Abstract
Purpose
The purpose of this study was to investigate the activation of the respiratory centers during insufflation of the larynx with CO2 at different flow rates and concentrations.
Materials and Methods
The experiments were carried out in spontaneous air breathing rabbits, anesthetized with thiopental sodium (25 mg kg-1i.v.). The larynx was separated from the oropharyngeal cavity and the trachea. The tidal volume (VT) and respiratory frequency (f min-1) were recorded from the lower tracheal cannula. The respiratory minute volume (VE) was calculated, the action potentials from the right phrenic nerve were recorded and the inspiratory (TI) and expiratory (TE) periods and the mean inspiratory flow rate (VT/TI) were calculated. The larynx was insufflated at flow rates of 500 mL min-1 and 750 mL min-1, with 7 and 12% CO2-Air by means of a respiratory pump.
Results
Insufflation of the larynx, with both gas mixtures, decreased the f and VT significantly. The TI and TE were found to increase significantly due to the decreasing in f. There was a significant decrease in VT/TI ratio. Following bilateral midcervical vagotomy, on the passing of both gas mixtures, significant decreases were observed in the VT, and the responses of f, TI and TE were abolished. After cutting the superior laryngeal nerve, the responses of the VT to both gas mixtures were abolished.
Many investigators have shown histologically that free neural plexuses, with or without myelin, could exist in the laryngeal mucosa and submucosa.1-5 Various classifications have been set forth with regard to the sensory receptors localized in the larynx. Based on the studies of San't Ambrogio et al.6 it is assumed that there are 5 different types of receptor in the larynx; these being pressure, drive, cold, irritant and C-fiber receptors.
It has been shown that the laryngeal receptors are sensitive to a wide range of physical and chemical stimulants, that various gases and aerosols stimulate the laryngeal receptors, which has also been confirmed by the potential records from the superior laryngeal nerve (SLN). Likewise, administration of CO2 at a constant flow rate through the larynx on dogs,7 cats8 and decerebrated cats9 under anesthesia stimulates the laryngeal mechanoreceptors.
It is reported that the laryngeal mechanoreceptors are sensitive to air flow as well as hypercapnic gas and reduced ventilation.1,8,10 In studies conducted on cat, it has been shown that constant CO2 administration to the upper airway inhibited respiration as a reflex and increased the motor neural activity of the upper airway muscle.11,12 Furthermore, constant pressure and/or air flow in the isolated upper airway has been shown to influence the tonus of the intrinsic laryngeal muscles and modulate the phasic respiratory activity.13
It is also suggested that the hypercapnia caused further increase in the width of the glottis for reducing inspiratory resistance, with its further narrowing during expiration, in turn preventing alveolar collapse.14 On the other hand, upper airway CO2 also enhances the laryngeal negativepressure activity during upper airway occlusion. This is why the activity of negative-pressure receptors is also dependent on the levels of CO2 occurring in the upper airway during inspiration and expiration.15 Stimulation of the laryngeal receptors by CO2 and flow changes and afferent laryngeal impulses may have important roles in the regulation of respiration and breathing patterns under pathophysiologycal conditions.
The laryngeal lumen is known to be phasically subjected to CO2, but only during expiration. Particularly under conditions where the flow rate varies, for example in the cases of hyperventilation or dyspnea, this variation or the end-tidal CO2 may influence respiration through the laryngeal receptors.
The aims of this study were to investigate whether the flow rate and concentration of the gas passing through the larynx has an influence upon the respiratory response to CO2 passage through the larynx and also if the ambient air flow rate would impose a change on the respiratory response.
For these purposes, along with the respiratory reflex responses occurring in the passage of the hypercapnic gas mixtures (7% CO2-Air, 12% CO2-Air), the central inspiratory activity and variations in the inspiration and expiration were also sought from recording of the phrenic neural action potential.
In our study, 9 New Zealand type Albino rabbits of both sexes (mean body weight 2.8 ± 0.75 kg) were anesthetized using thiopental sodium (25 mg.kg-1 i.v.). Following a lower cervical tracheotomy, a cannula caudally connected to the inspiration-expiration valve was placed in the trachea. The test animal was made to respirate through that cannula. The pharynx hyoid was then widely opened on the right rostral side of the bone, and the epiglottis slowly pulled ventrally.
To the lower part of the cricoid cartilage, a second tracheal cannula was rostrally mounted. During these operations, utmost care was taken to avoid disturbance of the afferent (superior laryngeal nerve) and efferent (recurrent laryngeal nerve) nerves.4 The right-hand and left-hand a. femoralis and v. femoralis were isolated, and a catheter placed therein. To prevent any thrombosis or clogging of the catheters during the operation, the test animals were injected i.v. with liquiemin (0.2 mL kg-1).
Through the larynxes of the test animals, two different hypercapnic gas mixture concentrations, namely ambient air (22℃) and 7% CO2-Air and 12% CO2-Air, were supplied at rates of 500 mL/min and 750 mL/min. In the meantime, the experimental animals constantly respirated normoxical ambient air through the lower tracheal cannula. Furthermore, a tracheotomy was performed and the tracheal cannula, connected to a inspiratory-expiratory valve, was inserted into the trachea. The tidal volume (VT) and respiratory frequency (f min-1) were recorded on a Grass Model 7 polygraph by means of a pneumatocograph and a Grass PT-5 volumetric pressure transducer. From these parameters, the respiratory minute volume (VE) was calculated. From the right-hand a. femoralis, the systemic arterial blood pressure was recorded using a physiological pres-sure transducer, and the mean systemic arterial pressure calculated.
A nerve to the right side was isolated in order to the record action potential from the N. Phrenicus. Under a stereomicroscope, the nerve was cut at the lower 1/3 part of the N. phrenicus neck zone at the point where the branches coming from the 5th and 6th cervical roots meet. The sleeve of the N. phrenicus, originating from the 5th root, was stripped off under microscopy. After this, the nerve was placed on a platinum electrode. In order that the nerve would preserve its activity through the testing period, the above mentioned region was stored in neutral paraffin. The electrodes were connected to the 7 P5 preamplifier of the Grass model 7 polygraph in an effort to record the action potential from the N. phrenicus. From the discharge group period of the N. phrenicus action potentials, the inspiration period (TI) was determined, while from the periods between the discharge groups the expiration period (TE) was determined. The tidal volume (VT) was divided by the inspiration period (TI) to give the mean inspiratory flow rate (VT/TI).
At the end of each phase, the PaO2, PaCO2 and pHa were determined from the blood samples received from the left femoral artery, using the AVL gas check type 937 at 37℃.
To distinguish the influences of the air flow and hypercapnic gas mixtures on the larynx of the test animals, ambient air was first passed at the defined by means of the respiratory pump. However, no variation was observed in the f (min-1), and the VT first decreased, but then reached its normal value. A decreased VT response was observed for only a few seconds (10 to 15 seconds). No meaningful variation was observed in the VE. Also, no meaningful variations were observed in the TI and TE periods. After a bilateral vagotomy, there were no changes in the respiratory parameters (Table 1).
When 7% CO2-Air was passed through the larynx of the test animals at the given pump speed, significant reductions in both the VT and f (min-1) were observed. Subject to the variation in the VT and f (min-1), the VE also realized a significant reduction. In accordance with the frequency reduction, significant extensions were observed in the TI and TE values, while the VT/TI rate revealed a significant reduction (Table 2).
After a bilateral midcervical vagotomy, on passing 7% CO2-Air through the larynx, a significant decrease was again observed in the VT, as seen in the control group. In contrast, the reduction observed in the frequency was fully eliminated. Also, no significant variation was seen in the TI and TE periods, but the VT/TI realized a significant reduction (Table 2).
Following a bilateral vagotomy and bilateral cutting of the SLN, the 7% CO2-Air mixture was passed, and the reduction previously observed in the VT was completely eliminated, although no variation was observed in the TI and TE periods (Table 2).
When the hypercapnic gas mixture, consisting of 12% CO2-Air was passed through the larynx, at a pumping rate of 500 mL min-1, significant reductions were observed in the VT and f (min-1), and therefore in the VE. Meaningful extensions in TI and TE were observed, while the VT/TI rate again was significantly reduced (Table 2).
After a bilateral midcervical vagotomy, when 12% CO2-Air was passed through the larynx, a significant decrease was again observed in the VT, as seen in the control group. On the other hand, the reduction observed in the frequency was abolished. Therefore, no significant variation was seen in the TI and TE periods, but the VT/TI realized a significant reduction (Table 2).
After bilateral cutting of the SLN, the reduction in VT was eliminated, and again no change observed in the TI and TE periods (Table 2).
When the pumping speed in Table 4 was 500 mL min-1, the percentage changes were seen depending on the air phase in the determined parameters on passing of the two hypercapnic gases. When 12 and 7% CO2-Air were passed through the larynx at the same rate, the percentage change in the reductions observed in the VT and VE were more significant with the 12% CO2-Air compared with the that of the 7% CO2-Air.
When 7% CO2-Air was passed through the larynx of the test animals at the given pump speed (750 mL/min), again significant reductions were observed in the VT and f (min-1), and therefore in the VE. Significant extensions were observed in the TI and TE periods, in agreement with the frequency reduction. The VT/TI ratio was also significantly decreased (Table 3).
Following a bilateral midcervical vagotomy, there was a significant decrease in the VT, as seen in the control group. The frequency reduction was completely eliminated, and no changes observed in the TI and TE periods. The VT/TI ratio again underwent a significant reduction (Table 3). After a vagotomy, by means of bilateral cutting of the SLN, the reduction in VT was completely eliminated, and no variations observed in the TI and TE period (Table 3).
When 12% CO2-Air was passed through the larynx at the same pumping speed (750 mL min-1), significant reductions were observed in the f (min-1) VT and VE. The extensions in TI and TE were also found to be significant. A significant reduction was observed in the VT/TI ratio (Table 3).
A bilateral midcervical vagotomy again produced a significant decrease in the VT and VT/TI ratio and eliminated the reduction in f (min-1). No meaningful variation was observed in the TI and TE periods.
After a vagotomy and bilateral cutting of the SLN, the reduction in the VT formed in response to hypercapnia was completely eliminated. No significant variation was observed in the TI and TE periods (Table 3).
To see the influence of differing CO2 concentrations on the parameters at the same pumping speed, the percentages in the variations were calculated (Table 4). When a hypercapnic gas mixture, consisting of 12% CO2-Air, was passed through the larynx at a pump rate of 750 m/min, the percentage changes in the reduction observed in the VT and VE were much higher with the 12 than the 7% CO2-Air mixture (Table 4).
On passing both hypercapnic gas mixtures throught the larynx, significant changes in the PaO2, PaCO2 and pHa values were not observed (Table 5).
The laryngeal mechanoreceptors are sensitive to pressure and/or flow, with rapid or slow adapting response patterns.1,2 They generally increase their activities through inspiration in response to the respiratory movements of the larynx.16 Intralaryngeal CO2 reduces ventilation as a reflex and increases the upper airway muscular activity.1,8-10,16-19
In our study, before passing the gas mixtures consisting of 7% and 12% CO2-Air directly through the larynx, 500 mL min-1 and 750 mL min-1 ambient air were passed for the purpose of determining the influence of the air flow on ventilation. In doing so, the influences of the air flow and hypercapnic gas mixtures on the larynx were distinguished. As seen from our findings, when ambient air was passed through the larynx, the reduction in the tidal volume recovered to its normal value in a short time. No significant variation was observed in the respiration frequency. While the bilateral vagotomy did not influence the short time reduction in the VT, cutting the SLNs bilaterally eliminated this response. As no short time reduction was observed in the VT by means of cutting the afferent nerves of the larynx, this is evidence that the response is through the laryngeal mechanoreceptors. Likewise, in rabbits, it is observed that the laryngeal mechanosensitive endings increased their discharges through stress, while such discharges were reduced by collapse.18,20 Our finding shows that the mechanoreceptors adapt the ambient air passage very quickly.
In our study, the TI and TE periods were observed to be extended, with reductions in the respiration frequency and volume while passing both hypercapnic gases through the larynx at a rate of 500 mL min-1. When the mean inspiratory flow rate (VT/TI) was calculated, this value was considerably decreased when passing both hypercapnic gases through the larynx. In such a case, both the hypercapnic gas mixtures can be said to have passed through the larynx, which inhibit the ventilation by means of reducing the central inspiratory activity. Because the inspiration period gets longer, the central inspiratory activity takes a long time, but as the rising rate is low, it can reach a relatively lower value. The reduced tidal volume also supports this finding.
Boushey et al.8 administered three different concentrations, namely 5%, 10% and 30% CO2-Air mixtures, to the anesthetized and decerebrated cats at the larynx with a syringe, and observed that 5 and 10% CO2 reduced the minute volume by only slowing down the respiration. A biphasic response occurred against 30% CO2, first the respiration frequency and volume reduced, and after 2 to 10 respiratory cycles, the tidal volume was restored to its control level, although the respiratory frequency remained lower.
In our finding, the reductions in ventilation when passing 7 and 12% CO2 were both associated with the reductions in the tidal volume and respiratory frequency.
In other studies, it has been demonstrated that ipsilateral SLN stimulation caused an interruption of the activity in the dorsal respiratory group neurons for a short time.21-23 Furthermore, following the SLN stimulation, it has been reported that inhibitor responses were formed on the soma of inspiratory laryngeal motor neuron, while exitator responses occurred on the axon.21 SLN stimulation is reported to have strong inhibitions on phrenic neural discharge and hyperpolarization on the phrenic motor neural membrane potentials.24,25 In light of these data, the reduction in the central inspiratory activity is due to the influence of the direct laryngeal afferent impulses on the respiration centers.
In our findings, it was surprising that the respiratory frequency did not change in passing both hypercapnic gas mixtures through the larynx following the bilateral cervical vagotomy. The vagotomy eliminated the inhibitory effect of CO2 on the respiration frequency. A cervical vagotomy also eliminated the efferent impulses that would reach the larynx through the laryngeal nerve. Van Lunteren et al.26 set forth that the activity of the upper airways was modulated by a feedback arranged through vagal means, which had a greater value than that of the phrenic nerve and diaphragm. Apparently, the vagal fibers are very significant for upper airway modulation and adjustment of the respiratory rhythm.27 It can be thought that its mechanoreceptors had a vagal innervation, which are stimulated by CO2 and thereby affected the frequency.
On the other hand, after cutting the afferent nerves of the larynx following a vagotomy, the variation in the respiratory volume was also eliminated, which shows that the receptors stimulated by CO2 and inhibiting the VT had sent their impulses to the center through the superior laryngeal nerves.1,2
Bradford et al.15 have shown that stimulation of the laryngeal receptors by 5 and 9% CO2 caused reflex influences on the ventilation and upper airway muscular activity, but there was no significant difference between the responses caused by the constant administration of the differing concentrations of CO2 or during expiration. Our findings show that the increasing hypercapnic gas concentration, passing at the same flow rate, particularly support the reduction in the VT; and therefore in the VE. When the mean inspiratory flow rates of the 7 and 12% CO2-Air mixtures were compared, the decrease was greater with the 12% CO2-Air mixture. So far, no study has realized how the mechanoreceptors are stimulated by CO2. However, it can be said that the greater the CO2 concentration, the less the central inspiratory activity.
Stella et al.28 studied the influence of negative pressure, inspiration and the expiratory flow at positive pressure and expiration on the airway of the inspiratory flow. These investigations have shown that the tidal volume and diaphragmatic and abdominal muscular activities were not affected by the upper airway flow and/or pressure at ambient and body temperatures, and that the total respiratory time and expiration time had very minor increases.
In our study, the respiratory frequency decreased due to the significant increases in the TI and TE periods, as estimated from the direct phrenic activity. Therefore, it can be said that the intralaryngeal CO2 influenced the respiratory rhythm through the organization of the laryngeal receptors.
In their study, Bartlett et al.9 recorded the single fiber afferent activity from the superior laryngeal nerve when 3.5 and 10% CO2 were passed at a constant flow rate in anesthetized and paralyzed cats. They set forth that the discharge frequency of 48 out of the 53 receptors were decreased by increasing CO2 concentrations. They also showed that only 5 receptors were stimulated by intralaryngeal CO2. As a result, they stated that the reflex responses caused by CO2 were associated with the weakening of the laryngeal receptor activity and the variation in the afferent impulse discharge that commence from the larynx due to ventilatory inhibition.
It is a well known fact that in rabbits the laryngeal mechanosensitive endings change their discharges through negative and positive pressures1,29 Therefore, when the pumping speed was increased in order to determine the relation between the straining of the larynx and the concentration of the hypercapnic gas passing through, it was seen that at low hypercapnic gas concentrations, increasing the flow rate reduced the ventilation inhibition, but conversely, under higher CO2 concentrations, increasing the flow rate in turn increased the ventilation inhibition.
Apparently, the greater the flow rate, the less the unit time needed for lower concentrations of hypercapnic gas to affect the receptors. Besides, the influence in the unit time gets stronger with increasing concentration.
According to our findings, the air passage CO2 concentration and flow changes play important roles in the reflex regulation of ventilation by stimulating the laryngeal mechanoreceptors. Under the pathophysiology conditions of the upper airways, and in the application of non-invasive mechanical ventilation, stimulation of the laryngeal afferents can produce important reflex effects on the respiratory regulation and upper airway patency. In fact, during non-invasive mechanical ventilation (NIMV) the laryngeal mechanoreceptors could be stimulated by the affect of flow and CO2 concentration changes. With NIMV the ventilator forces air through the vocal cords, which are well known to widen during spontaneous inspiration. During NIMV the substantial turbulence occurring in this area strongly stimulates the laryngeal mechanoreceptors and decreases ventilation. As a matter of fact, apnea could occur following mechanical ventilation due to the affect of the flow.30
Our findings show that local laryngeal hypercapnia inhibits the central inspiratory activity by means of a reflex pathway; and furthermore, the increases in both the hypercapnic gas concentration and in the passage speed, produce stronger reflex inhibition in the ventilation. It is for this reason that, although there may be other factors involved, our findings indicate that the inhibition of the central inspiratory activity caused by the increase in both the hypercapnic gas concentration and the flow rate may play a role in apnea after NIMV.
Figures and Tables
Table 1
Effects of Ambient Air at 500 mL min-1 and 750 mL min-1 Passing through the Larynx on the Respiratory Parameters in the Indicated Experimental Phases (means ± SE)
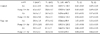
Table 2
Effects of 7 and 12% CO2-Air Mixtures Passing through the Larynx on the Respiratory Parameters in the Indicated Experimental Phases (pump rate 500 mL min-1) (means ± SE)
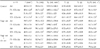
Table 3
Effects of 7 and 12% CO2-Air Mixtures Passing through the Larynx on the Respiratory Parameters in the Indicated Experimental Phases (pump rate 750 mL min-1) (means ± SE)
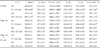
References
1. Widdicombe JG. Reflex from the upper respiratory tract. Handbook of Physiology Section 3: The respiratory system Vol II Control of Breathing: Part I Chapter II. 1986. Bethesda: USA: American Physiological Society;363–394.
3. Kirchner JA, Wyke B. Afferent discharges from laryngeal articular mechanoreceptors. Nature. 1965. 205:86–87.


4. Kirchner JA, Wyke BD. Articular reflex mechanisms in the larynx. Ann Otol Rhinol Laryngol. 1965. 74:749–768.
5. Storey AT. A functional analysis of sensory units innervating epiglottis and larynx. Exp Neurol. 1968. 20:366–383.


6. Sant'Ambrogio G, Tsubone H, Sant'Ambrogio FB. Sensory information from the upper airway: role in the control of breathing. Respir Physiol. 1995. 102:1–16.
7. Anderson JW, Sant'Ambrogio FB, Orani GP, Sant'Ambrogio G, Mathew OP. Carbon dioxide-responsive laryngeal receptors in the dog. Respir Physiol. 1990. 82:217–226.


8. Boushey HA, Richardson PS. The reflex effects of intralaryngeal carbon dioxide on the pattern of breathing. J Physiol. 1973. 228:181–191.


9. Bartlett D Jr, Knuth SL. Responses of laryngeal receptors to intralaryngeal CO2 in the cat. J Physiol. 1992. 457:187–193.
10. Szereda-Przestaszewska M, Paulev PE, Pokorski M. Hypoventilatory effects of increased CO2 in the laryngeal airway. Eur Respir J. 1992. 5:457S.
11. Bartlett D Jr, Knuth SL, Leiter JC. Alteration of ventilatory activity by intralaryngeal CO2 in the cat. J Physiol. 1992. 457:177–185.
12. Nolan P, Bradford A, O'Regan RG, McKeogh D. The effects of changes in laryngeal airway CO2 concentration on genioglossus muscle activity in the anaesthetized cat. Exp Physiol. 1990. 75:271–274.


13. Sant'Ambrogio FB, Mathew OP, Clark WD, Sant'Ambrogio G. Laryngeal influences on breathing pattern and posterior cricoarytenoid muscle activity. J Appl Physiol. 1985. 58:1298–1304.
14. Adachi T, Umezaki T, Matsuse T, Shin T. Changes in laryngeal muscle activities during hypercapnia in the cat. Otolaryngol Head Neck Surg. 1998. 118:537–544.


15. Bradford A, McKeogh D, O'Regan RG. Laryngeal-receptor responses to phasic CO2 in anesthetized cats. J Appl Physiol. 1998. 85:1135–1141.
17. Wang ZH, Bradford A, O'Regan RG. Effects of CO2 and H+ on laryngeal receptor activity in the perfused larynx in anaesthetized cats. J Physiol. 1999. 519:591–600.


18. Boushey HA, Richardson PS, Widdicombe JG, Wise JC. The response of laryngeal afferent fibres to mechanical and chemical stimuli. J Physiol. 1974. 240:153–175.


19. McBride B, Whitelaw WA. A physiological stimulus to upper airway receptors in humans. J Appl Physiol. 1981. 51:1189–1197.


20. Angell-James JE, Daly MB. Some aspects of upper respiratory tract reflexes. Acta Otolaryngol. 1975. 79:242–252.


21. Barillot JC, Bianchi AL, Gogan P. Laryngeal respiratory motoneurones: morphology and electrophysiological evidence of separate sites for excitatory and inhibitory synaptic inputs. Neurosci Lett. 1984. 47:107–112.


22. Donnelly DF, Sica AL, Cohen MI, Zhang H. Effects of contralateral superior laryngeal nerve stimulation on dorsal medullary inspiratory neurons. Brain Res. 1989. 505:149–152.


24. Bellingham MC, Lipski J, Voss MD. Synaptic inhibition of phrenic motoneurones evoked by stimulation of the superior laryngeal nerve. Brain Res. 1989. 486:391–395.


25. Jodkowski JS, Berger AJ. Influences from laryngeal afferents on expiratory bulbospinal neurons and motoneurons. J Appl Physiol. 1988. 64:1337–1345.


26. van Lunteren E, Strohl KP, Parker DM, Bruce EN, Van de Graaff WB, Cherniack NS. Phasic volume-related feedback on upper airway muscle activity. J Appl Physiol. 1984. 56:730–736.


27. Lewis J, Bachoo M, Polosa C, Glass L. The effects of superior laryngeal nerve stimulation on the respiratory rhythm: phase-resetting and after effects. Brain Res. 1990. 517:44–50.


28. Stella MH, England SJ. Modulation of laryngeal and respiratory pump muscle activities with upper airway pressure and flow. J Appl Physiol. 2001. 91:897–904.

