Abstract
Purpose
For patients with acute respiratory failure due to lung edema or atelectasis, Surplus lung water that is not removed during an initial stay in the Intensive Care Unit (ICU) may be related to early ICU readmission. Therefore, we performed a retrospective study of patient management during the first ICU stay for such patients.
Materials and Methods
Of 1,835 patients who were admitted to the ICU in the 36 months from January, 2003 to December, 2005, 141 were patients readmitted, and the reason for readmission was lung edema or atelectasis in 21 patients. For these 21 patients, correlations were investigated between body weight gain at the time of initial ICU discharge (weight upon discharge from the ICU ÷ weight when entering the ICU) and the time to ICU readmission, between body weight gain and the P/F ratio at ICU readmission, between the R/E ratio (the period using a respirator (R) ÷ the length of the ICU stay after extubation (E)) and the time to ICU readmission, between the R/E ratio and body weight gain, and between body weight gain until extubation and the time to extubation.
Results
A negative linear relationship was found between body weight gain at the time of initial ICU discharge and the time to ICU readmission, and between body weight gain at the time of ICU discharge and the P/F ratio at ICU readmission. If body weight had increased by more than 10% at ICU discharge or the P/F ratio was below 150, readmission to the ICU within three days was likely. Patients with a large R/E ratio, a large body weight gain, and a worsening P/F ratio immediately after ICU discharge were likely to be readmitted soon to the ICU. Loss of body weight during the period of respirator support led to early extubation, since a positive correlation was found between the time to extubation and body weight gain.
Conclusion
Fluid management failure during the first ICU stay might cause ICU readmission for patients who had lung edema or atelectasis. Therefore, a key to the prevention of ICU readmission is to ensure complete recovery from lung failure before the initial ICU discharge. Strict water management is crucial based on body weight measurement and removal of excess lung water is essential. In addition, an apparent improvement in respiratory state may be due to respiratory support, and such an improvement should be viewed cautiously. Loss of weight at the refilling stage of transfusion prevents ICU readmission and may decrease the length of the ICU stay.
Of 1,835 patients who entered the ICU during the 36 months from January, 2003 to December, 2005, 141 were readmitted within one month of their initial discharge. Acute respiratory failure by lung edema or atelectasis was present in 21 of these patients (14.9% of the readmitted patients, and 1.1% of all ICU patients). For these 21 patients, the body weight on the last day of the first ICU stay was markedly higher than that on the first day. In addition, most of the patients had normalized respiratory states at the time of initial ICU discharge, but aggravation of the respiratory state occurred immediately after discharge, with lung consolidation. Patients requiring a longer period of artificial respiration and those with a shorter ICU stay after extubation had a shorter time to ICU readmission. After readmission, their respiratory state was improved by decreasing the body weight by strict water management.
It is generally thought that weight reduction by muscle contraction of 0 - 0.5 kg/day occurs during bed rest,1-3 and that body weight gain due to improved nourishment occurs about four weeks later,4-6 therefore, it is highly likely that the body weight gain may occur become of internal surplus water. In turn, this might cause lung edema or atelectasis and worsening of the respiratory state, suggesting that excess lung water that was not removed during the first ICU stay may be associated with subsequent development of lung edema or atelectasis and early readmission to the ICU. Therefore, we performed a retrospective study of patient management during the first ICU stay for patients who were readmitted to the ICU with lung edema or atelectasis.
During the 36-month period from January 1, 2003 to December 31, 2005, 1,835 patients were admitted to our ICU. Of these patients, 141 (7.6% of the total number of admissions) were readmission cases, and 21 of the 141 patients were suffering from acute respiratory failure due to lung edema or atelectasis. Thus, patients with lung edema or atelectasis comprised 14.9% of readmitted patients and 1.1% of the total number of ICU patients. We investigated the patients who had lung consolidation and were diagnosed with acute respiratory failure due to lung edema or atelectasis. Patients with a diagnosis of a disease due to a specific bacterial cause, such as pneumonia, were excluded from the study. Since almost all patients readmitted to the ICU more than one month after their initial ICU stay did not have a condition that was connected directly with the disease that caused the first ICU admission, we investigated only patients who were readmitted to the ICU within one month of their initial ICU stay.
Acute respiratory failure was defined as PaO2 < 60 Tor for indoor inspiration or a respiratory disorder equivalent to PaO2 < 60 Tor.7 This threshold is the definition of respiratory failure in Japan. Respiratory function was evaluated using the P/F ratio.8 The initial and final body weights during the first ICU period, body weight gain at the time of ICU discharge (weight upon discharge from the ICU ÷ weight when entering the ICU), the time to ICU readmission, the P/F ratio on the last day of the first ICU stay and on the first day of the second admission, the period using the respirator, the length of the initial ICU stay after extubation, and the R/E ratio (the period using a respirator (R) ÷ the length of the ICU stay after extubation (E)) were measured retrospectively. Correlations were investigated between body weight gain at the time of ICU discharge and the time to ICU readmission, between body weight gain and the P/F ratio at ICU readmission, between the R/E ratio and the time to ICU readmission, between the R/E ratio and body weight gain, and between body weight gain until extubation and the time to extubation.
Clinical data during the first ICU admission period for the 21 readmitted acute respiratory failure patients are shown in Tables 1 and 2. A negative linear relationship was found between body weight gain at the time of initial ICU discharge and the time to ICU readmission (Fig. 1). A weight increase of more than 10% at the time of ICU discharge was likely to cause readmission to the ICU within three days. A negative linear relationship was also found between body weight gain at the time of ICU discharge and the P/F ratio at ICU readmission (Fig. 2). A weight increase of more than 10% at ICU discharge and a P/F ratio below 150 were likely to result in readmission to the ICU due to severe respiratory failure.
An inverse relationship was found between the R/E ratio and the time to ICU readmission (Fig. 3). A large R/E ratio indicated that patients left the ICU soon after long-term respirator management, and this was associated with a short period before ICU readmission. An R/E ratio of over 5 indicated that patients were likely to return to the ICU within 5 days. A direct relationship was found between the R/E ratio and body weight gain (Fig. 4); hence, a large R/E ratio correlated with high body weight gain at the time of ICU discharge. A positive linear relationship was found between body weight gain until extubation and the time to extubation (Fig. 5); as weight increased during the period of respiratory support, the time to extubation lengthened.
Cytokine release is stimulated by inflammation, and these cytokines stimulate adherence of leukocytes and monocytes to endothelial cells of blood vessels. Elastase and oxygen radicals released by leukocytes may then cause damage to the endothelial cells,9-11 causing circulation failure and increased blood-vessel permeability.12-15 Transfusion can be performed to compensate for the insufficient amount of plasma due to increased blood-vessel permeability and this may stabilize the circulation dynamics.
The lungs are especially prone to leukocyte-induced damage. Once the blood-vessel endothelial cells are damaged by activated leukocytes in the acute phase, increased blood-vessel permeability in the lungs allows plasma to move into the alveolar space from outside the lung blood vessels.16-18 Transfusion can be performed to stabilize the circulation, nevertheless plasma may leak outside of the lung blood vessels due to increased permeability.19-21 The relative increase in the amount of fluid outside the lung blood vessels causes alveolar collapse, a fall in lung compliance, lung edema, and atelectasis, thus leading to pre-lung failure. This kind of lung failure reflects a state in which alveolar regions with poor ventilation are formed locally. Even if breathing movement is satisfactory and alveolar ventilation is maintained, full inspiration of oxygen cannot occur because a blood-flow shunt has arisen in the lungs.22-25
Once the lung blood-vessel endothelium is restored, the permeability is reduced and the circulation is stabilized, the transfusion procedure should enter the refilling stage.26 At this stage, diuresis is used to normalize the amount of fluid outside the blood vessels since extracellular fluid has returned to the lung blood vessels. This requires immediate reduction of transfusion, performance of diuresis and prompt discharge of the extracellular fluid from the body. Improvement of oxygenation is also important for recovery from pre-lung failure. However, judgment of the timing of this procedure is not easy in practice, and there is often a failure to normalize the extracellular fluid due to continuation of superfluous transfusion and inadequate diuresis. Consolidation of the lungs may therefore arise, and this kind of lung failure might be viewed as iatrogenic respiratory failure. Many cases of lung edema in the ICU may not also be due to bacteria.27,28
The development of respirators has allowed maintenance of oxygenation, even in patients with severe lung failure, by providing the correct amount of ventilation. PEEP is particularly effective for atelectasis caused by lung edema, because it increases the lung expiration capacity, widens the peripheral respiratory tract, and re-expands the collapsed alveolar space. As a result, oxygenation appears to improve, however, when PEEP is stopped the respiratory state worsens; hence, this procedure does not address the fundamental causes of lung failure. The basic treatment for lung failure is simply to normalize surplus extracellular fluid outside the lung blood vessels. Extubation by PEEP before the extracellular fluid is normalized does allow oxygenation to be maintained at a high concentration of oxygen, but the alveolar space opened by PEEP collapses in a short time and this may cause lung consolidation in this region.
In the current work, a negative linear relationship was found between body weight gain at the time of ICU discharge and the time to ICU readmission (Fig. 1) and between body weight gain at the time of ICU discharge and the P/F ratio at the time of ICU readmission (Fig. 2). A weight increase of more than 10% at ICU discharge or a P/F ratio of below 150 was associated with readmission to the ICU within three days. Body weight should decrease steadily from entering the ICU by about 0.5 kg/day due to muscle wasting by bed rest, as mentioned above; therefore, the actual body weight gain at ICU discharge is larger than the measured value, since some weight loss should have occurred in the ICU.
A large R/E ratio, which indicates that a patient left the ICU soon after long-term respirator management, was associated with a large body weight gain at the time of ICU discharge (Fig. 4). Since a large body weight gain tends to lead to ICU readmission (Figs. 1 or 2), it is desirable to have a small R/E ratio. A causative link cannot be proved from this study, however the data in Fig. 3 suggest that readmission to the ICU is unlikely when the R/E ratio is around 1, suggesting that it may be preferable to keep patients in the ICU after extubation for the same period for which a respirator was used. However, this is difficult in practice due to medical costs and desire to limit the number of hospitalization days. Reduction of the R value (the period of respiratory support) would also have a favorable effect on the R/E ratio.
From Fig. 5, losing weight during the period of respiratory support is likely to lead to early extubation, since the time to extubation was related to body weight gain. In other words, losing body weight at the refilling stage prevents ICU readmission and may decrease the length of the ICU stay. Fluid management failure during the first ICU stay may result in ICU readmission due to lung edema or atelectasis. A key for prevention of ICU readmission is to obtain complete reversal of lung failure before ICU discharge, using strict water management based on weight measurements and with care not to assume that an apparent improvement in the respiratory state will be sustained, since this improvement may be due to respiratory support.
Among patients readmitted to the ICU with respiratory failure due to lung edema or atelectasis, there was an association between fluid overload at discharge and respiratory status, as well as a negative correlation between fluid overload at the time of initial discharge from the ICU and the time to readmission. A decrease in body weight before initial ICU discharge may be crucial for prevention of ICU readmission.
Figures and Tables
Fig. 1
A negative linear relationship (R = - 0.98) was found between body weight gain at the initial ICU discharge and the time to ICU readmission. A weight increase of more than 10% at the time of ICU discharge suggested readmission to the ICU within three days was likely.
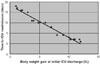
Fig. 2
A negative linear relationship (R = - 0.96) was found between body weight gain at the initial ICU discharge and the P/F ratio at ICU readmission. A weight increase of more than 10% at ICU discharge or a P/F ratio below 150 indicated probable readmission to the ICU with severe respiratory failure.
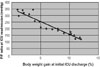
Fig. 3
An inverse relationship (R = - 0.74) was found between the R/E ratio and the time to ICU readmission. A large R / E ratio was correlated with a short time to ICU readmission.
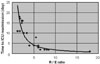
Fig. 4
A direct relationship (R = 0.81) was found between the R/E ratio and body weight gain. A large R/E ratio was correlated with a large body weight gain at the time of initial ICU discharge.
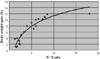
Fig. 5
A positive linear relationship (R = 0.75) was found between body weight gain until extubation and the time to extubation. As the weight increased during respirator use, the time to extubation was prolonged.
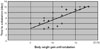
Table 1
Clinical Data During the First ICU Admission Period for the 21 Readmitted Acute Respiratory Failure Patients
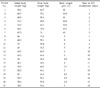
References
1. Forbes GB. Weight loss during fasting: implications for the obese. Am J Clin Nutr. 1970. 23:1212–1219.


2. Benedict FG. A study of Prolonged Fasting. 1915. Washington, DC: Carnegie Institution of Washington;69–87.
3. Drenick EJ, Swendseid ME, Blahd WH, Tuttle SG. Prolonged starvation as treatment for severe obesity. JAMA. 1964. 187:100–105.


4. Spady DW, Payne PR, Picou D, Waterlow JC. Energy balance during recovery from malnutrition. Am J Clin Nutr. 1976. 29:1073–1088.


5. Long CL. Energy balance and carbohydrate metabolism in infection and sepsis. Am J Clin Nutr. 1977. 30:1301–1310.
6. Long CL, Kinney JM, Geiger JW. Nonsuppressability of gluconeogenesis by glucose in septic patients. Metabolism. 1976. 25:193–201.


7. Zaitsu A. The latest trends in the treatment of acute respiratory failure. Fukuoka Igaku Zasshi. 1990. 81:391–395.
8. Covelli HD, Nessan VJ, Tuttle WK 3rd. Oxygen derived variables in acute respiratory failure. Crit Care Med. 1983. 11:646–649.


9. Nakae H, Endo S, Inada K, Kasai T, Yoshida M. Significance of alpha-tocopherol and interleukin 8 in septic adult respiratory distress syndrome. Res Commun Chem Pathol Pharmacol. 1994. 84:197–202.
10. Endo S, Inada K, Inoue Y, Kuwata Y, Suzuki M, Yamashita H, et al. Two types of septic shock classified by the plasma levels of cytokines and endotoxin. Circ Shock. 1992. 38:264–274.
11. Endo S, Inada K, Yamada Y, Kasai T, Takakuwa T, Nakae H, et al. Plasma tumour necrosis factor-alpha (TNF-alpha) levels in patients with burns. Burns. 1993. 19:124–127.


12. Endo S, Inada K, Yamashita H, Takakuwa T, Nakae H, Kasai T, et al. Platelet-activating factor (PAF) acetylhydrolase activity, type II phospholipase A2, and cytokine levels in patients with sepsis. Res Commun Chem Pathol Pharmacol. 1994. 83:289–295.
13. Endo S, Inada K, Ceska M, Takakuwa T, Yamada Y, Nakae H, et al. Plasma interleukin 8 and polymorphonuclear leukocyte elastase concentrations in patients with septic shock. J Inflamm. 1995. 45:136–142.
14. Petty TL. Protease mechanisms in the pathogenesis of acute lung injury. Ann N Y Acad Sci. 1991. 624:267–277.


15. Edens HA, Parkos CA. Neutrophil transendothelial migration and alteration in vascular permeability: focus on neutrophil-derived azurocidin. Curr Opin Hematol. 2003. 10:25–30.


16. Su X, Camerer E, Hamilton JR, Coughlin SR, Matthay MA. Protease-activated receptor-2 activation induces acute lung inflammation by neuropeptide-dependent mechanisms. J Immunol. 2005. 175:2598–2605.


17. Guo M, Wu MH, Granger HJ, Yuan SY. Focal adhesion kinase in neutrophil-induced microvascular hyperpermeability. Microcirculation. 2005. 12:223–232.


18. Brigham KL, Meyrick B. Endotoxin and lung injury. Am Rev Respir Dis. 1986. 133:913–927.
20. Falk JL, O'Brien JF, Kerr R. Fluid resuscitation in traumatic hemorrhagic shock. Crit Care Clin. 1992. 8:323–340.


22. Dantzker DR. Dantzker DR, editor. Pulmonary gas exchange. Cardiopulmonary critical care. 1991. 2nd ed. Philadelphia: W.B. Saunders;25–43.
23. Lanken PN. Grippi MA, editor. Ventilation-perfusion relationships. Pulmonary pathophysiology. 1995. Philadelphia: JB Lippincott;195–210.
24. Buohuys A. Fenn WO, Rahn H, editors. Respiratory dead space. Handbook of physiology: respiration. 1964. Bethesda, MD: American Physiological Society;699–714.
25. D'Alonzo GE, Dantzker DR. Respiratory failure, mechanisms of abnormal gas exchange, and oxygen delivery. Med Clin North Am. 1983. 67:557–571.
26. Matsui M, Kudo T, Kudo M, Ishihara H, Matsuki A. The endocrine response after burns. Agressologie. 1991. 32:233–235.