Abstract
Purpose
Local activation of the complement system plays a role in target organ damage. The aim of our study was to investigate the influence of cyclosporine (CsA)- induced renal injury on the complement system in the kidney.
Materials and Methods
Mice fed a low salt (0.01%) diet were treated with vehicle (VH, olive oil, 1mL/kg/day) or CsA (30mg/kg/day) for one or four weeks. Induction of chronic CsA nephrotoxicity was evaluated with renal function and histomorphology. Activation of the complement system was assessed through analysis of the expression of C3, C4d, and membrane attack complex (MAC), and the regulatory proteins, CD46 and CD55. CsA treatment induced renal dysfunction and typical morphology (tubulointerstitial inflammation and fibrosis) at four weeks.
The complement system is an important component of the immune system, but irregular or excessive activation of the complement system can damage tissues.1-3 For example, local activation of the terminal components of the complement system, C5a and C5b-9, is a key mediator of myocardial ischemia-reperfusion injury.4 In the kidney, excessive activation of the complement system is associated with ischemia-reperfusion injury,5 anti-glomerular basement membrane glomerulonephritis,6 immune complex nephritis,7 chronic tubulointerstitial damage,8 remnant kidney,9 nephrotic syndrome,10 and diabetic nephropathy.11 Rejection of renal transplants is caused by local synthesis of the components of the complement system.12 These findings suggest that activation of the complement system plays a role in the pathogenesis of immune and non-immune mediated renal injury.
Chronic cyclosporine A (CsA) nephrotoxicity is one of the chronic tubulointerstitial diseases in clinical practice. Long-term administration of CsA causes progressive renal failure with striped interstitial fibrosis, tubular atrophy, inflammatory cell infiltration, and hyalinosis of the afferent arterioles.13 The pathomechanism underlying the development of chronic CsA-induced nephrotoxicity is multifactorial, and several mediators including the intrarenal renin-angiotensin system, endothelin-1, nitric oxide (NO) and NO synthases, and apoptotic cell death have been implicated.13,14 However, the role of the activated complement system in the pathogenesis of chronic CsA nephrotoxicity is not well understood.
Using an experimental mouse model for chronic CsA nephrotoxicity, we evaluated the effect of chronic CsA treatment on complement components and regulatory proteins. The results of our study clearly demonstrate that the intrarenal complement system is activated in the mouse model of chronic CsA nephrotoxicity, and this may contribute to the progression of tubulointerstitial injury.
The experimental protocol was approved by the Animal Care Committee of the Catholic University of Korea. Male ICR mice (Taconic Anmed, Rockville, MD, USA) initially weighing 25g, were housed in cages in a temperature- and light- controlled environment, and allowed free access to a low salt diet (0.01% sodium, Teklad Premier, Madison, WI, USA) and tap water. Mice were randomized into two groups and treated daily for 1 (n = 12) or 4 weeks (n = 12) as follows: 1) Vehicle group (VH, n = 6): subcutaneous injection with olive oil (Sigma Diagnostics, St. Louis, MO, USA, 1mL/kg); 2) CsA group (n = 6): subcutaneous injection with CsA (Novartis Pharma, Basel, Switzerland, 30mg/kg). This dosage and method of CsA administration were chosen according to a previous report.15,16
After the treatment was started, mice were pair-fed and daily body weight (BW) was monitored. Whole blood CsA levels were measured by a monoclonal radioimmunoassay (Incstar Co., Stillwater, MN, USA). Prior to sacrifice, animals were individually housed in metabolic cages (Tecniplast Gazzada S.A.R.L., Italy) for 24-h urine collection, and blood samples were obtained to evaluate serum creatinine (Scr) and blood urea nitrogen (BUN) levels. The creatinine clearance rate (ClCr) was calculated by the standard formula.
Mice were perfused through the left ventricle of the heart, and the kidney tissues were fixed with a periodate-lysine-paraformaldehyde (PLP) solution and then embedded in wax. After dewaxing, 4-µm sections were processed and stained with Masson's trichrome and hematoxylin.
After dewaxing, sections were incubated with a 0.5% Triton X100-PBS solution for 30 min and washed with phosphate buffered saline (PBS) three times. Non-specific binding sites were blocked for 1h with normal donkey serum diluted 1:10 in PBS, and then incubated overnight at 4℃ with antibodies diluted in 1:1000 in a humid environment. After rinsing in Tris-buffered saline (TBS), sections were incubated in peroxidase-conjugated donkey anti-mouse or rabbit IgG Fab fragment (Jackson ImmunoResearch Laboratories, West Grove, PA, USA) for 30 min. For staining, sections were incubated with a mixture of 0.05% 3,3'-diaminobenzidine containing 0.033% H2O2 at room temperature until a brown color was visible, washed with TBS, counterstained with hematoxylin and examined under light microscopy. The primary antibodies were as follows: C3 (Santa Cruz Biotechnology, Santa Cruz, CA, USA); C9, rabbit anti-rat polyclonal antibody (cross-reactive with mouse C9, kindly provided by Dr. B. P. Morgan, Cardiff University);17 C4d, a murine monoclonal antibody (Quidel Corporation, San Diego, CA, USA); CD55, a rabbit anti-rat polyclonal antibody (H-319, Santa Cruz Biotechnology, Santa Cruz, CA, USA).
For immunoblotting analysis, whole kidney tissues were homogenized in lysis buffer. Homogenates were centrifuged at 3000 rpm for 15 min at 4℃, and the protein concentration of the lysate was determined using the Bradford method (Bio-RAD, Hercules, CA, USA). Protein samples were resolved on a 15% SDS-polyacrylamide gel (SDS-PAGE) and then electroblotted onto Bio-Blot nitrocellulose membrane (Bio-RAD, Hercules, CA, USA). Ponceau S staining was performed to verify that equal amounts of protein (50µg) were loaded. The membrane was blocked for 1h in Tris-buffered saline with tween-20 (TBS-T, 10mM Tri-CL, 150mM NaCl [pH8.0], 0.05% tween-20) containing 5% nonfat powdered milk. C9 and CD46 were detected by incubating membranes for 1h with primary antibodies diluted 1:1000 (rabbit polyclonal anti-CD46 antibody, H-294, Santa Cruz Biotechnology, Santa Cruz, CA, USA). Primary antibody incubation was followed by six washes with TBS-T. The blot was then incubated with peroxidase-linked anti-mouse or rabbit IgG as a secondary antibody (Amersham Biosciences, Buckinghamshire, England) for 1h at room temperature. Antibody-reactive protein was detected using enhanced chemiluminescence (ECL, Amersham Life Science, UK). Optical densities were obtained using the VH group as a reference for 100%.
For double labeling with C4d or C3 and α-smooth muscle actin (α-SMA, Sigma Diagnostics), the tissue sections were prepared as described above. In brief, before incubation with primary antibodies, the tissue sections were permeabilized by incubation for 15 min in 0.5% Triton X-100 in PBS, and then blocked with normal donkey serum diluted 1:10 in PBS for 1h. Subsequently, the tissue sections were incubated overnight at 4℃ with monoclonal C4d or C3 antibody. The tissue sections were rinsed in PBS and incubated with Cy3-conjugated donkey anti-mouse antibody (diluted 1:500; Jackson ImmunoResearch Laboratories) for 2 h at room temperature. Tissues were rinsed with PBS, and then incubated with α-SMA antibody (diluted 1:300; Molecular probes, Inc. Eugene, OR, USA) for 2h at room temperature. The microscopic analysis was carried out using an MRC-1024 laser confocal microscope (Bio-Rad, Hercules, CA, USA) after the sections were mounted in Kaiser's glycerol gelatin (Merck, Dermstadt, Germany).
Mean body weights of both treatment groups increased during the experiment. Mean body weight (28 ± 0.8 vs. 35 ± 0.8, p < 0.05) and renal function (Scr: 0.70 ± 0.4 vs. 0.54 ± 0.04; BUN: 106 ± 24 vs. 42 ± 5; ClCr: 0.13 ± 0.03 vs. 0.24 ± 0.03, p < 0.05, respectively) was significantly lower in the CsA group than in the vehicle group after week 4 (Table 1). The mean concentration of CsA was 1305 ± 28ng/mL after week 1, and 1683 ± 83ng/mL after week 4. After week 4, the morphological features of the kidneys from CsA-treated mice (striped tubulointerstitial fibrosis, tubular atrophy, and inflammatory cell infiltration) were similar to those of kidneys from human cases of chronic CsA-induced nephrotoxicity (Fig. 1).
Immunoreactivity of C4d and C3 in the kidneys of mice treated with vehicle was slight. Expression of C4d and C3 in the renal tubular epithelium of the kidneys after week 1 was significantly increased by CsA treatment. A further increase was observed after week 4, when interstitial fibrosis and tubular atrophy appeared (Fig. 2 and Fig. 3). Glomeruli were negative for C3 and C4d. To clarify the localization of C4d-positive cells, double immunolabeling was performed using α-smooth muscle actin as a capillary marker. There were no merged cells within the tubulointerstitium (Fig. 4), which suggests that the C4d-positive cells are renal tubular cells.
We evaluated the expression of the terminal membrane attack complex (MAC) using an antibody against C9. CsA treatment enhanced MAC immunoreactivity in the interstitium and renal proximal tubules; this effect was time-dependent and restricted to subapical regions with an injured brush border (Fig. 5). Consistent with the immunohistochemical results, immunoblotting for MAC revealed that the expression of MAC protein was significantly increased by CsA in a time-dependent manner (1wk: 201 ± 36 vs. 100 ± 2; 4wk: 891 ± 37 vs. 100 ± 2, p < 0.05 vs. VH).
Expression of complement regulatory proteins (CD55 and CD46) was studied using immunohistochemistry or immunoblotting. Immunohistochemical analysis revealed that CD55 was expressed in the endothelial cells of the glomeruli and the vascular bundles of the outer medullas in the VH group and immunoreactivity of CD55 was increased by CsA treatment (Fig. 6). CD46 protein was also significantly increased by 4 weeks of CsA treatment (Fig. 7, 180 ± 11 (CsA) vs. 100 ± 20 (VH), p < 0.05).
The results of this study clearly demonstrate that CsA-induced renal injury increased the expression of C3, C4d, and the MAC (C9), and this was accompanied by upregulation of complement system regulatory proteins (CD46 and CD55). Immunohistochemistry revealed that the activated components of the complement system were mainly in the injured tubules and interstitium. These findings suggest that CsA-induced renal injury is associated with activation of the intrarenal complement system.
To define whether CsA-induced renal injury activates the complement system in the kidney, we evaluated C3 expression since the alternative, classical and mannose-binding lectin pathways activate variants of C3, causing a cascade of cleavage and generation of the proinflammatory and regulatory molecules.1,2 We found that C3 immunoreactivity was significantly increased by CsA treatment and this increase was localized to the tubular cells in the injured area. This finding is consistent with previous reports that the C3 gene is expressed in renal tubular epithelial cells and that expression of C3, which does not occur in histologically normal specimens, is associated with diffuse inflammatory process and focal renal scarring.18,19
In general, deposition of C4d along peritubular capillaries is regarded as an index of an antibody-mediated rejection (AMR) in chronic allograft nephropathy.20,21 However, C4d expression overlaps with other clinical conditions such as ischemia-reperfusion injury22 and other toxic injuries.23 In this study, immunohistochemical analysis revealed that CsA treatment increased C4d immunoreactivity in the interstitial fibrosis area, and doubling labeling with α-smooth muscle actin showed that the C4d-positive cells were renal tubular cells. Our finding suggests that C4d deposition in the renal tubular cells in response to CsA-induced renal injury is an important point for differentiating from antibody-mediated rejection.
The MAC is a terminal product of complement activation, and mediates chronic tubulointerstitial damage.24,25 Immunohistochemistry and immunoblotting showed that the expression of C9 in CsA-treated kidneys was twice that in the controls at the end of week 1 and nine times that in the controls after week 4. Immunolocalization revealed that C9 was present on the luminal side of the tubular cells. Our observation is consistent with a previous study showing that renal epithelial cells are vulnerable to attack by C5b-9 and that MAC formation is closely associated with morphologic alterations of the kidney (interstitial fibrosis and inflammatory cells influx).25
Host tissues express a number of membrane-bound complement inhibitors to overwhelm complement-mediated autologous attacks. The protective roles of complement regulatory proteins have been established in studies of CD55- and CD59-deficient mice7 and by administration of an anti-CD59 antibody.12 Decay accelerating factor (DAF, CD55), membrane cofactor protein (MCP, CD46), and CD59 are ubiquitously distributed in the kidney, and their expression may be affected by complement attack and cytokines. In our study, CsA treatment significantly increased CD55 and CD46 expression in parallel with complement deposition, which is consistent with previous results in studies on membranoproliferative glomerulonephritis, IgA nephropathy, and lupus nephritis.26-28 This finding suggests that CsA-induced renal injury increases complement regulatory proteins as well as complement components, and an increase in regulatory proteins may be explained as a compensatory response against an activated complement system.
Although the mechanism by which chronic CsA treatment stimulates the complement system in chronic CsA nephrotoxicity is unknown, two mechanisms are possible. Firstly, chronic CsA nephrotoxicity is associated with enhancement of proinflammatory mediators (C-reactive protein and tumor necrosis factor-α),29,30 which induce production of the central complement component C3.31,32 Secondly, activated toll-like receptors may indirectly induce expression of the complement system. Previously, we demonstrated that long-term CsA treatment upregulates TLR4 mRNA and protein expression29 and that this may be related to an increased synthesis of complement. Mice deficient in toll-like receptor 4 had lower serum C3 deposition through the alternative pathway and lower de novo synthesis of C3 than wild-type mice,33 suggesting that TLR-4 signaling affects the turnover rate of the complement cascades. Taken together, the results indicate that activated inflammatory mediators and toll-like receptors during CsA-induced renal injury may trigger activation of the intrarenal complement system.
Two issues should be considered in the interpretation of our study. First, the choice of drug dosage is important in evaluating any effects. In this study, CsA was administered at a dose of 30mg/kg/d, which is higher than that given to humans (usually 4-5mg/kg/d in clinical practice). Unlike the human kidney, the mouse kidney is resistant to CsA-induced renal injury. Indeed, our group15 and other groups16,34,35 have shown that a high dose of CsA is required to reproduce a mouse model of chronic CsA nephrotoxicity. In addition, the role of intrarenal complement system activation in CsA-induced renal injury is unknown. However, there are some studies linking complement activation and renal tubular interstitial injury.9,34 The results of this study revealed that the intrarenal complement system is activated in the injured tubulointerstitium of a mouse model for chronic CsA nephrotoxicity. Therefore, we propose that the activated complement components may be related, in part, to renal tubulointerstitial injury in this animal model of chronic CsA nephrotoxicity. Future clinical studies are required to confirm this finding.
In conclusion, overexpression of components of the complement system may be one of the mechanisms responsible for the development of chronic CsA nephrotoxicity. This finding provides further information on the mechanism of CsA-induced renal injury and identifies potential therapeutic targets.
Figures and Tables
Fig. 1
Representative photomicrographs of the histomorphology for each group. CsA treatment for four weeks induced interstitial fibrosis. Trichrome stain, original magnification × 200.
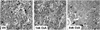
Fig. 2
Representative photomicrographs of immunohistochemistry for C3. CsA treatment resulted in a significant increase in C3 immunoreactivity. Immunohistochemical staining, original magnification, × 200; insets × 1000.
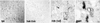
Fig. 3
Representative photomicrographs of immunohistochemistry for C4d. CsA treatment resulted in a significant increase in C4d immunoreactivity (arrow heads). Immunohistochemical staining, original magnification, × 200; insets (a) × 1000.
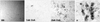
Fig. 4
Double immunofluorescence with vessel markers for α-smooth muscle actin (green) and C4d or C3 (red). Original magnification × 400.
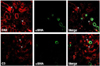
Fig. 5
Immunohistochemistry (A) and immunoblotting (B) for membrane attack complex (MAC). Note that the increase in MAC protein expression in renal tubules induced by CsA treatment was confined to the apical side (double arrows) and the tubulointerstitium (asterisks). Magnifications: inset × 1000; others × 200. #p < 0.05 vs. VH.
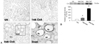
Fig. 6
Immunohistochemistry of CD55. CD55 is strongly expressed in the endothelial cells of the glomeruli and the vascular bundles of the outer medulla (arrows). Dotted lines indicate the vascular bundle of the outer medulla. VB, vascular bundle. Original magnification × 200.
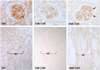
References
1. Walport MJ. Complement. First of two parts. N Engl J Med. 2001. 344:1058–1066.
2. Walport MJ. Complement. Second of two parts. N Engl J Med. 2001. 344:1140–1144.
3. Nangaku M, Shankland SJ, Couser WG. Cellular response to injury in membranous nephropathy. J Am Soc Nephrol. 2005. 16:1195–1204.


4. Vakeva AP, Agah A, Rollins SA, Matis LA, Li L, Stahl GL. Myocardial infarction and apoptosis after myocardial ischemia and reperfusion: role of the terminal complement components and inhibition by anti-C5 therapy. Circulation. 1998. 97:2259–2267.


5. Yamada K, Miwa T, Liu J, Nangaku M, Song WC. Critical protection from renal ischemia reperfusion injury by CD55 and CD59. J Immunol. 2004. 172:3869–3875.


6. Sogabe H, Nangaku M, Ishibashi Y, Wada T, Fujita T, Sun X, et al. Increased susceptibility of decay-accelerating factor deficient mice to anti-glomerular basement membrane glomerulonephritis. J Immunol. 2001. 167:2791–2797.


7. Sacks SH, Zhou W, Andrews PA, Hartley B. Endogenous complement C3 synthesis in immune complex nephritis. Lancet. 1993. 342:1273–1274.


8. Hsu SI, Couser WG. Chronic progression of tubulointerstitial damage in proteinuric renal disease is mediated by complement activation: a therapeutic role for complement inhibitors? J Am Soc Nephrol. 2003. 14(7 Suppl 2):S186–S191.


9. Nangaku M, Pippin J, Couser WG. C6 mediates chronic progression of tubulointerstitial damage in rats with remnant kidneys. J Am Soc Nephrol. 2002. 13:928–936.


10. Nangaku M, Alpers CE, Pippin J, Shankland SJ, Kurokawa K, Adler S, et al. CD59 protects glomerular endothelial cells from immune-mediated thrombotic microangiopathy in rats. J Am Soc Nephrol. 1998. 9:590–597.


11. Qin X, Goldfine A, Krumrei N, Grubissich L, Acosta J, Chorev M, et al. Glycation inactivation of the complement regulatory protein CD59: a possible role in the pathogenesis of the vascular complications of human diabetes. Diabetes. 2004. 53:2653–2661.
12. Pratt JR, Basheer SA, Sacks SH. Local synthesis of complement component C3 regulates acute renal transplant rejection. Nat Med. 2002. 8:582–587.


13. Li C, Lim SW, Sun BK, Yang CW. Chronic cyclosporine nephrotoxicity: new insights and preventive strategies. Yonsei Med J. 2004. 45:1004–1016.


14. Olyaei AJ, de Mattos AM, Bennett WM. Nephrotoxicity of immunosuppressive drugs: new insight and preventive strategies. Curr Opin Crit Care. 2001. 7:384–389.


15. Yang CW, Faulkner GR, Wahba IM, Christianson TA, Bagby GC, Jin DC, et al. Expression of apoptosis-related genes in chronic cyclosporine nephrotoxicity in mice. Am J Transplant. 2002. 2:391–399.


16. Mazzali M, Hughes J, Dantas M, Liaw L, Steitz S, Alpers CE, et al. Effects of cyclosporine in osteopontin null mice. Kidney Int. 2002. 62:78–85.
17. Turnberg D, Botto M, Warren J, Morgan BP, Walport MJ, Cook HT. CD59a deficiency exacerbates accelerated nephrotoxic nephritis in mice. J Am Soc Nephrol. 2003. 14:2271–2279.


18. Tang S, Sheerin NS, Zhou W, Brown Z, Sacks SH. Apical proteins stimulate complement synthesis by cultured human proximal tubular epithelial cells. J Am Soc Nephrol. 1999. 10:69–76.


19. Welch TR, Beischel LS, Witte DP. Differential expression of complement C3 and C4 in the human kidney. J Clin Invest. 1993. 92:1451–1458.


20. Collins AB, Schneeberger EE, Pascual MA, Saidman SL, Williams WW, Tolkoff-Rubin N, et al. Complement activation in acute humoral renal allograft rejection: diagnostic significance of C4d deposits in peritubular capillaries. J Am Soc Nephrol. 1999. 10:2208–2214.
21. Mroz A, Lewandowski Z, Cieciura T, Matlosz B, Pazik J, Kwiatkowski A, et al. C4d complement split product in diagnosis of immunological activity of chronic allograft nephropathy. Transplant Proc. 2006. 38:97–100.


22. Straatsburg IH, Boermeester MA, Wolbink GJ, van Gulik TM, Gouma DJ, Frederiks WM, et al. Complement activation induced by ischemia-reperfusion in humans: a study in patients undergoing partial hepatectomy. J Hepatol. 2000. 32:783–791.


23. Chang T, Chowdhry S, Budhu P, Kew RR. Smokeless tobacco extracts activate complement in vitro: a potential pathogenic mechanism for initiating inflammation of the oral mucosa. Clin Immunol Immunopathol. 1998. 87:223–229.


24. Nangaku M, Pippin J, Couser WG. Complement membrane attack complex (C5b-9) mediates interstitial disease in experimental nephrotic syndrome. J Am Soc Nephrol. 1999. 10:2323–2331.


25. Rangan GK, Pippin JW, Coombes JD, Couser WG. C5b-9 does not mediate chronic tubulointerstitial disease in the absence of proteinuria. Kidney Int. 2005. 67:492–503.


26. Endoh M, Yamashina M, Ohi H, Funahashi K, Ikuno T, Yasugi T, et al. Immunohistochemical demonstration of membrane cofactor protein (MCP) of complement in normal and diseased kidney tissues. Clin Exp Immunol. 1993. 94:182–188.


27. Cosio FG, Sedmak DD, Mahan JD, Nahman NS Jr. Localization of decay accelerating factor in normal and diseased kidneys. Kidney Int. 1989. 36:100–107.


28. Tamai H, Matsuo S, Fukatsu A, Nishikawa K, Sakamoto N, Yoshioka K, et al. Localization of 20-kD homologous restriction factor (HRF20) in diseased human glomeruli. An immunofluorescence study. Clin Exp Immunol. 1991. 84:256–262.


29. Lim SW, Li C, Ahn KO, Kim J, Moon IS, Ahn C, et al. Cyclosporine-induced renal injury induces toll-like receptor and maturation of dendritic cells. Transplantation. 2005. 80:691–699.


30. Li C, Yang CW, Park JH, Lim SW, Sun BK, Jung JY, et al. Pravastatin treatment attenuates interstitial inflammation and fibrosis in a rat model of chronic cyclosporine-induced nephropathy. Am J Physiol Renal Physiol. 2004. 286:F46–F57.


31. Shagdarsuren E, Wellner M, Braesen JH, Park JK, Fiebeler A, Henke N, et al. Complement activation in angiotensin II-induced organ damage. Circ Res. 2005. 97:716–724.


32. Pasch MC, Van Den Bosch NH, Daha MR, Bos JD, Asghar SS. Synthesis of complement components C3 and factor B in human keratinocytes is differentially regulated by cytokines. J Invest Dermatol. 2000. 114:78–82.


33. Lee EK, Kang SM, Paik DJ, Kim JM, Youn J. Essential roles of Toll-like receptor-4 signaling in arthritis induced by type II collagen antibody and LPS. Int Immunol. 2005. 17:325–333.

