Abstract
Purpose
Osteoprotegerin (OPG), a potent inhibitor of osteoclastic bone resorption, has a variety of biological functions that include anti-inflammatory effects. Adipocytes and osteoblasts share a common origin, and the formation of new blood vessels often precedes adipogenesis in developing adipose tissue microvasculature. We examined whether OPG is secreted from adipocytes, therefore contributing to the prevention of neovascularization and protecting the vessels from intimal inflammation and medial calcification.
Materials and Methods
The mRNA expression of OPG and receptor activator of NF-κB ligand (RANKL) was measured in differentiated 3T3L1 adipocytes and adipose tissues.
Results
OPG mRNA expression increased with the differentiation of 3T3L1 adipocytes, while RANKL expression was not significantly altered. OPG mRNA was expressed at higher levels in white adipose tissue than in brown adipose tissue and was most abundant in the epididymal portion. In differentiated 3T3L1 adipocytes, Rosiglitazone and insulin reduced the OPG/RANKL expression ratio in a dose- and time-dependent manner. In contrast, tumor necrosis factor-α (TNF-α) increased the expression of both OPG and RANKL in a time-dependent manner. The OPG/RANKL ratio was at a maximum two hours after TNF-α treatment and then returned to control levels. Furthermore, OPG was abundantly secreted into the media after transfection of OPG cDNA with Phi C31 integrase into 3T3L1 cells.
Conclusion
Our results indicate that OPG mRNA is expressed and regulated in the adipose tissue. Considering the role of OPG in obesity-associated inflammatory changes in adipose tissue and vessels, we speculate that OPG may have both a protective function against inflammation and anti-angiogenic effects on adipose tissue.
Osteoprotegerin (OPG), an anti-inflammatory protein derived from osteoblasts, decreases bone resorption by inhibiting the differentiation and activation of osteoclasts.1,2 The receptor activator of NF-κB ligand (RANKL) is highly expressed by T-cells in lymphoid tissue and osteoblast and stromal cells in bone, especially in areas undergoing active bone remodeling or inflammatory osteolysis.2 Since the discovery of RANKL, the receptor activator of NF-κB (RANK), and the OPG system, much has been learned about the developmental, homeostatic, and pathologic roles that these molecules have in controlling cell recruitment, differentiation, and apoptosis in skeletal and immune biology.2-4 Interest in the RANKL/RANK/OPG system has been further heightened by the discovery that it may mediate important and complex linkages between the vascular, skeletal, and immune systems.5,6
Vascular calcification is triggered by active processes involving inflammatory cytokines, other signals, or by disordered calcium and phosphate homeostasis.7 Like most biological processes, vascular calcification is subject to complex positive or negative regulatory networks.6,7 Recent research on a newly discovered group of molecules belonging to the tumor necrosis factor-related family has suggested that RANK, RANKL, and OPG may have unexpected roles in vascular biology and pathophysiology.6 In OPG knockout mice, two-thirds of the mice have unexpected late medial calcification of the renal and aortic arteries, which are sites of abundant endogenous OPG expression in normal animals.3,8 Calcified arteries in OPG knockout mice also express RANKL and RANK, but these proteins are undetectable in normal murine arteries.3,8 Systemic OPG delivery in OPG knockout mice from mid-gestation through adulthood prevented vascular calcification, whereas transient OPG administration failed to reverse arterial mineralization once it had occurred.7,8 In studies with rats, OPG administration prevented calcification induced by warfarin or high doses of vitamin D.9 In humans, plasma OPG levels were elevated in patients with type 2 diabetes, coronary artery diseases, hypothyroidism, hypercholesterolemia, or abdominal obesity, as well as in aging men.10-14 These findings suggest that the RANKL/RANK/OPG system may be involved in regulating vascular calcification in humans.
Angiogenesis, the development of new blood vessels from pre-existing endothelium, is a critical process for many physiological and pathological conditions including embryonic development, organ regeneration, chronic inflammation, and solid tumor growth.15 Recently, Min et al. reported that RANKL is involved in bone formation and cartilage destruction in a rat adjuvant arthritis model.8 Nakashima et al. showed that RANKL expression can be regulated in stromal cells by a variety of cytokines generated by tumor-associated macrophages.16 Furthermore, another study found that the inflammatory cytokines tumor necrosis factor-α (TNF-α) and interleukin-1α (IL-1α) elevate RANKL expression in human microvascular endothelial cells.17 Together, these findings suggest that RANKL plays a role in the formation of microvessels at both tumor and inflammatory sites.
In the search for novel anti-obesity drugs, Rupnick et al. recently speculated that neovascularization may be critical for adipose tissue growth.18 By using various mouse models of obesity and the unique features of the microvasculature within adipose tissue, this study showed that systemic treatment with angiogenesis inhibitors results in weight reduction and adipose tissue loss. Since adipose tissue has enormous growth potential, given an appropriate metabolic challenge, it displays a high degree of plasticity with respect to its vascularization. Interestingly, angiogenesis, or the formation of new blood vessels from existing vessels, often precedes adipogenesis in developing adipose tissue microvasculature.19 Therefore, we hypothesized that RANKL and OPG might be expressed in adipocytes and thus contribute to adipogenesis and intimal inflammation and medial calcification of blood vessels.
3T3L1 cells were cultured in Dulbecco's modified high-glucose Eagle's medium (DMEM 90%; 10% (v/v) fetal calf serum (FCS)) (Gibco, Gaithersburg, MD, USA) in a humidified incubator at 37℃ with a 5% CO2 atmosphere. When cells reached confluency the media was changed to DMEM containing 10% FCS, 10 µg/mL insulin, 1 µM dexamethasone, and 0.5 mM isobutylmethylxanthine. Two days later, the isobutylmethylxanthine and dexamethasone were removed and the insulin was maintained in the media for 2 additional days. After four days, the media was replaced with DMEM (without insulin supplementation) plus 10% fetal bovine serum. Thereafter, the cells were re-fed with the same media every 2 days. The 3T3L1 cells were serum-starved overnight in DMEM/0.5% (v/v) FCS and transferred to Krebs-Ringer bicarbonate Hepes buffer, pH 7.4, containing 1% (w/v) BSA and 5 mM glucose for 90 min prior to treatment.
A full-length mouse OPG cDNA was obtained by RT-PCR amplification of total RNA that had been isolated from MC3T3E1 cells. The cDNA was subcloned into a pcDNA 3.0 vector. For long-term expression of OPG, site-specific genomic integration of OPG cDNA was achieved by transfecting OPG cDNA subcloned into the attB restriction site of a pcDNA vector containing a Phi C31 integrase. The pTA-attB plasmid and pCMV-integrase were kindly donated by Professor Calos MP.20
3T3L1 cells were plated on 6-well plates at a density of 1 × 105 cells/well the day before transfection. A total of 1 µg of each plasmid DNA was mixed with lipofectamine reagent (Invitrogen) and transfected into cells according to the manufacturer's protocol. At 48 h post-transfection, the total cellular RNA was extracted from the cells.
Total RNA from adipose tissue of Sprague-Dawley rats (male, 5 - 6 wks) and 3T3L1 cells was isolated using Tri-reagent (Invitrogen, Carlsbad, CA, USA) and then treated with DNase I (Promega). The isolated RNA was quantitated by absorbance at 260 nm; the 260/280 ratio was 1.8 or higher. One µg of total RNA was reverse transcribed using 2 U of MMLV reverse transcriptase (Promega), 0.5 µg of random primer (Promega), 0.25 mM dNTPs (Promega), and 10 U RNAsin (Promega) in a total volume of 20 uL. PCR amplification reactions contained 1 uL of RT-PCR product, 0.2 mM dNTPs, and 1.5 U Taq polymerase (Promega) in a final volume of 50 uL. PCR amplification of mouse or rat OPG was performed with 30 cycles of 94℃ for 30 sec, 60℃ (mouse) or 55℃ (rat) for 30 sec, and 72℃ for 1 min. PCR amplification of mouse or rat RANKL was performed with 30 cycles of 94℃ for 30 sec, 56℃ (mouse) or 53℃ (rat) for 30 sec, and 72℃ for 1 min. Primers sequences were as follows: OPG (rat): forward 5'-GTTCTTGCACAGCTTCACCA-3' and reverse 5'-AAACAGCCCAGTGACCATTC-3'; OPG (mouse): forward 5'-ATGCCGAGAGTGTAGAGAGGAT-3' and reverse 5'-AAACAGCCCAGTGGACCATTCCT-3'; RANKL (rat): forward 5'-ACCAGCATCAAAATCCCAAG-3' and reverse 5'-TTTGAAAGCCCCAAAGTACG-3'; RANKL (mouse): forward 5'-ACCAGCATCAAAATCCCAAG-3' and reverse 5'-TTTGAAAGCCCCAAAGTACG-3'; β-actin (rat): forward 5'-AAGCAGGAGTATGACGAGTCCG-3' and reverse 5'-GCCTTCATACATCTCAAGTTGG-3'; β-actin (mouse): forward 5'-TTCAACACCCCAGCCATGT-3' and reverse 5'-TGTGGTACGACCAGAGGCATAC-3'.
To study the effects of TNF-α on the expression of OPG and RANKL in adipocytes, 3T3L1 cells were treated with TNF-α (20 ng/mL), insulin (10 µg/mL), or Rosiglitazone (10-7 - 10-5M) for 12 days after the induction of differentiation.
The concentration of OPG in the culture medium was determined using ELISA. Briefly, 96-well plates were coated with 2 µg/mL mouse monoclonal anti-human OPG (R&D Systems, QuantikineR). An OPG standard curve was generated using recombinant mouse OPG (R&D Systems) at concentrations from 31.25 to 2000 to pg/mL. Biotinylated anti-mouse OPG (R&D Systems) at 200 ng/mL was used for the secondary antibody, and OPG was detected with streptavidin-horseradish peroxidase (R&D Systems) in combination with 3,3', 5,5'-tetramethylbenzidine (Sigma Chemical Co.). The reaction was stopped after a 5 - 20 min incubation in the dark by the addition of 50 µL of 2 M H2SO4. The plate was read at 450 nm on a Dynatech plate reader with Revelation software.
Expression of OPG mRNA increased with 3T3L1 adipocyte differentiation from 0 to 12 days after treatment with a differentiation cocktail solution. In contrast, RANKL mRNA levels did not change significantly (Fig. 1). Results are expressed as the mean value of 5 different experiments.
In the rat, OPG mRNA was expressed at higher levels in white adipose tissue than in brown adipose tissue and most abundantly in epididymal adipose tissue (Fig. 2).
To study the effects of TNF-α on the expression of OPG and RANKL in adipocytes, 3T3L1 cells were treated with TNF-α (20 ng/mL) 12 days after the induction of differentiation. After 6 hrs of treatment with TNF-α, both OPG and RANKL mRNA levels increased significantly in differentiated 3T3L1 adipocytes. RANKL mRNA levels increased more (2.5-fold) than OPG mRNA levels (1.8-fold) when compared to their expression levels in untreated 3T3L1 cells. The maximum OPG/RANKL ratio occurred two hours after treatment with TNF-α and then returned to control levels (Fig. 3).
Exposure of 3T3L1 adipocytes to insulin (10 µg/mL) decreased the expression of OPG mRNA (Fig. 4). Indeed, after 4 hrs of insulin treatment, OPG mRNA levels were suppressed by up to 70 - 80%. Expression of RANKL mRNA was higher after 6 hrs of insulin treatment.
Exposure of 3T3L1 adipocytes to Rosiglitazone decreased the expression of OPG mRNA in a dose-dependent manner (Fig. 5). OPG was suppressed more strongly than RANKL.
After the OPG cDNA was transfected into 3T3L1 cells, the cellular expression of OPG was evident. In differentiated 3T3L1 cells, however, OPG that had been secreted into culture media was barely detectable by ELISA. In contrast, ample amounts of OPG were detectable in the media with 3T3L1 cells that had been transiently transfected with OPG cDNA (Fig. 6). One possible explanation for this result may be that OPG secretion in differentiated 3T3L1 cells might be present at levels insufficient for detection by ELISA.
In the present study, we found that both OPG and RANKL mRNAs were expressed in adipocytes and that both of these proteins were differentially expressed in various adipose deposits. Furthermore, both OPG and RANKL were regulated by TNF-α, insulin, and Rosiglitazone.
The phenotype of osteoporosis and vascular calcification in OPG knockout mice has revitalized the hypothesis that metabolic bone diseases and vascular diseases, such as arterial calcification, share common pathways.3 Price et al. reported that OPG administration prevents calcification induced by warfarin or high doses of vitamin D in rats,9 but the effects of OPG in humans are different from those in rodents. A cohort study of postmenopausal women, aged at least 65 years, revealed that OPG serum levels are 30% higher in women with diabetes mellitus than those without, and are positively correlated with overall cardiovascular mortality.10 Similarly, a study on Japanese subjects found that serum OPG levels correlated positively with the severity of coronary arterial disease.12 However, based on the phenotype of OPG-deficient mice, elevated serum OPG levels in humans should be an insufficient counter-regulatory mechanism to prevent further bone loss and vascular lesions.7 RANKL and OPG are produced by extraosseous tissues and various skeletal tissues, therefore, serum levels for OPG or RANKL might not reflect their actual expression in specific tissues. Thus, the regulation and specific roles of OPG and RANKL in extraosseous tissues, including vessels, have not yet been fully determined.
Disturbance of the OPG/RANKL ratio is considered a pivotal cause of a number of osteolytic disorders, including glucocorticoid-induced osteoporosis, rheumatoid arthritis, hyperparathyroidism, myeloma bone disease, and osteolytic bone metastases.21 Here, we demonstrated the expression of both OPG and RANKL in adipocytes (Fig. 1). Specifically, expression of OPG mRNA increased with the differentiation of 3T3L1 preadipocytes, while RANKL mRNA expression was not significantly altered. Adipose tissue is not simply an energy storage depot; adipose tissue also functions as an active endocrine organ through its secretion of various cytokines.22 In our study, we observed the differential expression of OPG and RANKL in various adipose deposits in a Sprague-Dawley rat (Fig. 2). The pattern of OPG/RANKL mRNA expression in subcutaneous adipose tissue was similar in mice, rats, and humans (data not shown). OPG mRNA was expressed at higher levels in white adipose tissue than in brown adipose tissue and was most abundant in epididymal adipose tissue. Most fat in the human body is white adipose tissue. Recently, secretion of inflammatory cytokines and angiogenic factors by white adipose tissue has been stressed. Therefore, increases in the OPG/RANKL ratio in white adipose tissue have an important significance and are more indispensable for obese patients.
OPG was exclusively secreted 12 hours after transient transfection with OPG cDNA in undifferentiated and differentiated 3T3L1 cells (Fig. 6). Because adipose tissue comprises considerable portions of the body and the amount of plasma OPG varies in different metabolic conditions, our results may provide a reasonable explanation for the previously generated clinical data.10-13
Many cytokines and systemic factors are known to regulate the expression of OPG and RANKL in osteoblasts.23 TNF-α and interleukin-1β stimulate OPG mRNA expression and secretion by osteoblast-like MG-63 cells.24 We also observed that TNF-α significantly increased both OPG and RANKL mRNA levels in differentiated 3T3L1 adipocytes (Fig. 3). In obese subjects, adipocytes secrete low levels of TNF-α, which can stimulate preadipocytes to produce the monocyte chemoattractant protein-1, thereby recruiting macrophages.25 Recently, Min et al. reported that RANKL is an angiogenic factor in bone and cartilage.8 Therefore, elevation of RANKL by TNF-α could stimulate angiogenesis in adipose tissue. This effect of TNF-α might be aggravated by the potentially vicious cycle of macrophage recruitment to adipose tissue, which produces inflammatory cytokines (including TNF-α). These cytokines result in further angiogenesis and adipogenesis in morbid obesity. However, further study of these interactions is necessary because TNF-α also increased the expression of the OPG decoy receptor.
Insulin and Rosiglitazone suppressed OPG in differentiated 3T3L1 adipocytes (Fig. 4 and 5). Similar results have been observed in human vascular smooth muscle cells.26 RANKL expression was relatively preserved after treatment with insulin or Rosiglitazone, suggesting that some of the angiogenic effects induced by insulin or Rosiglitazone may be mediated through a decrease in the OPG/RANKL ratio in adipose tissue.
Like most adipokines, however, secretion of OPG into the media from both undifferentiated and fully differentiated 3T3L1 cells was barely detectable by ELISA. In order to exclude the possibility that the translation of OPG in adipocytes was impaired, we transfected 3T3L1 cells with OPG cDNA before and after differentiation. Indeed, we were able to detect an elevation of OPG in media, suggesting that the amount of OPG secreted from differentiated 3T3L1 cells may be below the detection limits of ELISA.
Our study was limited by the fact that the OPG protein was only detected in OPG-transfected cells. Accordingly, we were unable to draw conclusions about the physiologic role of OPG. Further study of the functional significance of OPG expression in adipocytes is needed.
In conclusion, we demonstrated that OPG expression was elevated during adipocyte differentiation and after TNF-α treatment in differentiated adipocytes. Considering the role of OPG in obesity-associated inflammatory changes in adipose tissue and vessels, we speculate that OPG plays a protective role against inflammation and angiogenic effects on adipose tissue. Although a causal relationship between OPG and TNF-α in vivo has not been established, we cautiously propose that OPG might be one of the adipocytokines that can reflect systemic inflammatory status. Further study is needed to clarify this issue.
Figures and Tables
Fig. 1
Expression of OPG/RANKL during adipocyte differentiation. Expression of OPG mRNA increased with the level of 3T3L1 preadipocyte differentiation, while mRNA levels of RANKL did not significantly change. OPG, osteoprotegerin; RANKL, receptor activator of NF-κB ligand. *p < 0.05.
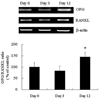
Fig. 2
Differential expression of OPG/RANKL in various adipose depots in Sprague-Dawley rats. The pattern of mRNA expression of OPG/RANKL in subcutaneous adipose tissue was similar in mice, rats, and humans. Levels of OPG mRNA were higher in white adipose tissue than in brown adipose tissue, with the most abundant levels occurring in epididymal adipose tissue. WAT, white adipose tissue; BAT, brown adipose tissue; eWAT, epididymal white adipose tissue; pWAT, periomental white adipose tissue; sqWAT, subcutaneous white adipose tissue.
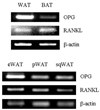
Fig. 3
Regulation of OPG and RANKL expression in the presence of TNF-α. TNF-α enhanced the expression of both OPG and RANKL mRNA in 3T3L1 preadipocytes. The OPG/RANKL ratio after TNF-α treatment was at a maximum two hours after treatment with TNF-α and then returned to control levels. *p < 0.05.
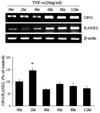
Fig. 4
Regulation of OPG/RANKL expression in the presence of insulin. Insulin (10 µg/mL) decreased the expression of OPG mRNA in 3T3L1 preadipocytes. *p < 0.01.
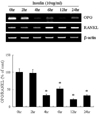
Fig. 5
Regulation of OPG/RANKL expression in the presence of Rosiglitazone. Rosiglitazone decreased the expression of OPG mRNA in 3T3L1 preadipocytes in a dose-dependent manner. *p < 0.05, **p < 0.01.
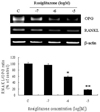
Fig. 6
OPG secretion after transfection of OPG cDNA into 3T3L1 preadipocytes. (A) Differentiation of 3T3L1 preadipocytes: a) 3T3L1 cells were first transfected with pcDNA 3.0-attB-OPG-IRES-puromycin DNA and pCMV integrase DNA for genomic integration. Then, the adipocytes were differentiated with puromycin selection for 14 days. b) 3T3L1 cells were first differentiated into adipocytes for 12 days and then transfected with pcDNA 3.0-attB-OPG-IRES-puromycin DNA only for acute transfection. The culture media was then collected and assayed 3 days after transfection. (B) Levels of OPG secretion as measured with an ELISA kit. Mock represents results from media obtained from 3T3L1 cells transfected with vector DNA. BD, before differentiation; AD, after differentiation; TBD, transfection before differentiation, which was from media obtained from 3T3L1 cells transfected with both pcDNA 3.0-attB-OPG-IRES-puromycin DNA and pCMV integrase DNA prior to differentiation into adipocytes; and TAD, transfection after differentiation, which was from media obtained from 3T3L1 cells differentiated into adipocytes first for 12 days and transfected with pcDNA 3.0-attB-OPG-IRES-puromycin DNA. Values are mean ± SE from 5 observations.
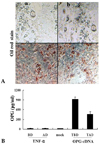
References
1. Lacey DL, Timms E, Tan HL, Kelley MJ, Dunstan CR, Burgess T, et al. Osteoprotegerin ligand is a cytokine that regulates osteoclast differentiation and activation. Cell. 1998. 93:165–176.


2. Simonet WS, Lacey DL, Dunstan CR, Kelley M, Chang MS, Lüthy R, et al. Osteoprotegerin: a novel secreted protein involved in the regulation of bone density. Cell. 1997. 89:309–319.


3. Bucay N, Sarosi I, Dunstan CR, Morony S, Tarpley J, Capparelli C, et al. Osteoprotegerin-deficient mice develop early onset osteoporosis and arterial calcification. Genes Dev. 1998. 12:1260–1268.


4. Emery JG, McDonnell P, Burke MB, Deen KC, Lyn S, Silverman C, et al. Osteoprotegerin is a receptor for the cytotoxic ligand TRAIL. J Biol Chem. 1998. 273:14363–14367.


5. Malyankar UM, Scatena M, Suchland KL, Yun TJ, Clark EA, Giachelli CM. Osteoprotegerin is an alpha vbeta 3-induced, NF-kappa B-dependent survival factor for endothelial cells. J Biol Chem. 2000. 275:20959–20962.


6. Collin-Osdoby P, Rothe L, Anderson F, Nelson M, Maloney W, Osdoby P. Receptor activator of NF-kappa B and osteoprotegerin expression by human microvascular endothelial cells, regulation by inflammatory cytokines, and role in human osteoclastogenesis. J Biol Chem. 2001. 276:20659–20672.


7. Sattler AM, Schoppet M, Schaefer JR, Hofbauer LC. Novel aspects on RANK ligand and osteoprotegerin in osteoporosis and vascular disease. Calcif Tissue Int. 2003. 74:103–106.


8. Min JK, Kim YM, Kim YM, Kim EC, Gho YS, Kang IJ, et al. Vascular endothelial growth factor up-regulates expression of receptor activator of NF-kappa B (RANK) in endothelial cells. Concomitant increase of angiogenic responses to RANK ligand. J Biol Chem. 2003. 278:39548–39557.


9. Price PA, June HH, Buckley JR, Williamson MK. Osteoprotegerin inhibits artery calcification induced by warfarin and by vitamin D. Arterioscler Thromb Vasc Biol. 2001. 21:1610–1616.


10. Browner WS, Lui LY, Cummings SR. Associations of serum osteoprotegerin levels with diabetes, stroke, bone density, fractures, and mortality in elderly women. J Clin Endocrinol Metab. 2001. 86:631–637.


11. Schoppet M, Sattler AM, Schaefer JR, Herzum M, Maisch B, Hofbauer LC. Increased osteoprotegerin serum levels in men with coronary artery disease. J Clin Endocrinol Metab. 2003. 88:1024–1028.


12. Jono S, Ikari Y, Shioi A, Mori K, Miki T, Hara K, et al. Serum osteoprotegerin levels are associated with the presence and severity of coronary artery disease. Circulation. 2002. 106:1192–1194.


13. Schoppet M, Schaefer JR, Hofbauer LC. Low serum levels of soluble RANK ligand are associated with the presence of coronary artery disease in men. Circulation. 2003. 107:e76.


14. Rasmussen LM, Ledet T. Osteoprotegerin and diabetic macroangiopathy. Horm Metab Res. 2005. 37:Suppl 1. 90–94.


16. Nakashima T, Kobayashi Y, Yamasaki S, Kawakami A, Eguchi K, Sasaki H, et al. Protein expression and functional difference of membrane-bound and soluble receptor activator of NF-kappa B ligand: modulation of the expression by osteotropic factors and cytokines. Biochem Biophys Res Commun. 2000. 275:768–775.


17. Collin-Osdoby P, Rothe L, Anderson F, Nelson M, Maloney W, Osdoby P. Receptor activator of NF-kappa B and osteoprotegerin expression by human microvascular endothelial cells, regulation by inflammatory cytokines, and role in human osteoclastogenesis. J Biol Chem. 2001. 276:20659–20672.


18. Rupnick MA, Panigrahy D, Zhang CY, Dallabrida SM, Lowell BB, Langer R, et al. Adipose tissue mass can be regulated through the vasculature. Proc Natl Acad Sci U S A. 2002. 99:10730–10735.


19. Hausman GJ, Richardson RL. Adipose tissue angiogenesis. J Anim Sci. 2004. 82:925–934.
20. Groth AC, Olivares EC, Thyagarajan B, Calos MP. A phage integrase directs efficient site-specific integration in human cells. Proc Natl Acad Sci U S A. 2000. 97:5995–6000.


21. Hofbauer LC, Schoppet M. Clinical implications of the osteoprotegerin/RANKL/RANK system for bone and vascular diseases. JAMA. 2004. 292:490–495.


22. Trayhurn P, Wood IS. Adipokines: inflammation and the pleiotropic role of white adipose tissue. Br J Nutr. 2004. 92:347–355.


23. Collin-Osdoby P. Regulation of vascular calcification by osteoclast regulatory factors RANKL and osteoprotegerin. Circ Res. 2004. 95:1046–1057.


24. Pantouli E, Boehm MM, Koka S. Inflammatory cytokines activate p38 MAPK to induce osteoprotegerin synthesis by MG-63 cells. Biochem Biophys Res Commun. 2005. 329:224–229.

