Abstract
Purpose
The sarco/endoplasmic reticulum Ca2+-ATPase (SERCA), encoded by ATP2A2, is an essential component for G-protein coupled receptor (GPCR)-dependent Ca2+ signaling. However, whether the changes in Ca2+ signaling and Ca2+ signaling proteins in parotid acinar cells are affected by a partial loss of SERCA2 are not known.
Materials and Methods
In SERCA2+/- mouse parotid gland acinar cells, Ca2+ signaling, expression levels of Ca2+ signaling proteins, and amylase secretion were investigated.
Results
SERCA2+/- mice showed decreased SERCA2 expression and an upregulation of the plasma membrane Ca2+ ATPase. A partial loss of SERCA2 changed the expression level of 1, 4, 5-tris-inositolphosphate receptors (IP3Rs), but the localization and activities of IP3Rs were not altered. In SERCA2+/- mice, muscarinic stimulation resulted in greater amylase release, and the expression of synaptotagmin was increased compared to wild type mice.
The parotid gland is a well-known model for Ca2+ signaling because salivary fluid secretion is evoked by increases of intracellular calcium concentration ([Ca2+]i) following the activation of G protein coupled receptors (GPCRs). Increases of [Ca2+]i accelerate fluid secretion by adjusting the opening of Ca2+-dependent Cl- channels.1 Together with fluid secretion, protein secretion in parotid gland acinar is usually caused by an increase in intracellular cAMP concentration with stimulation of β-adrenergic receptor and activation of cAMP-dependent protein kinase A (PKA), which induces cell membrane fusion of secretory vesicles.1 However, the exact mechanism for exocytosis of secretory vesicles is still not known, specifically which protein is phosphorylated by PKA.2 In addition to β-adrenergic stimulation, stimulation of the muscarinic, substance P, or β-adrenergic receptors also elicits significant amylase release from the parotid although the levels of amylase are significantly lower than those observed from a β-adrenergic receptor-mediated response. The stimulation of these receptors activates phosphatidyl-inositide metabolism and induces an increase in [Ca2+]i without affecting intracellular cAMP levels.3
Ca2+ acts as an intracellular messenger, relaying information within cells to regulate their activity. The intracelluar free calcium ion, Ca2+, is a common second messenger, and has roles in fertillization, muscle contraction, neurotransmitter release, fluid secretion, exocytosis, memorization, and learning.4 There is a close connection between GPCRs and Ca2+ signaling in parotid gland acinar cell. GPCRs activate Gq to release Gαq and Gβγ. Gαq activates phospholipase Cβ to generate 1, 4, 5-trisinositolphosphate (IP3) in the cytosol to release Ca2+ from the endoplasmic reticulum (ER). Ca2+ release from the ER leads to activation of store-operated Ca2+ channels in the plasma membrane, and the Ca2+ release and influx increase the [Ca2+]i. Subsequently, the plasma membrane Ca2+ pump (PMCA) and SERCA remove Ca2+ from the cytosol to reduce [Ca2+]i toward resting levels until the [Ca2+]i stabilizes at a plateau.5
It is known that SERCA carries out several different functions in cells. First, it acts as a buffer of [Ca2+]i in resting cells6 and controls store operated Ca2+ channel activity when cells are stimulated.7 Second, it reloads Ca2+ stores at the end of cell stimulation. Moreover, it is reported that SERCA is involved in [Ca2+]i oscillations and Ca2+ waves that are typical physiological Ca2+ responses for the transmission of signals when cells are stimulated by agonists.8 ATP2A2 encodes 2 types of Ca2+-transporting ATPases, SERCA2a and SERCA2b, which differ in their C-terminal sequences as a result of alternative splicing.6 SERCA2a is expressed at the highest levels in the heart, where it plays a central role in Ca2+ handling required for excitation/contraction coupling in cardiomyocytes.9 In contrast to the limited tissue distribution and organ-specific function of SERCA2a, SERCA2b is expressed in all tissues, suggesting that it plays an essential housekeeping role, although it may serve some organ-specific functions as well.10 Therefore, in SERCA2 homozygote mice, lack of functional ATP2A2 gene leads to embryonic lethality.11 However, SERCA2 heterozygote mice (SERCA2+/-) have a normal appearance and do not seem to have any abnormal conditions.12 Recently a partial loss of SERCA2 in SERCA2+/- mouse pancreatic acinar cells has been known to cause increased PMCA expression and activation, resulting in a change of [Ca2+]i oscillation frequency, upregulation of synaptotagmin, and an overall adaptation phenomenon.13 This adaptation partially explains why patients with Dariers disease (DD), which is due to mutations in the ATP2A2 gene, do not present any extremely serious conditions. Although several of the mutations in ATP2A2 that cause DD were associated with a neuropsychiatric phenotype, all other physiological functions, including function of the cardiovascular system, appear normal in DD patients.14
In parotid acinar cells, an increase of [Ca2+]i induces fluid secretion and protein exocytosis including amylase. However, whether the changes in Ca2+ signaling and Ca2+ signaling proteins in parotid acinar cells are affected by a partial loss of SERCA2 are not known. In the present study, the effects of SERCA2 deficiency on Ca2+ signaling, expression levels of Ca2+ signaling proteins, and amylase secretion were investigated in SERCA2+/- mouse parotid gland acinar cells.
Fura-2-acetoxymethyl ester (Fura-2/AM), Fluo-3 K+ salt, and Fura-2 Na+/K+ (free acid form of fura-2) were purchased from Teflabs (Austin, TX, USA). Collagenase type IV, carbamyl choline chloride (carbachol), pilocarpine HCl, soybean trypsin inhibitor, N-[2-hydroxyethyl] piperazine-N'-[2-ethanesulfonic acid] (HEPES), and D-glucose were purchased from Sigma (St. Louis, MO, USA). Bovine serum albumin (BSA) and pyruvic acid were from Amnesco (Solon, OH, USA). All IP3Rs pAbs were a generous gift from Dr. Akihiko Tanimura (University of Hokkaido, Ishikari-Tobetsu, Japan). Synaptotagmin mAbs and syntaxin pAbs were generous gifts from Dr. Shmuel Muallem (University of Texas Southwestern Medical Center, Dallas, TX, USA). The PMCA mAb was purchased from Transduction Laboratory (San Jose, CA, USA).
Wild-type (WT, 25 - 28 g) and SERCA2+/- (25 - 28g) mice11 were sacrificed by cervical dislocation. The cells were prepared from the parotid gland by limited collagenase digestion as previously described.8 In order to achieve the pure isolation of acinar cells, density gradient centrifugation was performed with Accudenz and the purity of the acinar cells were confirmed via light microscopy. After isolation, the acinar cells were resuspended in an extracellular physiologic salt solution (PSS): 140 mM NaCl; 5 mM KCl; 1 mM MgCl2; 1 mM CaCl2; 10 mM HEPES; and 10 mM glucose titrated to pH 7.4. The osmolality of the extracellular solution (measured with a FISKE 110 osmometer) was 310 mOsm.
Cells were incubated for 40 min in PSS containing 5 µM fura 2-AM with pluronic F-127 to enhance dye loading. Changes in [Ca2+]i were measured by means of fura 2 fluorescence, with excitation wavelengths of 340 nm and 380 nm, and an emission wavelength of 510 nm at room temperature. Background fluorescence was subtracted from the raw signals at each excitation wavelength before calculating the fluorescence ratio as: ratio = F340/F380. The emitted fluorescence was monitored with a CCD camera (Photon Technology International Inc., Lawrenceville, NJ, USA) attached to an inverted microscope. Fluorescence images were obtained at 2-s intervals. Each cell was then stimulated by carbachol in a dose-dependent manner.
Animals were allowed water but starved for 24h prior to the experiment. Each acinar cell was stimulated with equal concentrations of the carbachol used in the [Ca2+]i measurement study. Acinar cells were incubated with carbachol for 20 min in a shaking incubator at 37℃ and 60 rpm. Acinar cells were lysed by sonication. The lysates were clarified by centrifugation at 13,000 rpm for 10 min. The total amylase content or content of amylase released into the medium was determined by the method described by Bernfeld et al.15 Aliquots of the incubation medium and the supernatants of the homogenized glands were incubated with a 0.5% starch suspension for 10 min at 37℃. Absorbance was measured at 540 nm. Amylase activity in the medium was expressed as a percentage of the total activity.
Protein extracts were prepared from parotid acinar cells as follows. Pure acinar cells were washed with ice-cold PBS and then lysed by the addition of Tris-Hcl, NaCl, and EDTA buffer [1% NP-40, 10 mM of Tris HCl (pH 7.8), 150 mM NaCl, 1 mM EDTA, 2 mM Na3VO4, 10 mM NaF, 10 µg/mL aprotinin, 10 µg/mL leupeptine, 10 µg/mL PMSF]. The lysates were clarified by centrifugation at 13,000 rpm for 10 min. Samples were separated by 12% SDS-PAGE. Proteins in the gel were transferred onto nitrocellulose membrane (Schleicher and Schuell Bioscience, Dassel, Germany) for 1 h at 200 mA. The nitrocellulose membrane was blocked by incubation in 6% skim milk in TTBS buffer (1X TBE solution + 0.1% Tween 20). The membrane was then probed overnight at 4℃ with primary Abs. After the membrane was washed 3 times with TTBS buffer, it was incubated with horseradish peroxidase conjugated secondary antibody (Santa Cruz Biotechnology, Santa Cruz, CA, USA) in TTBS buffer containing 3% skim milk for 1 h at room temperature, and washed again with TTBS buffer. Detection was performed using an ECL detection system (Amersham Biosciences, Uppsala, Sweden), and immunoreactive bands were visualized using Medical X-Ray film (AGFA).
Cells were plated on a glass coverslip for 10 min at room temperature prior to fixation with methanol. After fixation, the immunostaining was performed as previously described.16 All of the antibodies were used at a dilution of 1 : 200 for immunolocalization of IP3Rs. Images were collected with a confocal microscope (Carl Zeiss, Germany).
To directly measure the rate of Ca2+ efflux by PMCA, the appearance of Ca2+ in the external medium was measured as previously described17 with minor modifications. Intact acini were washed once and suspended in medium containing 120 mM KCl, 20 mM NaCl, 10 mM HEPES pH7.4 with KOH, 10 mM glucose, and 2 µM of the free acid fura-2. After initiation of fluorescence recording, 7.5 mM EGTA was added to reduce the extra cellular Ca2+ concentration to ~100 nM. After establishing a baseline leak for ~1 min, the cells were stimulated with 1 mM carbachol. At the end of experiment, the signals were calibrated by adding 1 mM CaCl2 and 1 mM MnCl2 to the medium.
This was achieved using the previously described SLO permeabilization systems.13 The cells were washed with a high K+, chelax-treated medium and added to the same medium containing an ATP regeneration system (composed of 3 mM ATP, 5 mM MgCl2, 10 mM creatine phosphate and 5 U/mL creatine kinase), a cocktail of mitochondria inhibitors, 2 µM Fluo3 and 3 mg/mL SLO (Difco, Detroit, MI, USA). After addition, the cells were instantaneously permeabilized to molecules up to 20 kDa so that Ca2+ uptake into the ER could be measured immediately. Uptake of Ca2+ into the ER was allowed to continue until medium [Ca2+] was stabilized. Increasing concentrations of nonmetabolized IP3 were added to measure the extent of Ca2+ release and the potency of IP3 in mobilizing Ca2+ from the ER.
WT and SERCA2+/- mice were anesthetized with chloral hydrate (500 mg/kg body weight, intraperitoneal), and the main secretory ducts of the parotid glands were isolated using a dissecting microscope. The parotid glands were stimulated with Pilocarpine HCl (mg/kg, intraperitoneal), and parotid saliva was collected directly from the isolated parotid gland ducts to avoid contamination of saliva from other areas (e.g. tracheal and nasal secretions).
We hypothesized that the Ca2+ signaling-related protein expression levels or activities in SERCA2+/- mouse parotid gland acinar cells might be changed due to the reduced gene dosage and decreased expression of SERCA2. Thus, protein expression levels of 3 types of IP3Rs, PMCA, and SERCA2b were investigated in WT and SERCA2+/- mice. SERCA2b expression was decreased to 59 ± 3.52%, PMCA increased to 74 ± 10.68%, IP3R1 increased to 48 ± 4.21%, IP3R2 decreased to 67 ± 5.04%, and IP3R3 increased to 38 ± 8.94% in SERCA2+/- mice compared to WT (n = 4) (Figs. 1A and B). In addition, the localization of IP3Rs was not affected by a partial loss of SERCA2 (n = 4), as they were present on the apical side as reported in a previous study.8
In order to measure the activity of the IP3Rs, the permeability of plasma membrane was increased using streptolysin O (SLO) and Fluo-3 was loaded into the cells. The cells were sequentially stimulated with non-metabolized 2, 4, 5-IP3 and the change of Ca2+ concentration changes were measured as the activity of the IP3Rs. The rate of Ca2+ reduction was measured as SERCA activity as described in the Methods section. As shown in Fig. 2A, SERCA activity in SERCA2+/- parotid acinar cells was decreased by ~2-folds. However, the [Ca2+] after maximum Ca2+ removal was similar in both cell types with the WT at 17.75 ± 4.99 nM and SERCA2+/- at 17.25 ± 3.09 nM (n = 4). When 1 µM IP3 was added, the Ca2+ concentration was increased to 30.75 ± 4.78 nM in WT and 35 ± 3.55 nM in SERCA2+/-. In the second trial of 1 µM IP3, WT had a Ca2+ concentration of 53.75 ± 5.79 nM and SERCA2+/- was 55.00 ± 6.68 nM. In the third trial, the Ca2+ concentration in WT was 90.00 ± 9.83 nM, while Ca2+ concentration was 106.75 ± 16.81 nM (n= 5) in SERCA2+/-. Lastly, when 1 mM carbachol was added, the Ca2+ concentration in WT was 155.25 ± 16.21 nM and 177.5 ± 18.62 nM in SERCA2+/- (n = 5) (Figs. 2A and B). These results demonstrated that there was no significant difference in the reactivity of their IP3Rs between the two types of mice.
To measure PMCA activity, intact acinar cells were used, and Ca2+ concentration outside the cells increased to saturation level approximately 5 - 6 min after agonist stimulation (Fig. 2C). The Ca2+ concentration in WT at this point was 12.74 ± 1.49 nM and 20.98 ± 2.71 nM in SERCA2+/-; thus, PMCA activity was increased approximately 2-fold in SERCA2+/- cells (p = 0.0147, n = 4) (Figs. 2C and D).
Previous studies showed that Ca2+ signaling could be changed by decreased expression of SERCA2 gene in SERCA2+/- mice parotid gland acinar cells.18,13 In this study, therefore, [Ca2+]i was measured as fura2 ratio intensity (340/380): the increasing rate of [Ca2+]i was compared with the Ca2+ entry from outside of cells (Figs. 3A and B) and amounts of Ca2+ in ER (Ca2+ER, Fig. 3C) between WT and SERCA2+/- mouse parotid gland acinar cells when evoked by agonist stimulation. Carbachol, a muscarinic agonist, was used to stimulate both types of cells to increase [Ca2+]i. The first stimulation with 1 mM carbachol caused rapid [Ca2+]i increase to 0.907 ± 0.031 in WT and to 0.910 ± 0.053 in SERCA2+/- (Fig. 3A). Cells were subsequently washed with nominally Ca2+-free media and perfused with PSS containing 1 mM Ca2+. The [Ca2+]i increased to 1.085 ± 0.107 in WT and to 1.167 ± 0.139 in SERCA2+/- mice. Cells were washed with Ca2+-free media containing 1 µM thapsigargin, an inhibitor of SERCA, and perfused with PSS containing 1 mM Ca2+. The Ca2+ entry from the outside was measured and found to be 0.652 ± 0.062 in WT and 0.684 ± 0.056 in SERCA2+/- (n = 5). After Ca2+ER was depleted by thapsigargin with carbachol in Ca2+-free media, the Ca2+ entry was 0.464 ± 0.058 in WT and 0.448 ± 0.063 in SERCA2+/- mice (n = 6, Fig. 3B). The Ca2+ER and frequency of [Ca2+]i oscillations were not different between both cells (n = 5, n = 4, respectively) (Figs. 3C and D). These results indicate that there are no significant differences in Ca2+ signaling between WT and SERCA2+/- cells.
The effects of partial loss of SERCA2 in parotid acinar cells on salivary fluid secretion and amylase secretion were investigated. Each mouse was anesthetized and injected with pilocarpine HCl (1 mg/kg), a muscarinic agonist, to induce saliva secretion. Approximately 10 min after injection of the agonist, maximal secretion was observed in the WT and SERCA2+/- mice. In the WT, 4.45 ± 0.44 mg of saliva was secreted, while 4.46 ± 0.39 mg was secreted (n = 4) in the SERCA2+/- (Fig. 4A). Thus, no significant difference in secretion rate was noted. When the parotid acinar cells of the WT and SERCA2+/- were stimulated with 10-7, 3 × 10-7, 10-6, 3 × 10-6, and 10-4 M carbachol, amylase secretion was dependent on the agonist concentrations in both types of mice (Fig. 4B). In the WT, amylase releases in response to the stimulation of carbachol were as follows: at 10-7 M, 1.7 ± 0.21%; at 3 × 10-7 M, 5.3 ± 0.59%; at 10-6 M, 9.5 ± 0.61%; at 3 × 10-6 M, 11.1 ± 1.0%; and at 10-4 M, 8.9 ± 1.0%. In the SERCA2+/-, amylase release rates in response to the stimulation of carbachol were as follows: at 10-7 M, 2.9 ± 0.39%; at 3 × 10-7 M, 7.0 ± 1.25%; at 10-6 M, 11.7 ± 0.78%; at 3 × 10-6 M, 13.6 ± 0.77%; and at 10-4 M, 10.6 ± 0.75%. These results demonstrate a significant difference in amylase release rates between WT and SERCA2+/- mice parotid acinar cells (p < 0.05, n = 6) (Fig. 4B), indicating that Ca2+-dependent amylase secretion in SERCA2+/- mice was more sensitive to the agonist, although Ca2+-dependent salivary secretion was similar in both types.
To examine Ca2+ sustaining rates in both types of mice, [Ca2+]i in response to a agonist exposure was measured for 20 min after stimulation with 1, 3, and 100 µM carbachol. Each concentration resulted in rapid [Ca2+]i increases and sustained plateau for 20 min. At 20 min after stimulation with 1 µM carbachol, the WT had a sustained plateau at 0.1384 ± 0.0184 and the SERCA2+/- at 0.1678 ± 0.0206. At 3 µM stimulation, the WT was 0.1354 ± 0.0104 while the SERCA2+/- was 0.1809 ± 0.0176. At 100 µM stimulation, the WT was 0.3184 ± 0.0664 while the SERCA2+/- was 0.3878 ± 0.0543 (n = 4) (Fig. 4C). Next, the total amount of amylase and syntaxin-2, potentially related to amylase secretion,19 were measured. No significant changes in the expression levels of syntaxin-2 and β-amylase were found (Fig. 4D), however synaptotagmin expression was increased by 88 ± 4.54% in SERCA2+/- cells (p = 0.00148, n = 4, Figs. 4D and E).
In the present work, we studied Ca2+ signaling and related function in parotid gland acinar cells from SERCA2+/- mice in which other tissues, such as cardiac muscle cells and pancreas, have earlier been examined.18,13 We found that the expression level of SERCA2b was ~40% lower in parotid gland acinar cells from SERCA2+/- mice than that of WT mice, and the rate of Ca2+ uptake into SLO-permeabilized parotid acinar cells was ~50% slower than into cells from WT mice, indicating that a function of SERCA in SERCA2+/- mice was significantly decreased (Figs. 1 and 2). The expression level and activity of PMCA were increased ~2-fold, suggesting that a partial loss of SERCA2 is compensated by upregulation of PMCA in these cells. Upregulation of PMCA has also been seen in SERCA2+/- mice pancreatic acinar cells13: In pancreatic acinar cells from SERCA2+/- mice, [Ca2+]i transient by agonist stimulation was shorter and there is ~50% reduction in [Ca2+]i oscillation frequency.13 However, in the present study, there was no difference in Ca2+ signaling, including the increase of [Ca2+]i rate, Ca2+ entry, and the amount of Ca2+ in ER between WT and SERCA2+/- mouse parotid gland acinar cells, when stimulated with the maximal concentration of agonist (Fig. 1). There was also no difference in the sinusoidal [Ca2+]i oscillation frequency induced by 0.5 µM carbachol between the cell types (Fig. 3D). These results indicate that the reduction in SERCA2 expression did not affect overall Ca2+ signaling in SERCA2+/- mouse parotid acinar cell, which is different from that observed in SERCA2+/- mouse pancreatic acinar cells. Parotid acinar cells show a sinusoidal type of [Ca2+]i oscillations which is dependent on high Ca2+ entry.20 In contrast, pancreatic acinar cells evoke repetitive baseline typed [Ca2+]i oscillations, not dependent on Ca2+ entry.21 It is possible that the high Ca2+ entry in parotid gland acinar cells, compared to pancreatic cells, might explain the discrepancy in the findings between the cell types. It is also possible that other Ca2+-dependent physiological functions undergo different adaptations of PMCA.
We also found that the expression levels of IP3Rs in parotid gland acinar cells were different from these in SERCA2+/- mice (Figs. 1A and B): They have not been examined before in pancreatic cells from SERCA2+/- mice. There are 3 isoforms of IP3Rs, and each has a different affinity for IP322 and distinct functional properties at the single channel level.23 Most cells express 2 or all 3 IP3Rs isoforms.24 The presence of 3 isoforms of the IP3Rs in parotid acinar cells indicates that each isoform may also have a distinct effect on Ca2+ signaling. In the present study, we found higher expression of IP3R1 and IP3R3 and lower expression of IP3R2 in SERCA2+/- cells. Presently, however, it is not known how a partial decrease of SERCA2 affects the expression of each IP3R. It has been reported that IP3R3 and many other Ca2+ signaling-related proteins in rat pancreatic acinar cells co-localize with each other and have protein-protein interactions.25 Therefore, 1 possibility is that a partial loss of SERCA2 may affect the protein-protein interaction between Ca2+ signaling complexes, thereby functionally affecting the expression levels of IP3Rs in parotid acinar cells. However, changes in IP3Rs, PMCA, and SERCA expression levels did not affect their location in SERCA2+/- cells (Fig. 1C and data not shown, respectively) and the activity of IP3Rs was not changed (Figs. 2A and B). Since IP3 receptors are formed as heterotetrameric complexes,26 it seems likely that changes in IP3Rs expression levels did not affect their activity in SERCA2+/- mice.
The important finding of this work is that Ca2+-dependent amylase secretion by muscarinic stimulation was more sensitive to the levels of agonist in SERCA2+/- mice (Fig. 4B), although total salivary secretion by pilocarpine (Fig. 4A) or cAMP-dependent amylase secretion by the stimulation of isoproterenol (data not shown) was not affected by partial loss of SERCA2. There are 3 possible explanations as to why this occurred. The SERCA2+/- mice may be better able to maintain Ca2+ levels during long agonist stimulation than WT mice. There may be a difference in the amount of amylase present in the WT and SERCA2+/- mice, or there may be a change in Ca2+-dependent amylase secretion-related proteins in the SERCA2+/- mice. After examining all 3 of these possibilities, we found no significant difference in Ca2+-sustaining capacity between the both types of cells (Fig. 4C). In addition, there was no change in the amylase contents in secretory vesicles (Fig. 4D). Because differences in the amounts of amylase secretion amounts depend on Ca2+, we assessed changes of protein expression related to Ca2+-dependent amylase secretion with Western blot to examine all synaptotagmin isotypes, and found that the expression of synaptotagmin was increased in SERCA2+/- mice (Figs. 4C and D). In contrast, the amount of syntaxin-2, potentially related to amylase secretion in parotid acinar, was not significantly changed in SERCA2+/- parotid acinar cells (Fig. 4D). Synaptotagmin is known to be a Ca2+ sensor for Ca2+ dependent neurotransmitter release,19 however its role in non-neuronal cells is still unclear. Nevertheless, increased expression of synaptotagmin may explain in part the agonist-sensitive amylase secretion in SERCA2+/- mice. On the other hand, a recent study showed that mitogen-activated protein kinase (MAPK)/extracellular signal regulated kinase (ERK) expression was changed in human embryonic kidney 293 cells in which SERCA was inhibited by thapsigargin, suggesting that decreases in SERCA activity causes changes in MAPK pathway.27 Therefore, it is possible that this signaling cascade may play a role in synaptotagmin expression.
Salivary function as well as pancreatic function appears to be normal in DD patients, and our present work demonstrates by using SERCA2+/- parotid gland acinar cells that the overall Ca2+ signaling from agonist stimulation adapts to the reduction of SERCA2 by upregulation of PMCA, except in the case of Ca2+-dependent amylase secretion and its related protein expression. Since [Ca2+]i is involved in so many cellular functions, it is reasonable to assume that Ca2+ signaling is adapted to agonist-mediated response in SERCA2+/- parotid gland acinar cells.
Figures and Tables
Fig. 1
Western blotting and immunofluorescence in parotid gland acinar cells of SERCA2+/- mouse. (A and B) for Western blots in A, parotid acini were collected from 3 wild-type and 3 SERCA2+/- mice with density gradient centrifugation, and proteins were extracted. Note that a partial loss of SERCA2b upregulated the expressions of PMCA and IP3R1, and IP3R3. (C) Confocal images of immunolocalization of IP3R1, IP3R2, and IP3R3 in parotid acinar cells from SERCA2+/- and WT mice. A partial loss of SERCA2b did not affect the localization of IP3Rs. A scale bar indicates µm.
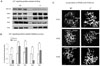
Fig. 2
Measurement of SERCA, IP3Rs, and PMCA activity in parotid gland acinar cells of SERCA2+/- mouse. (A and B)
To directly measure Ca2+ uptake and release from internal stores, parotid acini from WT or SERCA2+/- mice were permeabilized with SLO-containing medium. Where indicated by the arrow, the cells were stimulated with 1 µM IP3 and 1 mM carbachol. (C and D) Intact parotid acini from WT and SERCA2+/- were added to a Ca2+-free, high K+ solution containing 7.5 mM EGTA and 2 µM fura-2 free acid form. Where indicated by the arrow, the cells were stimulated with 1 mM carbachol to measure the activity of PMCA.
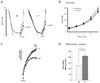
Fig. 3
Measurements of Ca2+ response in parotid gland acinar cells of SERCA2+/- mouse. (A) Cells from WT and SERCA2+/- mice were stimulated with 1 mM of carbachol in PSS solution. The cells were washed in nominally Ca2+-free medium. After reduction of [Ca2+]i to a basal level, the cells were incubated in PSS containing 1 mM CaCl2 and 1 µM thapsigargin to induce Ca2+ influx. (B) Cells from WT or SERCA2+/- mice were incubated in Ca2+ medium prior to stimulation with 1 mM carbachol. After reduction of [Ca2+]i to a basal level, the cells were perfused with solution containing 1 mM CaCl2 and 1 µM thapsigargin to induce Ca2+ influx. (C) Cells from WT or SERCA2+/- mice were incubated in 1 mM Ca2+ medium and thapsigargin was added prior to stimulation with 1 mM carbachol to measure the amounts of Ca2+ER. (D) Frequency of [Ca2+]i oscillations evoked by 3 µM carbachol in Ca2+-medium.
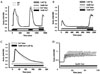
Fig. 4
Measurements of Ca2+-dependent salivary and amylase secretion and expression level of the Ca2+-dependent secretion-related proteins in parotid gland acinar cells of SERCA2+/- mouse. (A and B) Comparison of saliva flow rate and amylase release between WT and SERCA2+/-. (C) Intracellular Ca2+ responses when cells were stimulated with 1 µM, 3 µM, and 100 µM carbachol for 20 min. (D and E) Alterations in expression levels of the Ca2+- dependent secretion-related proteins in parotid acinar cells from WT and SERCA2+/- mice.
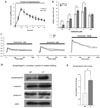
References
2. Takuma T, Ichida T. Evidence for the involvement of protein phosphorylation in cyclic AMP-mediated amylase exocytosis from parotid acinar cells. FEBS Lett. 1994. 340:29–33.


3. Sugiya H, Furuyama S. The activation of Ca2+ mobilizing receptors in salivary gland. Biomed Res. 1989. 10:111–121.
4. Berridge MJ, Bootman MD, Roderick HL. Calcium signalling: dynamics, homeostasis and remodelling. Nat Rev Mol Cell Biol. 2003. 4:517–529.


5. Kiselyov K, Shin DM, Muallem S. Signalling specificity in GPCR-dependent Ca2+ signalling. Cell Signal. 2003. 15:243–253.
6. East JM. Sarco(endo)plasmic reticulum calcium pumps: recent advances in our understanding of structure/function and biology (review). Mol Membr Biol. 2000. 17:189–200.


7. Bolotina VM. Store-operated channels: diversity and activation mechanisms. Sci STKE. 2004. 243:pe34.


8. Lee MG, Xu X, Zeng W, Diaz J, Wojcikiewicz RJ, Kuo TH, et al. Polarized expression of Ca2+ channels in pancreatic and salivary gland cells. Correlation with initiation and propagation of [Ca2+]i waves. J Biol Chem. 1997. 272:15765–15770.


9. Anwar A, Taimor G, Korkususz H, Schreckenberg R, Berndt T, Abdallah Y, et al. PKC- independent signal transduction pathways increase SERCA2 expression in adult rat cardiomyocytes. J Mol Cell Cardiol. 2005. 39:911–919.


10. Verboomen H, Wuytack F, Van den Bosch L, Mertens L, Casteels R. The functional importance of the extreme C-terminal tail in the gene 2 organellar Ca2+-transport ATPase (SERCA 2a/b). Biochem J. 1994. 303:979–984.


11. Periasamy M, Reed TD, Liu LH, Ji Y, Loukianov E, Paul RJ, et al. Impaired cardiac performance in heterozygous mice with a null mutation in the sarco(endo)plasmic reticulum Ca2+-ATPase isoform 2 (SERCA2) gene. J Biol Chem. 1999. 274:2556–2562.


12. Prasad V, Okunade GW, Miller ML, Shull GE. Phenotypes of SERCA and PMCA knockout mice. Biochem Biophys Res Commun. 2004. 322:1192–1203.


13. Zhao XS, Shin DM, Liu LH, Shull GE, Muallem S. Plasticity and adaptation of Ca2+ signaling and Ca2+- dependent exocytosis in SERCA2+/- mice. EMBO J. 2001. 20:2680–2689.


14. Ruiz-Perez VL, Carter SA, Healy E, Todd C, Rees JL, Steijlen PM, et al. ATP2A2 mutations in Darier's disease: variant cutaneous phenotypes are associated with missense mutations, but neuropsychiatric features are independent of mutation class. Hum Mol Genet. 1999. 9:1621–1630.
15. Bernfeld P. Enzymes of starch degradation and synthesis. Adv Enzymol Relat Subj Biochem. 1951. 12:379–428.


16. Shin DM, Luo X, Wilkie TM, Miller LJ, Peck AB, Humphreys-Beher MG, et al. Polarized expression of G protein-coupled receptors and an all-or-none discharge of Ca2+ pools at initiation sites of [Ca2+]i waves in polarized exocrine cells. J Biol Chem. 2001. 276:44146–44156.


17. Zhang BX, Muallem S. Feedback inhibition of Ca2+ release by Ca2+ is the underlying mechanism of agonist-evoked intracellular Ca2+ oscillations in pancreatic acinar cells. J Biol Chem. 1992. 267:24387–24393.


18. Ji Y, Lalli MJ, Babu GJ, Xu Y, Kirkpatrick DL, Liu LH, et al. Disruption of a single copy of the SERCA2 gene results in altered Ca2+ homeostasis and cardiomyocyte function. J Biol Chem. 2000. 275:38073–38080.


19. Chapman ER. Synaptotagmin: A Ca2+ sensor that triggers exocytosis? Nat Rev Mal Cell Biol. 2002. 3:498–508.
20. Foskett JK, Roifman CM, Wong D. Activation of calcium oscillations by thapsigargin in parotid acinar cells. J Biol Chem. 1991. 266:2778–2782.


21. Thorn P, Lawrie AM, Smith PM, Gallacher DV, Petersen OH. Ca2+ oscillations in pancreatic acinar cells: spatiotemporal relationships and functional implications. Cell Calcium. 1993. 10:746–757.
22. Newton CL, Mignery GA, Südhof TC. Co-expression in vertebrate tissues and cell lines of multiple inositol 1,4,5-trisphosphate (InsP3) receptors with distinct affinities for InsP3. J Biol Chem. 1994. 269:28613–28619.


23. Hagar RE, Burgstahler AD, Nathanson MH, Ehrlich BE. Type III InsP3 receptor channel stays open in the presence of increased calcium. Nature. 1998. 396:81–84.


24. Hattori M, Suzuki AZ, Higo T, Miyauchi H, Michikawa T, Nakamura T, et al. Distinct roles of inositol 1,4,5-trisphosphate receptor types 1 and 3 in Ca2+ signaling. J Biol Chem. 2004. 279:11967–11975.


25. Shin DM, Zhao XS, Zeng W, Mozhayeva M, Muallem S. The mammalian Sec6/8 complex interacts with Ca2+ signaling complexes and regulates their activity. J Cell Biol. 2000. 150:1101–1112.

