentiation efficiency.
Human embryonic stem cells were cultured according to previously described methods.18 Undifferentiated hESCs were maintained on mitomycin C-(Sigma, St. Louis, MO, USA) treated STO (ATCC, Manassas, VA, USA) feeder layer. Undifferentiated hESC colonies were dissected and replated onto a fresh feeder layer every 7 days. DMEM/F12 (Invitrogen, Carlsbad, CA, USA), supplemented with 20% knockout serum replacement (KO-SR; Invitrogen), 1% nonessential amino acids (Invitrogen), 0.1 mM β-mercaptoethanol (Sigma), 0.4 ng/mL basic fibroblast growth factor (bFGF; Invitrogen), 50 U/mL penicillin (Invitrogen) and 50 µg/mL streptomycin (Invitrogen), was used as hESC culture medium.
To form EBs, undifferentiated hESC colonies were cultured for 5 days. Day 5 hESC colonies were incubated with 2 mg/mL collagenase type IV (Invitrogen) for 30 minutes at 37℃ to detach colonies from dish bottom. Detached colonies were transferred to bacterial dish and cultured for 10, 20, and 30 days in suspension. hESC culture medium, excluding bFGF was used as EB medium. Medium was changed every other day.
After culture for 10, 20 and 30 days in suspension, EBs were transferred to gelatin pre-coated tissue culture dishes. Knockout DMEM (Invitrogen), supplemented with 20% fetal bovine serum (FBS; HyClone, Logan, UT, USA), was used as a differentiation medium for further differentiation, and medium was changed every other day. Ten, 20 and 40 ng/mL BMP2 (R & D Systems, Minneapolis, MN, USA) was treated for 5 days after plating to promote differentiation.
Cells were washed with phosphate-buffered saline (PBS; Invitrogen) and fixed with 4% paraformaldehyde (PFA; Sigma) for 15 minutes at room temperature (RT). After washing with PBS, cells were incubated overnight with 3% bovine serum albumin (BSA; Sigma) solution, including 0.1% Triton X100 (Sigma), at 4℃ to prevent nonspecific reaction. After washing with PBS, mouse anti-human Nkx2.5 (R & D Systems), goat anti-Nkx2.5 (Santa Cruz Biotechnology, Santa Cruz, CA, USA), mouse anti-human cardiac Troponin I (cTn I; Chemicon, Billerica, MA, USA), goat anti-α-myosin heavy chain (αMHC; Santa Cruz Biotechnology), goat anti-human α-actinin (Santa Cruz) and mouse anti-smooth muscle actin (SMA; Chemicon) antibodies were added overnight at 4℃ and then washed 3 times with PBST (PBS with 0.05% Tween 20). Each Alexa Fluor 488-labeled donkey anti-mouse IgG, Alexa Fluor 488-labeled donkey anti-goat IgG, Alexa Fluor 594-labeled donkey anti-mouse IgG and Alexa Fluor 594-labeled donkey anti-goat IgG (all from Molecular Probes, Carlsbad, CA, USA) antibodies were applied for 60 minutes at RT and washed three times with PBST. 4'-6-diamidino-2-phenylindole (DAPI; Molecular Probes) solution was added and incubated for 30 minutes at RT for staining of nuclei. Stained cells were analyzed using a confocal laser microscopy system (BioRad, Hercules, CA, USA).
Total RNA was isolated from EBs and differentiated cells using RNeasy mini kit (Qiagen, Valencia, CA, USA). cDNA was synthesized from 1 µg of total RNA using Superscript II first-strand synthesis system (Invitrogen). Quantitative PCR was performed in Rotor-Gene 3000 (Corbett Life Science, Sydney, Australia) using QuantiTect SYBR green PCR kit (Qiagen). Primers used for the reactions are listed in Table 1. CT was calculated under default settings of Rotor-gene 6 program (Corbett Life Science). Relative gene expression was normalized to GAPDH expression.
Cells were fixed with 2.5% glutaraldehyde (Sigma) for 20 minutes at RT. Cells were then postfixed with 1% osmium tetroxide (OsO4; Sigma) in 0.04 M phosphate buffer for 10 minutes at 4℃, supplemented with 0.14 M sucrose. Following serial dehydration in ethanol and infiltration with epoxy resin, cells were transferred to beam capsules for polymerization. The capsules were separated from the polymerized resin with a razor blade. Embedded cells in hardened blocks were observed under an optical microscope for ultra-thin sectioning. Subsequently, ultra-thin sections were obtained using an ultramicrotome (Sorvall MT 6000). Cells were then observed under a transmission electron microscope (JEM-1400; JEOL Ltd.) at 50 kV.
Cells were washed with PBS and treated with 0.25% trypsin-EDTA (Invitrogen) for 40 minutes at 37℃ to dissociate them into single cells. After washing with PBS, 3% BSA solution, including 0.05% Triton X100, was added for 20 minutes at RT. Mouse anti-human Nkx2.5 (R & D Systems) and goat anti-αMHC (Santa Cruz Biotechnology) antibodies were applied for 60 minutes at RT and then washed 3 times with PBST. Alexa Fluor 488-labeled donkey anti-goat IgG and Alexa Fluor 594-labeled donkey anti-mouse IgG (all from Molecular Probes) were applied for 60 minutes at RT and washed 3 times with PBST. Cells were then analyzed using BD FACS Calibur System™ (BD Sciences, San Jose, CA, USA).
Schematic process of differentiation into cardiac cells is presented in Fig. 1. Briefly, EBs were formed from hESCs at day 6, differentiated in suspension for 30 days and attached for further differentiation. From 7 days after attachment, beating clusters started to appear and lasted for as long as 30 days. We first examined the expressions of alkaline phosphatase, Oct4, SSEA4, Tra-1-60 and Tra-1-81 in undifferentiated hESCs. All pluripotent hESC specific markers were highly expressed in undifferentiated hESC colonies (Fig. 2). Expressions of cardiac specific markers were evaluated in undifferentiated hESC colonies to test specificity of each marker. Cardiac specific markers, cTn I and αMHC, were not expressed in undifferentiated hESCs. Only nuclei were stained with DAPI (Fig. 2). These results indicated that hESCs maintained their pluripotent characteristics stably in vitro.
Undifferentiated hESC colonies of SNUhES3 and SNUhES4 were detached to form EBs. These EBs were cultured in suspension for 10 days, 20 days, and 30 days to induce differentiation into cardiomyocytes, and mRNA expression in suspension cultured EBs was then evaluated. Expression of mesoderm lineage markers, such as Brachyury and cardiac specific markers, Nkx2.5 and αMHC, was evaluated by Q-PCR (Fig. 3C). Brachyury expression was the highest at day 30. Nkx2.5 and αMHC were also highly expressed in day 30 EBs. Based on these results, 30-day EBs were used for cardiomyocyte differentiation (Fig. 3A-a). Reattached EBs differentiated into cardiac lineage precursor cells (Fig. 3A-b). Immunostaining showed that cardiac specific transcription factors, such as Nkx2.5, proteins cTn I and αMHC, were expressed in differentiated cells (Fig. 3B). Although differentiated cells expressed specific markers, however, their expression levels were not high in both cell lines. Expression of cTn I in differentiated cells was restricted to small area.
Various concentrations of BMP2, 10, 20 and 40 ng/mL, were administered to promote differentiation into cardiomyocytes for 5 days after attachment. Among the concentrations, 10 ng/mL was most effective for inducing differentiation.
Thirty-day EBs (Fig. 4A-a and e) were reattached for further differentiation. Two or three days after plating, differentiating cardiac potential cells started to appear in reattached EBs (Fig. 4A-b and f), and myocyte-like cells were also observed at the periphery of reattached EBs (Fig. 4A-f). Contractile cluster-like cells were distinguishable 5 days postplating (Fig. 4A-c) and became distinct as differentiation progressed (Fig. 4A-g). Contractile clusters were observed from 7 days to 20 days after plating of EBs. Clusters showed regularity in beating and the beating lasted more than 30 days in vitro (Fig. 4A-d and h, Supplementary Fig. 1).
Expressions of cardiac specific markers were significantly increased by treatment with BMP2. Immunostaining demonstrated the expressions of transcription factor Nkx2.5, structural protein α MHC (Fig. 4B, upper panel) and cardiac specific protein cTn I (Fig. 4B, middle panel). Alpha actinin (α-actinin) was also detected in BMP2-treated cells (Fig. 4B, bottom panel). Intriguingly, when myocyte-like structures located around the clusters were evaluated, these cells were positively stained with SMA (Fig. 4C).
Q-PCR analysis showed that the expression of Nkx2.5 and αMHC was increased in BMP2-treated group more than untreated group. Especially, BMP2 treatment strikingly increased the expression of structural protein αMHC (Fig. 4D).
Ultrastructures of hESC-derived cardiomyocytes were observed using transmission electron microscopy. Differentiated cells were analyzed to confirm the possession of Z bands. Z bodies, which are precursors of Z bands, and unorganized sarcomeric structure were abundant in differentiated cells (Fig. 4E-a). Differentiated cells from BMP2-treated group showed many unorganized (Fig. 4E-b) as well as organized (Fig. 4E-c and d) Z bands. This ultrastructural analysis showed various stages of Z band development even in a single cell, suggesting that high proportion of cells was in progress for differentiation.
To find out the efficiency, the proportion of cardiac specific marker-positive cells was evaluated by FACS analysis. Proportion of cardiac lineage specific cells was significantly higher than those in untreated differentiated cells (data not shown). As high as 73.6% of cells in BMP2-treated group (range, 10.8 - 73.6%) were doubly positive for Nkx2.5 and α MHC (Fig. 5). These results clearly show that BMP2 could be an effective inducing factor for differentiation into cardiomyocytes, and the use of long-term cultured EBs may enhance the yield.
To our best knowledge, this is the first study that used long-term cultured EBs in differentiation into cardiomyocytes from hESCs. When these long-term cultured EBs differentiated by treatment with BMP2, their differentiation efficiency was as high as 73.6%. This figure is higher than that in previous reports.16 This high yield of differentiation demonstrated the use of long-term cultured EBs with BMP2 treatment may enhance differentiation into cardiomyocytes in the cell lines used. In addition, our protocol generated healthier, vigorous, contracting clusters, and the beating lasted more than 30 days in vitro. We also demonstrated that differentiated cells from long-term cultured EBs with BMP2 possessed many unorganized and organized Z bands. These findings suggest that differentiation was ongoing from precursors of Z bands to organized mature forms.
To date, many methods have been utilized for generation of cardiomyocytes from hESCs, such as spontaneous differentiation,3,4,6,7 co-culture with END-2 cell line5,8,9 and serum-free or low-serum culture condition.8-10 As for cardiac lineage inducing agents, 5'-aza-deoxycytidine and BMP4 were among a few molecules that are known to work in differentiation into cardiomyocytes from hESCs.4 BMPs are well-known morphogen that contribute to differentiation into cardiac lineage; e.g., BMP signaling is crucial in mesodermal induction and cardiac formation in vertebrate.14 Tomescot et al. used BMP2 to induce differentiation into cardiac committed cells that were used in a rat infarct model,15 and Pal et al. showed that BMP2 is another effective molecule for in vitro differentiation into cardiomyocytes from hESCs in low serum condition.16 However, these researchers used short-term cultured EBs, and one of these reports retrieved a yield of about 30%.16
In this study, we tried to generate cardiomyocytes from our established hESC lines, SNUhES32 and SNUhES4.17 When treated with BMP2, both cell lines showed comparable differentiation into cardiomyocytes. To determine the optimal time point of differentiation induction initiation, EBs were cultured in suspension for 10 days, 20 days and 30 days. Brachyury, which is known to be expressed at precursor stage,19 and cardiac specific markers, Nkx2.5 and αMHC, were highly expressed in 30-day EBs. Accordingly, we were able to obtain a high yield by using this stage's EBs.
Interestingly enough, each cluster among many hESC-derived contractile clusters showed different frequencies of contraction (23 - 62/minutes). In addition, contractility of these hESC-derived cardiac cells was found to be sensitive to temperature: contractility was significantly reduced when exposed to lower temperature and beat rhythm was recovered when temperature rose.
At the periphery of contractile regions, elongated myocyte-like cells were observed. These cells were connected to contractile clusters and contracted when the cluster beat (Fig. 4A-c and g). These cells were identified as smooth muscle cells. Smooth muscle cells are derived from common progenitor with cardiomyocytes and known to contribute to formation of the mature heart.19 Our findings suggested that differentiation from hESCs into cardiomyocytes is accompanied by differentiation of smooth muscle cells, which were abundant at the peripheral region of clusters.
We used serum-containing media for the induction of differentiation. Reduced concentration of serum did not effectively generate cardiomyocytes in our cell lines (data not shown). Role of serum in cardiomyocytes differentiation is still controversial, although many studies adopted serum-free or low-serum culture conditions in cardiac differentiation.8,10 Therefore, the effects of serum concentration should be the subject for further studies.
In conclusion, we demonstrated that the use of long-term cultured EBs with BMP2 treatment may enhance differentiation into cardiomyocytes from 2 hESC lines, confirmed by increased expression of cardiac specific markers, and observation of contractile clusters and Z bands in differentiated cells.
Figures and Tables
Fig. 1
A schematic presentation of in vitro differentiation strategy for induction of cardiomyocytes from human embryonic stem cells (hESCs), using long-term cultured embryoid bodies (EBs) treated with BMP2.

Fig. 2
Expressions of pluripotent hESC- and cardiac specific markers in hESCs. (upper panel) Alkaline phosphatase (AP), Oct4 and SSEA4 were highly expressed in undifferentiated hESCs, Bars: 500 µm. Lower panel) Tra-1-60 and Tra-1-81 were also highly expressed in undifferentiated hESCs. However, cardiac troponin (cTn) I and α-myosin heavy chain (αMHC), cardiac specific markers, were not expressed in undifferentiated hESCs. Merged picture showed only nuclei stained with DAPI. Bars: 200 µm.
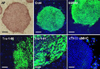
Fig. 3
Evaluation of mesoderm- and cardiac-specific markers in long-term cultured EBs. (A) Morphology of a 30-day EB (a) in suspension and (b) after attachment. Bars: 500 µm. (B) Expressions of cardiac lineage markers in spontaneously differentiated cells from SNUhES3 (upper panel) and SNUhES4 (lower panel). At 20 days after plating, differentiated cells from both hESC lines were positively stained with cardiomyocyte-specific markers, Nkx2.5, αMHC and cTn I, however, their expression was not high and showed a scattered pattern. Bars: 200 µm. (C) Relative expression of mesoderm- and cardiac-lineage markers in long-term cultured EBs from SNUhES3 by Q-PCR. 10-, 20- and 30-day EBs were compared in terms of expression of Brachyury, Nkx2.5 and αMHC, which showed the highest level at day 30 for all three markers.
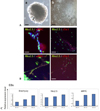
Fig. 4
Evaluation of mesoderm- and cardiac specific markers in cardiomyocytes differentiated from BMP2-treated cells (SNUhES3). (A) Morphologies of long-term cultured embryoid bodies (EBs) and cardiomyocytes differentiated from BMP2-treated cells. Phase contrast microscopic appearance of 30-day EBs (a and e) in suspension and (b and f) after attachment, of hESC-derived cardiomyocytes 4 days (c) and 11 days after attachment (g), and contractile clusters derived from BMP2-treated differentiated cells (d and h) Bar: a-g, 500 µm; (h) 200 µm. (B) Expression of cardiac specific markers in differentiated cardiomyocytes. Differentiated cells were evaluated 20 days after plating and nuclei were stained with DAPI. Top panel, expression of transcription factor Nkx2.5 and protein αMHC; Middle panel, expression of Nkx2.5 and cTn I; Bottom panel, expression of Nkx2.5 and α-actinin (arrow of Fig 4A-h). Bars: 100 µm. (C) Expression of Nkx2.5 and smooth muscle actin (SMA), which is abundant in peripheral cells of cardiomyocyte cluster (yellow box of Fig. 4A-g). Bars: 100 µm. (D) Relative expression of cardiac lineage markers evaluated by Q-PCR. Differentiated cells showed significant expression of Brachyury, Nkx2.5 and αMHC. At 10 ng/mL of BMP2, αMHC expression was the highest, and beating cells were observed in this group (Suppl. Fig. 1). (E) Ultrastructures of differentiated cells observed by transmission electron microscopy (TEM). Red arrows, (a) Z bodies (dense spots), which are the precursors of Z bands. (b) unorganized Z bands. Many unorganized, unconnected bands were observed in many parts. (c and d) organized Z bands. Magnification, a, × 12K; b, × 30K; c, × 60K; d, × 100K.
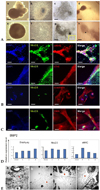
Fig. 5
FACS analysis of cardiac lineage markers, Nkx2.5 and αMHC, in BMP2-induced differentiated cells from plated EBs. As high as 73.6% of differentiated cells were positive for both cardiac markers, evaluated 20 days after plating (Suppl. Fig. 1). Moving image of hESC-derived cardiomyocytes from long-term cultured EBs treated with BMP2. Images were recorded under phase contrast microscope (× 200), and contractile region showed regular contractions with a frequency of 1 beat/second.
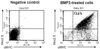
References
1. Thomson JA, Itskovitz-Eldor J, Shapiro SS, Waknitz MA, Swiergiel JJ, Marshall VS, et al. Embryonic stem cell lines derived from human blastocysts. Science. 1998. 282:1145–1147.


2. Oh SK, Kim HS, Ahn HJ, Seol HW, Kim YY, Park YB, et al. Derivation and characterization of new human embryonic stem cell lines: SNUhES1, SNUhES2 and SNUhES3. Stem Cells. 2005. 23:211–219.


3. Kehat I, Kenyagin-Karsenti D, Snir M, Segev H, Amit M, Gepstein A, et al. Human embryonic stem cells can differentiate into myocytes with structural and functional properties of cardiomyocytes. J Clin Invest. 2001. 108:407–414.


4. Xu C, Police S, Rao N, Carpenter MK. Characterization and enrichment of cardiomyocytes derived from human embryonic stem cells. Circ Res. 2002. 91:501–508.


5. Mummery C, Ward-van Oostwaard D, Doevendans P, Spijker R, van den Brink S, Hassink R, et al. Differentiation of human embryonic stem cells to cardiomyocytes: role of coculture with visceral endoderm-like cells. Circulation. 2003. 107:2733–2740.


6. He JQ, Ma Y, Lee Y, Thomson JA, Kamp TJ. Human embryonic stem cells develop into multiple types of cardiac myocytes: action potential characterization. Circ Res. 2003. 93:32–39.
7. Kehat I, Khimovich L, Caspi O, Gepstein A, Shofti R, Arbel G, et al. Electromechanical integration of cardiomyocytes derived from human embryonic stem cells. Nat Biotechnol. 2004. 22:1282–1289.


8. Passier R, Oostwaard DW, Snapper J, Kloots J, Hassink R, Kuijk E, et al. Increased cardiomyocyte differentiation from human embryonic stem cells in serum-free cultures. Stem Cells. 2005. 23:772–780.
9. Beqqali A, Kloots J, Ward-van Oostwaard D, Mummery C, Passier R. Genome-wide transcriptional profiling of human embryonic stem cells differentiating to cardiomyocytes. Stem Cells. 2006. 24:1956–1967.


10. Bettiol E, Sartiani L, Chicha L, Krause KH, Cerbai E, Jaconi ME. Fetal bovine serum enables cardiac differentiation of human embryonic stem cells. Differentiation. 2007. 75:669–681.


11. Schlange T, Andrée B, Arnold HH, Brand T. BMP2 is required for early heart development during a distinct time period. Mech Dev. 2000. 91:259–270.


12. Yuasa S, Itabashi Y, Koshimizu U, Tanaka T, Sugimura K, Kinoshita M, et al. Transient inhibition of BMP signaling by Noggin induces cardiomyocyte differentiation of mouse embryonic stem cells. Nature Biotechnol. 2005. 23:607–611.
13. Antin PB, Taylor RG, Yatskievych T. Precardiac mesoderm is specified during gastrulation in quail. Dev Dyn. 1994. 200:144–154.


14. Schultheiss TM, Burch JB, Lassar AB. A role for bone morphogenetic proteins in the induction of cardiac myogenesis. Genes Dev. 1997. 11:451–462.


15. Tomescot A, Leschik J, Bellamy V, Dubois G, Messas E, Bruneval P, et al. Differentiation in vivo of cardiac committed human embryonic stem cells in postmyocardial infarcted rats. Stem Cells. 2007. 25:2200–2205.


16. Pal R, Khanna A. Similar pattern in cardiac differentiation of human embryonic stem cell lines, BG01V and ReiCell® hES1, under low serum concentration supplemented with bone morphogenetic protein-2. Differentiation. 2007. 75:112–122.


17. Kwon YD, Oh SK, Kim HS, Ku SY, Kim SH, Choi YM, et al. Cellular manipulation of human embryonic stem cells by TAT-PDX1 protein transduction. Mol Ther. 2005. 12:28–32.

