Abstract
Purpose
We have used a genetically attenuated adenoviral vector which expresses HSVtk to assess the possible additive role of suicidal gene therapy for enhanced oncolytic effect of the virus. Expression of TK was measured using a radiotracer-based molecular counting and imaging system.
Materials and Methods
Replication-competent recombinant adenoviral vector (Ad-ΔE1B19/55) was used in this study, whereas replication-incompetent adenovirus (Ad-ΔE1A) was generated as a control. Both Ad-ΔE1B19/55-TK and Ad-ΔE1A-TK comprise the HSVtk gene inserted into the E3 region of the viruses. YCC-2 cells were infected with the viruses and incubated with 2'-deoxy-2'-fluoro-β-D-arabinofuranosyl-5-iodouracil (I-131 FIAU) to measure amount of radioactivity. The cytotoxicity of the viruses was determined, and gamma ray imaging of HSVtk gene was performed. MTT assay was also performed after GCV treatment.
Results
On gamma counter-analyses, counts/minute (cpm)/µg of protein showed MOIs dependency with ΔE1B19/55-TK infection. On MTT assay, Ad-ΔE1B19/55-TK led to more efficient cell killing than Ad-ΔE1A-TK. On plate imaging by gamma camera, both Ad-ΔE1B19/55-TK and Ad-ΔE1A-TK infected cells showed increased I-131 FIAU uptake in a MOI dependent pattern, and with GCV treatment, cell viability of ΔE1B19/55-TK infection was remarkably reduced compared to that of Ad-ΔE1A-TK infection.
Conclusion
Replicating Ad-ΔE1B19/55-TK showed more efficient TK expression even in the presence of higher-cancer cell killing effects compared to non-replicating Ad-ΔE1A-TK. Therefore, GCV treatment still possessed an additive role to oncolytic effect of Ad-ΔE1B19/55-TK. The expression of TK by oncolytic viruses could rapidly be screened using a radiotracer-based counting and imaging technique.
Adenoviral vectors have great gene transfer efficiency of in both in vitro and in vivo settings, and have been used for a variety of gene therapy applications.1-3 Of the adenoviral vectors, replication-incompetent adenovirus has demonstrated promises as anticancer agents delivering various transgenes to cancer cells in preclinical studies. However, studies using replication-incompetent adenovirus have not shown benefits in clinical patients as in preclinical models.4 One of the major limitations in replication-incompetent adenovirus is that anticancer therapeutic effects of the viral vectors are only limited primarily to those infected cells within solid tumors. Therefore, many genetically modified replication-competent adenoviruses have developed to selectively replicate in cancer cells. The life cycle of replication-competent adenoviruses results in lysis of the infected cells, spread into adjacent cells for further viral multiplication, and improving treatment efficacy.
In spite of safety and feasibility of treatment, the low potency of replication-competent selective adenoviral vectors has been problematic,5 and the incorporation of therapeutic transgenes such as HSVtk (herpes simplex-1 thymidine kinase) has been developed as an alternative to improve anticancer efficacy in cancer gene therapy.6 HSVtk is a well-known enzyme that converts prodrug ganciclovir (GCV) into a cytotoxic metabolite. The oncolysis caused by a replicating virus and suicide/prodrug gene therapy that uses HSVtk gene to sensitize tumors to ganciclovir (GCV) have been reported to compliment each other in increasing the potency of anticancer effects and treatment outcome. In contrast to some promising results, several studies have shown no additive effect of GCV in the context of highly potent oncolysis mediated by highly effective infectivity-enhanced viruses.7 The effect of GCV seems to be different depending on the potency of oncolysis in differently modified vectors. Rapid screening system to assess the amount of transgene expression by oncolytic viruses can be useful to decide the use or timing of GCV treatment for newly designed vectors.
In this study, we have used a genetically attenuated adenoviral vector which expresses HSVtk to assess the possible additive role of suicidal gene therapy to the enhanced oncolytic effect of the genetically engineered virus. The expression of transgene was qualitatively and quantitatively measured using a radiolabeled substrate which can be used both in vitro and in vivo for preclinical and clinical research.
A human-transformed embryonic kidney cell line expressing the adenoviral E1 region (HEK293) and human gastric adenocarcinoma cell line (YCC-2) were used in this study. The cells were maintained in Dulbecco's modified Eagle's medium (DMEM; GIBCO-BRL, Grand Island, NY, USA) supplemented with 10% fetal bovine serum (GIBCO-BRL) and penicillin-streptomycin (100 IU/mL) in humidified atmosphere of 95% air and 5% CO2 at 37℃.
A replication-competent recombinant adenoviral vector (Ad-ΔE1B19/55) containing normal E1A but deleting E1B19 kD and E1B-55 kD was constructed. A replication-incompetent adenovirus (Ad-ΔE1A) deleting the whole E1 region was also generated as a control. Both Ad-ΔE1B19/55-TK and Ad-ΔE1A-TK comprise the HSVtk gene inserted into the E3 region of Ad-ΔE1B19/55 and Ad-ΔE1A, respectively. The recombinant adenoviruses were propagated in 293 cells and purified by CsCl equilibrium centrifugation. Purified virion preparations were dialyzed against 10 mM phosphate-buffered saline (PBS)- 4% sucrose and finally stored at - 80℃. The number of viral particles was calculated by measuring optical density at 260 nm using a conversion factor of 1.1 × 1012 viral particles/mL/absorbance unit. The multiplicity of infection (MOI) was calculated from viral particle numbers.
Generated adenoviruses were lysed with proteinase K, and then viral DNA was isolated with a genomic isolation kit (Qiagene, Santa Clarita, CA, USA). PCR was accomplished using 2 kinds of E1- and HSVtk-specific primer sets, and the expected sizes of PCR products were 479 and 700 bp, respectively. The PCR product fragments were separated by 1% agarose gel electrophoresis and visualized by UV fluorescence.
The 2'-deoxy-2'-fluoro-β-D-arabinofuranosyl-5-iodouracil (FIAU) and the radiolabeling precursor, 2'-deoxy-2'-fluoro-β-D-arabinofuranosyluracil (FAU) were obtained from Futurechem (Incheon, Korea). FAU was radioiodinated with I-131 and purified by HPLC. After vacuum dry, I-131 FIAU was further tested for purity by HPLC analyses and a purity of > 95% was assured in this study.
All experiments were conducted in triplicate in this study. Expression of TK resulting from adenoviral infection was evaluated in YCC-2 cells. The cells were seeded at 5 × 104 cells/well on 24-well plates. Twenty four hours later, the cells were infected with a range of titers (1 MOI to 40 MOI, in triplicate) of Ad-ΔE1B19/55-TK or Ad-ΔE1A-TK for 24 to 72 hours. After washing with PBS, the cells were incubated with I-131 FIAU (4µCi/well) for 4 hours. In additional wells, I-131 FIAU was added without infection of virus or only cells were incubated without virus and radiotracer.
After 4 hours of incubation, the cells were washed twice with PBS, and 200 µL of lysis buffer was added to each cell for 1 hour. And the lysed cells were harvested to measure the amount of radioactivity by gamma counter (Wallac 148 wizrad 3, Perkin Elmer, Finland). In addition, the amount of protein content was also measured by BCA protein assay to normalize radioactivity to protein content in the wells.
The cytotoxicity of adenoviruses was determined by MTT (Sigma, St. Louis, MO, USA) assay which measures the conversion of the tetrazolium salt MTT (3-(4,5-dimethylthiazolyl-2)-2,5-diphenyltetrazolium bromide) to formazan. YCC-2 cells were seeded at 5 × 104 cells per well on 24-well plates. After 24 hours of plating, the cells were infected with a range of titers (1 MOI to 40 MOI, in triplicate) of Ad-ΔE1B19/55-TK or Ad-ΔE1A-TK for 24 to 72 hours. At the indicated times postinfection, 200 µL of MTT in PBS (1 mg/mL) was added to each well and incubated for 4 hours at 37℃. Then, the supernatant was discarded, and the precipitate was dissolved in 200 µL of dimethyl sulfoxide (DMSO) to dissolve MTT formazan crystals. Finally, plates were read on a microplate reader at 540 nm.
For gamma ray imaging of HSVtk gene expression, YCC-2 cells were seeded on 24-well plates (1 × 105 cells/well) and cultured overnight. Then, the cells were infected with Ad-ΔE1B19/55-TK or Ad-ΔE1A-TK at MOIs of 1, 10, and 40 for 48 hours. After washing with PBS, the cells were then incubated with I-131 FIAU (4 µCi/well) for 4 hours. In additional triplicate wells, I-131 FIAU was added without infection of virus. Following incubation, the plates were washed 3 times with PBS and imaged with gamma camera equipped with a high-energy, parallel-hole collimator using a 512 × 512 matrix size for 30 minutes. After imaging, the cells were lysed and harvested to measure the amount of radioactivity by gamma counter. The counts in each well were normalized to protein content in the well.
GCV was purchased from Roche (Espoo, Finland) and 50 mg/mL stock solution of GCV in sterilized water was diluted in growth media before use. YCC-2 cells were seeded on 24-well plates at 1 × 105 cells/well 24 hours prior to infection. The cells were infected with a range of titers (1 MOI to 40 MOI, in triplicate) of Ad-ΔE1B19/55-TK or Ad-ΔE1A-TK for 48 hours. At 48 hours postinfection, GCV was added at final concentrations of 100 µg/mL. The cells were incubated at 37℃ for additional 3 days, and MTT assay was performed to measure the degree of cell viability.
To generate adenoviruses expressing HSVtk gene, we generated E1-deleted replication-incompetent Ad (Ad-ΔE1A-TK) and replication competent Ad (Ad-ΔE1B19/55-TK). To verify each adenovirus, viral DNA was isolated and subsequently used for PCR amplification with E1- and TK-specific primer sets (Fig. 1). As expected with the E1A-specific primer set, PCR generated a DNA fragment of 479 bp from replication competent Ads (Ad-ΔE1B19/55 and Ad-ΔE1B19/55-TK) but replication incompetent Ads (Ad-ΔE1A and Ad-ΔE1A-TK) were not detected. Also, with the TK-specific primer set, PCR generated a DNA fragment of 700 bp from adenoviruses expressing HSVtk, Ad-ΔE1-TK and Ad-ΔE1B19/55-TK. This result indicated that replication competent Ads (Ad-ΔE1B19/55 and Ad-ΔE1B19/55-TK) express E1A and Ad-ΔE1-TK and Ad-ΔE1B19/55-TK express effectively HSVtk gene.
YCC-2 cells were infected with a range of titers (1 MOI to 40 MOI, in triplicate) of Ad-ΔE1B19/55-TK or Ad-ΔE1A-TK for 24 to 72 hours to measure the level of TK expression using I-131 FIAU (Fig. 2). On gamma counter analyses of lysed cells, cpm/µg of protein showed MOIs dependency with a linear increase from 1 to 40 MOI at all time points with ΔE1B19/55-TK infection. However, with Ad-ΔE1A-TK infection, cpm/µg of protein were unchanged over MOIs after 24 hours of infection and slightly increased after 48 and 72 hours of infection with 40 MOI. Cpm/µg of protein with ΔE1B19/55-TK infection tended to be higher than those with Ad-ΔE1A-TK infection at all time points. In other words, higher amounts of TK were produced from replicating than non-replicating viruses.
As an indicator of cell viability, we performed MTT assay after Ad-ΔE1B19/55-TK or Ad-ΔE1A-TK infection (Fig. 3). After 24 hours of infection, both Ad-ΔE1B19/55-TK and Ad-ΔE1A-TK showed no remarkable changes of cell viability over the ranges of MOIs. However, after 48 hours of infection, cell viability was slightly reduced over MOIs only with Ad-ΔE1B19/55-TK infection. After 72 hours of infection, cell viability showed even more MOI dependency with a linear decrease from 1 to 40 MOI with Ad-ΔE1B19/55-TK, whereas it was slightly decreased at 40 MOI with Ad-ΔE1A-TK infection. Ad-ΔE1B19/55-TK led to more efficient cell killing than Ad-ΔE1A-TK after 48 and 72 hours of infection, and the amount of viruses needed was lower with Ad-ΔE1B19/55-TK for the same cytotoxicity.
The cells were infected with Ad-ΔE1B19/55-TK or Ad-ΔE1A-TK at MOIs of 1, 10, and 40 for 48 hours for gamma ray imaging of TK expression. The incubation time of 48 hours was selected to allow the virus to replicate and produce enough tansgene products, but did not yet show remarkable cell killing effect. On plate imaging by gamma camera, both Ad-ΔE1B19/55-TK and Ad-ΔE1A-TK infected cells showed increased I-131 FIAU uptake in a MOI dependent pattern (Fig. 4A). At 1 MOI, there was similar I-131 FIAU uptake between the two viruses. At 10 and 40 MOIs, uptakes of I-131 FIAU were higher with Ad-ΔE1B19/55-TK than those with Ad-ΔE1A-TK. The differences in uptakes of I-131 FIAU between the 2 viral infections were larger with higher viral titers. In this study, the quantitative results on gamma counting for I-131 FIAU were in line with the results on plate imaging (Fig. 4B). Although there was no remarkable I-131 FIAU uptake in cells without viruses and I-131 FIAU, cells treated only with I-131 FIAU showed mild uptake.
To evaluate if the TK/GCV system could improve the efficacy of treatment in vitro, YCC-2 cells were infected with Ad-ΔE1B19/55-TK or Ad-ΔE1A-TK, and exposed to GCV for 3 days at 48 hours postinfection (Fig. 5). Without GCV treatment, cell viability was slightly reduced only with Ad-ΔE1B19/55-TK infection at higher MOIs. With GCV treatment, cell viability of ΔE1B19/55-TK infection was remarkably reduced compared to that of Ad-ΔE1A-TK infection. The difference in enhanced cell killing effect by the combination of GCV treatment between the two viruses was prominent at a viral titer of 5 or more MOIs. When GCV was given 48 hours postinfection with 40 MOIs of replicating viruses, virus alone resulted in about 80% of cell viability, whereas combination treatment decreased the amount of living cells to less than 40%.
The 2 major genes in the adenovirus E1B region, E1B 55 kDa and E1B 19 kDa, have been used as the targets of modification in genetically attenuated replicating adenoviruses.8 E1B 55 kDa inhibits p53 function and E1A induces apoptosis during viral replication. Therefore, the deletion of E1B 55 kDa gene in a vector system is beneficial for tumor-selective replication and specific cytotoxicity against p53 mutated cancer cells.9,10 However, it is known that E1B 55 kDa deleted adenoviruses (Ad-ΔE1B55) show reduced cytopathic effect, presumably due to the important role of E1B 55 kDa in productive viral replication through cytoplasmic translocation and accumulation of adenoviral mRNA.11,12 E1B 19 kDa gene, a homologue of bcl-2 related apoptosis inhibitors, has been a promising target of deletion to overcome the limited cytotoxicity of Ad-ΔE1B55. In our previous report, adenoviral vectors with the deletions of both E1B 19 kDa and E1B 55 kDa (Ad-ΔE1B19/55) resulted in higher viral release and greater cytopathic effect than Ad-ΔE1B55 in most cancer cell lines.8
In addition to increased anticancer cell killing effect of Ad-ΔE1B19/55, these replication-competent adenoviruses may have another potential advantage of a high capacity of transgene expression.13 Therefore, the incorporation of different therapeutic transgenes has been tried to increase therapeutic potency of replicating adenoviruses for cancer gene therapy.4,6,14 However, the usefulness of transgenes-inserted replicating adenoviruses seems to be uncertain, depending on the effects of transgenes on productive viral replication. Constitutive expression of transgenes may cause premature cellular toxicity and impairment of viral replication, confounding the anticancer effect of oncolysis.15 The retardation of viral replication was also reported due to transgene production competing for the cellular resources needed for viral amplification as well as the impact of larger genome size on speed of viral replication.7 Furthermore, the efficacy of transgene targeting prodrug treatment combined with oncolysis by replicating adenoviruses seems controversial at least in some replicating adenoviruses.16 Therefore, the use of effective screening system is needed in assessing the feasibility of new transgene-incorporated replicating viral vectors in prodrug combination therapy for cancer.
In this study, we have used quantitative molecular imaging technique using gamma ray detection and imaging system to evaluate the usefulness of replicating adenoviruses in combination with GCV for cancer gene therapy. The utilization of radiolabled substrates to image transgene products has been established as a high capacity screening method of gene transfer to cancer cells growing in vitro.17 This technique has also been directly adapted to animal models and human beings for pre-clinical and clinical trials of gene therapy.18,19 Based on our results, the amount of transgene product represented as cpm/µg of protein on gamma counter system showed no MOI dependency with Ad-ΔE1A-TK infection until 24 hours and mild increases only at higher viral titers with longer incubation time. However, ΔE1B19/55-TK infection resulted in a linear fashion of MOI dependency of transgene expression from 24 to 72 hours postinfection. MTT assay as an indicator of cell viability showed that replicating viruses could have earlier and higher cytotoxicity than non-replicating viruses. Nevertheless, higher amounts of TK production were noted with replicating than non-replicating viruses at all time points.
The cells on plates were also imaged with a gamma camera used in routine clinical practice. The viruses were added for 48 hours to allow sufficient replication and produce enough TK without remarkable cell killing yet. On plate imaging, both Ad-ΔE1B19/55-TK and Ad-ΔE1A-TK infected cells showed increased I-131 FIAU uptake in a MOI dependent pattern. The differences in uptakes of I-131 FIAU between the 2 viral infections were larger with higher viral titers. The results on plate imaging for I-131 FIAU were in agreement with the results on gamma camera imaging. It seems clear that the expression of TK was qualitatively and quantitatively measured under various incubation conditions using a radiotracer based molecular imaging technique. Differing from cells without viruses and I-131 FIAU, cells treated only with I-131 FIAU showed mild uptake, likely due to the activity of endogenous TK-like enzymes.17 This information on gamma imaging would also be helpful in developing those prodrugs or radiotracers more specific to viral TK.
We performed MTT assay to measure cell viability after Ad-ΔE1B19/55-TK or Ad-ΔE1A-TK infection. Ad-ΔE1B19/55-TK led to more efficient cell killing than Ad-ΔE1A-TK after 48 and 72 hours of infection, and the amount of viruses needed was lower with Ad-ΔE1B19/55-TK for the same cytotoxicity. The results also confirmed the use of replicating viruses instead of non-replicating viruses for enhanced anticancer potency like others. However, the fraction of viable cells was still very high (around 50%) even with the highest viral titer of 40 MOIs of Ad-ΔE1B19/55-TK and longest incubation time of 72 hours. Despite the increased potency of cancer cell killing compared to non-replicating Ad-ΔE1A-TK, there is still need for further improvement of oncolytic profiles with ΔE1B19/55-TK. The deletion of E1B 55 kDa could have been attributable to the low oncolytic potency since it plays an important role in productive viral replication.7,14
While ΔE1B19/55-TK seems to have a low oncolytic potential, the high efficiency of TK expression in spite of cytotoxicity can be useful in combination treatment with GCV. In fact, Raki et al recently reported that GCV might not add antitumor activity in the context of highly potent oncolysis.7 We seeded cells on 24-well plates and infected with Ad-ΔE1B19/55-TK or Ad-ΔE1A-TK. Then, GCV was applied for 3 days at 48 hours postinfection. Without GCV treatment, the viral incubation time of 48 hours resulted in slightly reduced cell viability only with Ad-ΔE1B19/55-TK infection at higher MOIs. With GCV treatment, the reduction of cell viability was rather remarkable with ΔE1B19/55-TK infection than that with Ad-ΔE1A-TK infection. Therefore, in spite of the possibility of GCV treatment interfering with viral replication in vitro,20 anticancer efficacy of Ad-ΔE1B19/55-TK in combination with GCV in this study remains beneficial It is possible that the enhanced anticancer activity of the oncolytic adenovirus with HSVtk gene can include the bystander effects of the TK/GCV system.
In conclusion, the use of a replication-competent adenoviral variant of Ad-ΔE1B19/55-TK showed more efficient TK expression even in the presence of higher cancer cell killing effect than a replication-incompetent adenovirus of ΔE1A-TK. Although there have been contradictory results on the value of GCV in combination with replication-competent viruses, GCV treatment still possessed an additive role to the oncolytic effect of Ad-ΔE1B19/55-TK used in this study. Finally, we confirmed that the expression of transgene by oncolytic viruses could rapidly be screened qualitatively and quantitatively using a radiotracer-based counting and imaging technique which simulated the clinical setting of disease diagnosis and monitoring.
Figures and Tables
Fig. 1
Characterization of adenoviruses expressing HSVtk gene. (A) Specific primer sets corresponding to E1 and HSVtk gene are shown below the diagram of E1 and E3 region of adenovirus genome, and each expected size of PCR products is 479 bp and 700 bp, respectively. (B) PCR product analysis of Ad-ΔE1A, Ad-ΔE1A-TK, Ad-ΔE1B19/55, and Ad-ΔE1B19/55-TK. The presence of each PCR product verified the expression of the E1A gene and the insertion of HSVtk gene.
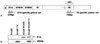
Fig. 2
Measurement of TK expression by I-131 FIAU and gamma counter. The YCC-2 cells were infected with a range of titers of Ad-ΔE1B19/55-TK (■) or Ad-ΔE1A-TK (▲) for 24 to 72 hours and were incubated with I-131 FIAU. On gamma counter analyses of lysed cells, cpm/µg of protein showed MOIs dependency with a linear increase from 1 to 40 MOI at all time points with ΔE1B19/55-TK infection. With Ad-ΔE1A-TK infection, cpm /µg of protein were unchanged over MOIs after 24 hours of infection and slightly increased after 48 and 72 hours of infection with 40 MOI. MOI, multiplicity of infection.
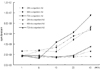
Fig. 3
MTT assay after Ad-ΔE1B19/55-TK (■) or Ad-ΔE1A-TK (▲) infection. The cytotoxicity was determined after infection with a range of titers of Ad-ΔE1B19/55-TK or Ad-ΔE1A-TK for 24 to 72 hours. Ad-ΔE1B19/55-TK led to more efficient cell killing than Ad-ΔE1A-TK after 48 and 72 hours of infection and the amount of viruses needed was lower with Ad-ΔE1B19/55-TK for the same cytotoxicity. MOI, multiplicity of infection.
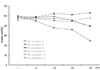
Fig. 4
(A) Gamma ray imaging using I-131 FIAU after Ad-ΔE1B19/55-TK or Ad-ΔE1A-TK infection. The cells were infected with Ad-ΔE1B19/55-TK or Ad-ΔE1A-TK at MOIs of 1, 10, and 40 for 48 hours. Both Ad-ΔE1B19/55-TK and Ad-ΔE1A-TK infected cells showed increased I-131 FIAU uptake in a MOI dependent pattern. (B) The differences in uptakes of I-131 FIAU between the two viral infections were larger with higher viral titers. MOI, multiplicity of infection.
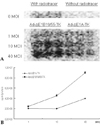
Fig. 5
Combination treatment with Ad-ΔE1B19/55-TK (■) or Ad-ΔE1A-TK (▲) and GCV. GCV was added at final concentration of 100 µg/mL at 48 hours postinfection. After additional 3 days of incubation, MTT assay was performed. Cell viability of ΔE1B19/55-TK infection was remarkably reduced compared to that of Ad-ΔE1A-TK infection. MOI, multiplicity of infection; GCV, ganciclovir.
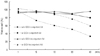
References
1. Glasgow JN, Bauerschmitz GJ, Curiel DT, Hemminki A. Transductional and transcriptional targeting of adenovirus for clinical applications. Curr Gene Ther. 2004. 4:1–14.


2. Barnett BG, Crews CJ, Douglas JT. Targeted adenoviral vectors. Biochim Biophys Acta. 2002. 1575:1–14.


3. Kay MA, Glorioso JC, Naldini L. Viral vectors for gene therapy: the art of turning infectious agents into vehicles of therapeutics. Nat Med. 2001. 7:33–40.


4. Hermiston T. Gene delivery from replication-selective viruses: arming guided missiles in the war against cancer. J Clin Invest. 2000. 105:1169–1172.


5. Kirn D. Clinical research results with dl1520 (Onyx-015), a replication-selective adenovirus for the treatment of cancer: what have we learned? Gene Ther. 2001. 8:89–98.


6. Wildner O, Morris JC, Vahanian NN, Ford H Jr, Ramsey WJ, Blaese RM. Adenoviral vectors capable of replication improve the efficacy of HSVtk/GCV suicide gene therapy of cancer. Gene Ther. 1999. 6:57–62.


7. Raki M, Hakkarainen T, Bauerschmitz GJ, Särkioja M, Desmond RA, Kanerva A, et al. Utility of TK/GCV in the context of highly effective oncolysis mediated by a serotype 3 receptor targeted oncolytic adenovirus. Gene Ther. 2007. 14:1380–1388.


8. Kim J, Cho JY, Kim JH, Jung KC, Yun CO. Evaluation of E1B gene-attenuated replicating adenoviruses for cancer gene therapy. Cancer Gene Ther. 2002. 9:725–736.


9. Bischoff JR, Kirn DH, Williams A, Heise C, Horn S, Muna M, et al. An adenovirus mutant that replicates selectively in p53-deficient human tumor cells. Science. 1996. 274:373–376.


10. Lee H, Kim J, Lee B, Chang JW, Ahn J, Park JO, et al. Oncolytic potential of E1B 55 kDa-deleted YKL-1 recombinant adenovirus: correlation with p53 functional status. Int J Cancer. 2000. 88:454–463.


11. Dix BR, O'Carroll SJ, Myers CJ, Edwards SJ, Braithwaite AW. Efficient induction of cell death by adenoviruses requires binding of E1B55k and p53. Cancer Res. 2000. 60:2666–2672.
12. Braithwaite AW, Russell IA. Induction of cell death by adenoviruses. Apoptosis. 2001. 6:359–370.
13. Nanda D, Vogels R, Havenga M, Avezaat CJ, Bout A, Smitt PS. Treatment of malignant gliomas with a replicating adenoviral vector expressing herpes simplex virus-thymidine kinase. Cancer Res. 2001. 61:8743–8750.
14. Haviv YS, Takayama K, Glasgow JN, Blackwell JL, Wang M, Lei X, et al. A model system for the design of armed replicating adenoviruses using p53 as a candidate transgene. Mol Cancer Ther. 2002. 1:321–328.
15. Edholm D, Molin M, Bajak E, Akusjärvi G. Adenovirus vector designed for expression of toxic proteins. J Virol. 2001. 75:9579–9584.


16. Lambright ES, Amin K, Wiewrodt R, Force SD, Lanuti M, Propert KJ, et al. Inclusion of the herpes simplex thymidine kinase gene in a replicating adenovirus does not augment antitumor efficacy. Gene Ther. 2001. 8:946–953.


17. Zinn KR, Chaudhuri TR, Buchsbaum DJ, Mountz JM, Rogers BE. Simultaneous evaluation of dual gene transfer to adherent cells by gamma-ray imaging. Nucl Med Biol. 2001. 28:135–144.


18. Johnson M, Sato M, Burton J, Gambhir SS, Carey M, Wu L. Micro-PET/CT monitoring of herpes thymidine kinase suicide gene therapy in a prostate cancer xenograft: the advantage of a cell-specific transcriptional targeting approach. Mol Imaging. 2005. 4:463–472.
19. Jacobs A, Voges J, Reszka R, Lercher M, Gossmann A, Kracht L, et al. Positron-emission tomography of vector-mediated gene expression in gene therapy for gliomas. Lancet. 2001. 358:727–729.