Abstract
Purpose
This study was designed to investigate the change of peroxisome proliferator-activated receptor gamma (PPARγ) after the infection of the human coronary artery smooth muscle cells (HCSMCs) with Chlamydia pneumoniae (C. pneumoniae) and the effect of PPARγ agonist on the expression of PPARγ of C. pneumoniae-infected HCSMCs.
Materials and Methods
To determine the effect of PPARγ agonist on the proliferation of C. pneumoniae-infected HCSMCs, rosiglitazone at various concentrations was applied 1 hour before inoculation of HCSMCs.
Results
The expression of PPARγ mRNA in HCSMCs increased from 3 hours after C. pneumoniae infection and reached that of noninfected HCSMCs at 24 hours (p < 0.05). The expression of PPARγ protein in HCSMCs also increased from 3 hours after C. pneumoniae and persisted until 24 hours as compared with that of noninfected HCSMCs (p < 0.05). The pretreatment of HCSMCs with rosiglitazone followed by the infection with C. pneumoniae augmented the expression of PPARγ mRNA and protein (p < 0.05) and decreased cell proliferation.
Conclusion
Our results showed that the expression of PPARγ increases in response to C. pneumoniae infection and rosiglitazone further augmented the expression of PPARγ. It is suggested that rosiglitazone could ameliorate the chronic inflammation in the vessel wall induced by C. pneumoniae by augmenting PPARγ expression.
Infection has attracted attention as a novel risk factor for atherosclerosis, which is different from traditional risk factors.1 Among various infectious agents, Chlamydia pneumoniae (C. pneumoniae) is the major infectious agent that has been extensively investigated. Seroepidemiological studies showed that the seropositivity of anti-C. pneumoniae antibodies is two times higher in patients with cardiovascular diseases than that of healthy population controls.2 Furthermore C. pneumoniae were isolated from more than half of atherosclerotic lesions.3 In vitro studies suggest that C. pneumoniae could be detected in circulating leukocytes, and that they infect all atheroma cell types such as endothelial cells, vascular smooth muscle cells (VSMC), and macrophages,4-6 and induce the inflammatory cytokines, procoagulants, matrix metalloproteinases and adhesion molecules.7-9
Peroxisome proliferator-activated receptor-γ (PPARγ) is a member of the nuclear receptor superfamily that regulates lipid and lipoprotein metabolism and glucose homeostasis. In addition, PPARγ has been reported to be an important modulator of the inflammatory response of vessel wall not only through metabolic effects but also through their direct action in vascular and inflammatory cells.10,11 PPARγ is expressed in the major cellular constituents of the vessel wall such as endothelial cells, VSMC, and macrophages, and affects development of atherosclerosis.12,13 In endothelial cells, PPARγ agonist inhibits the expression of monocyte chemoattractant protein-1, vascular cell adhesion molecule-1, and intercellular adhesion molecule-1.14,15 PPARγ also interferes with leukocyte chemoattraction and recruitment to atherosclerotic lesions and suppresses the expression of inducible nitric oxide synthase and matrix metalloproteinase-9 (MMP-9) in macrophages. Furthermore, PPARγ inhibits VSMC proliferation by attenuating the mitogen-induced degradation of p27Kip1, and platelet-derived growth factor-induced VSMC migration by inhibiting MMP-9 expression.11,16
Although a number of studies indicate the role of PPARγ in the modulation of vascular inflammation, the effect of atheroprone infectious agents on the expression of PPARγ in vascular cells has not yet been studied. Interestingly, infection increases PPARγ expression in porcine white blood cells17 but down-regulates it in murine adipocytes,18 suggesting that PPARγ expression is differentially regulated according to cell types. We hypothesized that C. pneumoniae infection, which is a clear risk factor for the development of atherosclerosis, may have an effect on the expression of PPARγ in vascular smooth muscle cells. Therefore, the present study was undertaken to investigate whether C. pneumoniae infection could affect the expression of PPARγ in human coronary artery smooth muscle cells (HCSMC).
Human coronary artery smooth muscle cells (HCSMC) were purchased from Clonetics and cultured in SmBm media (smooth muscle cell basal media, Clonetics, Walkersville, MD, USA). Passages between 5 and 10 were used.
C. pneumoniae AR-39 strain was purchased from the American Type Culture Collection (ATCC, Manassas, VA, USA). The organisms were inoculated into shell vials containing McCoy cells (ATCC, Manassas, VA, USA), and then shell vials were centrifuged at 1500g for 1 hour and incubated in RPMI 1640 medium containing 1 µg/mL cycloheximide (Sigma, St. Louis, MO, USA). After 48 hours, the infected cells were disrupted by ultrasonication, and culture supernatants and cell debris were removed by centrifugation at 800g for 10 minutes. The organisms were then concentrated by a high-speed centrifugation at 30,000g for 30 minutes. The bacterial pellets were resuspended in sucrose phosphate glutamate transport medium and stored at -70℃ until use. The organisms resuspended in RPMI 1640 medium were used for experiments. The number of infectious C. pneumoniae was determined as inclusion forming units per mL by counting chlamydial inclusions formed in McCoy cells with fluorescein isothiocyanate-conjugated C. pneumoniae-specific monoclonal antibody (DAKO, Copenhagen, Denmark).
HCSMCs were trypsinized and plated at a density of 4 × 105 cells/well on 6-well plates. Once confluent, the medium was then changed to serum-free medium for 24 hour to achieve synchronous growth arrest. HCSMCs were inoculated with C. pneumoniae at a multiplicity of infection (MOI) of 2, and the plates were centrifuged at 500g for 1 hour at room temperature. Multiple inclusion bodies in HCSMCs were observed after 48 hours of C. pneumoniae infection.
HCSMCs were plated at a density of 5 × 103 cells/well on 96-well plates and inoculated with C. pneumoniae by the method described above. Cell proliferation was determined using XTT proliferation assay kit (JBI, Deagu, Korea) 6 hours, 24 hours and 48 hours after inoculation. As a control for cell proliferation, noninfected HCSMCs were used. To determine the effect of a PPARγ agonist on the proliferation of C. pneumoniae-infected HCSMCs, rosiglitazone (GlaxoSmithKline Corporate, King of Prussia, PA, USA) at various concentrations was applied 1 hour before inoculation.
Total RNA was extracted from HCSMCs using TRIZOL Reagent (Invitrogen, Carlsbad, CA, USA) according to the manufacturer's instructions. Total extracted RNA was subjected to reverse transcription using an onestep RT kit (Invitrogen). Expressions of PPARγ and glyceraldehyde 3-phosphate dehydrogenase (GAPDH) were examined in real time PCR using an SYBR Green dye. Primers used were as follows: human PPARγ sense, 5'-TCTCTC CGTAATGGAAGACC-3', human PPARγ antisense, 5'-CCCCTACAGAGTATTACG-3'; GAPDH sense, 5'-TGCACCACCAACTGCTTAGC-3', GAPDH antisense 5'-GGCATGGACTGTGGTCAT GAG-3'. To examine the changes in the expression of PPARγ mRNA according to time elapse after C. pneumoniae infection in HCSMCs, relative values were compared at various time intervals.
HCSMCs were seeded at 4 × 105 cells/well in 6-well plates and incubated with C. pneumoniae. After various time intervals, cells were harvested,lysed, and sonicated in Tris-lysate buffer [20 mM Tris-HCl (pH 7.4), 5 mM EDTA, 10 mM Na2P2O7, 100 mM NaF, 2 mM Na3VO4, 1 mM phenylmethylsulfonyl fluoride, 10 µg/mL aprotinin, 10 µg/mL leupeptin]. Cell lysate were cleared by centrifugation, and protein concentrations were determined by Bradford protein assay kit (Pierce, Rockford, IL, USA). Proteins were resolved on a 9% sodium dodecylsulfate-polyacrylamide gel, and electrophorectically transferred onto a nitrocellulose membrane. The membrane was incubated with anti-PPARγ monoclonal antibody (Santa Cruz Biotechnology, Santa Cruz, CA, USA). HRP-conjugated anti-mouse IgG (Amersham Bioscience, Piscataway, NJ, USA) was used as a secondary antibody. The antigen-antibody complexes were detected using enhanced chemilumnescence kit (Amersham Bioscience). Quantification of the protein band was done by densitometry.
Statistical analysis was performed using the Statistical Package for Social Sciences (version 10, SPSS Inc., Chicago, IL, USA). Values of continuous variables are expressed as mean ± standard deviation. Comparisons of data were made using Student t-test. P-values less than 0.05 were considered as indicative of statistical significance.
HCSMC proliferation was compared between C. pneumoniae-infected and uninfected-HCSMCs, according to time intervals such as 6, 24, and 48 hours after infection at a MOI of 2. As shown in Fig. 1, HCSMCs proliferation was stimulated after inoculation with C. pneumoniae in a time-dependent manner. At 48 hours, XTT assay showed that C. pneumoniae significantly increased the number of HCSMCs compared to the uninfected control (Fig. 1).
To explore whether PPARγ activation directly affects C. pneumoniae-induced HCSMCs proliferation, the proliferation rate was compared between C. pneumoniae-infected HCSMCs and rosiglitazone-treated C. pneumoniae-infected HCSMCs. Treatment of HCSMCs with 10 and 50 µM rosiglitazone significantly decreased the C. pneumoniae-induced proliferation of HCSMCs from 5 hours after C. pneumoniae infection and from 8 hours even at 1 µM concentration (Fig. 2).
To determine whether C. pneumoniae activates PPARγ mRNA in HCSMCs, cells were left untreated or stimulated with C. pneumoniae, and cDNA was then prepared from each HCSMCs after 3-, 6-, and 24-hour exposure to C. pneumoniae. The expression of PPARγ mRNA was evaluated by real time RT-PCR. The levels of PPARγ mRNA were significantly increased in HCSMCs 3 hours after C. pneumoniae inoculation, and remained elevated until 6 hours after infection as compared with uninfected control. At 24 hours, the level of PPARγ mRNA in HCSMCs infected with C. pneumoniae returned to the level of uninfected control (Fig. 3).
To investigate whether C. pneumoniae infection affects PPARγ protein expression in HCSMCs, cells were stimulated with C. pneumoniae infection for 3, 6, and 24 hours. Cell lysates were analyzed by Western blot using a anti-PPARγ monoclonal antibody. The levels of PPARγ protein were already increased in HCSMCs after 3-hour exposure to C. pneumoniae as compared with uninfected HCSMCs, and the status of elevation was maintained until 24 hours (Fig. 4).
The observation that C. pneumoniae has a stimulatory effect on PPARγ protein in HCSMCs prompted us to investigate the potential synergistic effect of rosiglitazone on the expression of PPARγ in HCSMCs. Thus, HCSMC cells were divided into untreated control, C. pneumoniae-inoculated, rosiglitazone (20 µM)-treated, and co-treated with both rosiglitazone and C. pneumoniae. Changes in the expression of PPARγ in each HCSMCs were evaluated by Western blot 24 hours after treatment. As compared with untreated control, both rosiglitazone and C. pneumoniae significantly increased the expression of PPARγ protein in HCSMCs. Interestingly, co-treatment of HCSMCs with rosiglitazone and C. pneumoniae resulted in an additive effect on the expression of PPARγ protein (Fig. 5).
The present study shows that C. pneumoniae could upregulate the expression of PPARγ mRNA and protein in HCSMCs, and PPARγ activator further increased its expression in concert with C. pneumoniae. In addition, rosiglitazone significantly attenuated C. pneumoniae-induced proliferation of HCSMCs. Our results suggest that PPARγ palys a role in the development of atherosclerosis induced by C. pneumoniae. It is suggested that rosiglitazone, a PPARγ agonist, could prevent the development of atherosclerosis through its anti-inflammatory and anti-atherogenic effects.
C. pneumoniae could infect and proliferate within endothelial cells, vascular smooth muscle cells, and macrophages, and evoke inflammatory response in these cells.4-9 Animal model studies have shown that C. pneumoniae could infect vessel wall and cause arterial inflammation, which leads to the initiation and development of atherosclerotic lesions.19,20 In contrast, PPARγ has an important effect of inhibiting growth and migration of all vascular cells, and reducing vessel inflammation, which leads to attenuation of atherosclerosis in the vessel wall.12,13,16,21-23 However, the influence of C. pneumoniae infection on the PPARγ in atherosclerosis-related vascular cells has not yet been studied. Since PPARγ is expressed in all vascular cells and has significant effects on the protection from vessel inflammation, it is of value to demonstrate the effect of C. pneumoniae on the PPARγ in vascular cells.
Few studies have evaluated the association between infection and PPARγ. Leininger et al. reported that in vivo lipopolysaccharide challenge caused a dynamic increase in PPARγ protein expression in peripheral white blood cells.17 In tissues of Helicobacter pylori-induced atrophic gastritis or gastric carcinoma, the expression of PPARγ was upregulated,24 and Huang B et al. showed that the expression of PPARγ was upregulated in the endothelial cells in mice infected with C. pneumoniae.25 In the present study, we also observed that C. pneumoniae stimulated the expression of PPARγ in vascular smooth muscle cells. This observation was contrary to the expectation that C. pneumoniae might suppress the expression of PPARγ because of their counter-balancing actions on all the vascular cells and vessel walls. However, the mechanisms of C. pneumoniae-induced PPARγ activation in HCSMCs are not clear. C. pneumoniae is a Gram-negative bacterium, and its cell wall is rich in lipopolysaccharides, which can induce hepatocytes and macrophages to secret prostaglandin (PG) D2.26,27 15d-PGJ2, a metabolite of PGD2, is a ligand for PPARγ and can induce the PPARγ expression. Further study is needed to elucidate in detail the mechanism of C. pneumoniae-induced PPARγ upregulation in vitro.
The increase of PPARγ expression may act as an counter-regulatory mechanism of attenuating the negative effect of C. pneumoniae in HCSMCs. Rosiglitazone suppressed C. pneumoniae-induced proliferation of HCSMCs and further elevated the expression of PPARγ protein. It might be a novel mechanism of PPARγ activators to be protective against the atheroprone C. pneumoniae. Further studies are required to clarify the association and influence between infection and PPARγ in vascular cells as well as in animal models.
Figures and Tables
Fig. 1
Cell proliferation assay of HCSMCs infected with Chlamydia pneumoniae. Cell proliferation was assessed by XTT proliferation assay kit (JBI, Daegu, Korea). Changes in cell proliferation are expressed by a percentage of the mean value of control (uninfected HCSMCs). *p < 0.05.
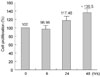
Fig. 2
Dose-dependent effects of rosiglitazone on the proliferation of HCSMCs infected with Chlamydia pneumoniae during 24 hours. *p < 0.05.
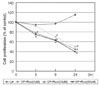
Fig. 3
Real time RT-PCR of PPARγ in HCSMCs infected with Chlamydia pneumoniae. Relative value was arbitarily defined as corrected for GAPDH mRNA value. CP represents Chlamydia pneumoniae. *p < 0.05 vs CP(-).
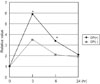
Fig. 4
Western blot analysis of PPARγ in HCSMCs infected with Chlamycia pneumoniae. After 3, 6, and 24 hours, cells were subjected to 10% SDS-PAGE and blotted with anti-PPARγ monoclonal antibody. Expression levelsof PPARγ were quantified by densitometry. *p < 0.05 vs CP(-).
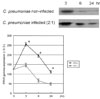
Fig. 5
Western blot analysis of PPARγ in HCSMCs infected with Chlamydia pneumoniae and/or treated with rosiglitazone (20 µM). After 24 hours, cells were subjected to 10% SDS-PAGE and blotted with anti-PPARγ monoclonal antibody. Expression levels of PPARγ were quantified by densitometry. *p < 0.05 vs CP(-).
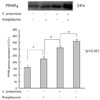
References
1. Leinonen M, Saikku P. Evidence for infectious agents in cardiovascular disease and atherosclerosis. Lancet Infect Dis. 2002. 2:11–17.


2. Danesh J, Whincup P, Walker M, Lennon L, Thomson A, Appleby P, et al. Chlamydia pneumoniae IgG titers and coronary heart disease: prospective study and meta-analysis. BMJ. 2000. 321:208–213.


3. Gibbs RG, Carey N, Davies AH. Chlamydia pneumoniae and vascular disease. Br J Surg. 1998. 85:1191–1197.
4. Gaydos CA, Summersgill JT, Sahney NN, Ramirez JA, Quinn TC. Replication of Chlamydia pneumoniae in vitro in human macrophages, endothelial cells, and aortic artery smooth muscle cells. Infect Immun. 1996. 64:1614–1620.


5. Lin TM, Campbell LA, Rosenfeld ME, Kuo CC. Monocyte-endothelial cell coculture enhances infection of endothelial cells with Chlamydia pneumoniae. J Infect Dis. 2000. 181:1096–1100.


6. Moazed TC, Kuo CC, Grayston JT, Campbell LA. Evidence of systemic dissemination of Chlamydia pneumoniae via macrophages in the mouse. J Infect Dis. 1998. 177:1322–1325.


7. Da Costa CU, Wantia N, Kirschning CJ, Busch DH, Rodriguez N, Wagner H, et al. Heat shock protein 60 from Chlamydia pneumoniae elicits an unusual set of inflammatory responses via Toll-like receptor 2 and 4 in vivo. Eur J Immunol. 2004. 34:2874–2884.


8. Dechend R, Maass M, Gieffers J, Dietz R, Scheidereit C, Leutz A, et al. Chlamydia pneumoniae infection of vascular smooth muscle and endothelial cells activates NF-Kappa B and induces tissue factor and PAI-1 expression: a potential link to accelerated arteriosclerosis. Circulation. 1999. 100:1369–1373.


9. Fryer RH, Schwobe EP, Woods ML, Rodgers GM. Chlamydia species infect human vascular endothelial cells and induce procoagulant activity. J Investig Med. 1997. 45:168–174.
10. Duval C, Chinetti G, Trottein F, Fruchart JC, Staels B. The role of PPARs in atherosclerosis. Trends Mol Med. 2002. 8:422–430.


11. Hsueh WA, Jackson S, Law RE. Control of vascular cell proliferation and migration by PPAR-gamma: a new approach to the macrovascular complications of diabetes. Diabetes Care. 2001. 24:392–397.
12. Marx N, Sukhova G, Murphy C, Libby P, Plutzky J. Macrophages in human atheroma contain PPAR gamma: differentiation-dependent peroxisomal proliferator-activated receptor gamma (PPAR gamma) expression and reduction of MMP-9 activity through PPAR gamma activation in mononuclear phagocytes in vitro. Am J Pathol. 1998. 153:17–23.
13. Ricote M, Huang J, Fajas L, Li A, Welch J, Najib J, et al. Expression of the peroxisome proliferator-activated receptor gamma (PPAR gamma) in human atherosclerosis and regulation in macrophages by colon stimulating factors and oxidized low density lipoprotein. Proc Natl Acad Sci U S A. 1998. 95:7614–7619.


14. Murao K, Imachi H, Momoi A, Sayo Y, Hosokawa H, Sato M, et al. Thiazolidinedione inhibits the production of monocyte chemoattractant protein-1 in cytokine-treated human vascular endothelial cells. FEBS Lett. 1999. 454:27–30.


15. Pasceri V, Wu HD, Willerson JT, Yeh ET. Modulation of vascular inflammation in vitro and in vivo by peroxisome proliferator-activated receptor gamma activators. Circulation. 2000. 101:235–238.


16. Marx N, Schonbeck U, Lazar MA, Libby P, Plutzky J. peroxisome proliferator-activated receptor gamma activators inhibit gene expression and migration in human vascular smooth muscle cells. Circ Res. 1998. 83:1097–1103.


17. Leininger MT, Portocarrero CP, Houseknecht KL. Peroxisome proliferator-activated receptor gamma 1 expression in porcine white blood cells: dynamic regulation with acute endotoxemia. Biochem Biophys Res Commun. 1999. 263:749–753.


18. Hill MR, Young MD, McCurdy CM, Gimble JM. Decreased expression of murine PPAR gamma in adipose tissue during endotoxemia. Endocrinology. 1997. 138:3073–3076.


19. Liu L, Hu H, Ji H, Murdin AD, Pierce GN, Zhong G. Chlamydia pneumoniae infection significantly exacerbates aortic atherosclerosis in an LDLR-/- mouse model within six months. Mol Cell Biochem. 2000. 215:123–128.
20. Moazed TC, Campbell LA, Rosenfeld ME, Grayston JT, Kuo CC. Chlamydia pneumoniae infection accelerates the progression of atherosclerosis in apolipoprotein E-deficient mice. J Infect Dis. 1999. 180:238–241.


21. Law RE, Goetze S, Xi XP, Jackson S, Kawano Y, Demer L, et al. Expression and function of PPAR gamma in rat and human vascular smooth muscle cells. Circulation. 2000. 101:1311–1318.


22. Wakino S, Kintscher U, Kim S, Yin F, Hsueh WA, Law RE. Peroxisome proliferator-activated receptor gamma ligands inhibit retinoblastoma phosphorylation and G1 →S transition in vascular smooth muscle cells. J Biol Chem. 2000. 275:22435–22441.


23. Goetze S, Xi XP, Kawano H, Gotlibowski T, Fleck E, Hsueh WA, et al. PPAR gamma-ligands inhibit migration mediated by multiple chemoattractants in vascular smooth muscle cells. J Cardiovasc Pharmacol. 1999. 33:798–806.


24. Konturek PC, Kania J, Konturek JW, Nikiforuk A, Konturek SJ, Hahn EG. H. pylori infection, atrophic gastritis, cytokines, gastrin, COX-2, PPAR gamma and impaired apoptosis in gastric carcinogenesis. Med Sci Monit. 2003. 9:SR53–SR66.
25. Huang B, Dong Y, Mai W, Li Y. Effect of Chlamydia pneumoniae infection and hyperlipidaemia on the expression of PPAR gamma, P50 and c-Fos in aortic endothelial cells in C57bL/6J mice. Acta Cardiol. 2005. 60:43–49.

