Abstract
Purpose
Surface antigen 3 (SAG3) of Toxoplasma gondii is very similar in structure to the major surface antigen 1 (SAG1). Although numerous studies have supported the importance of SAG1 in protection against T. gondii infection, few reports exist on SAG3.
Materials and Methods
Glutathione-S-transferase (GST)-fused SAG3 of T. gondii (rSAG3) were immunized into BALB/c mice alone or in combination with Quil A (rSAG3/Quil A), and then evaluated the protective immunity in vivo and in vitro against murine toxoplasmosis.
Results
Immunization with rSAG3 or rSAG3/Quil A resulted in significantly more survival days and fewer brain cysts after challenge with T. gondii compared to an infected control group. Mice immunized with rSAG3 alone or in combination with Quil A produced significantly more specific IgG2a antibody, whereas specific IgG1 antibody titers did not increase. The percentage of CD8+ T cells, IFN-γ mRNA expression, and nitric oxide production significantly increased in rSAG3- and rSAG3/Quil A-immunized mice.
Toxoplasma gondii is an obligate intracellular protozoan parasite with a global distribution among humans and mammals. Although this parasite most often causes subclinical infection, primary infection during pregnancy can induce fetal pathology and abortion in both humans and animals. In the chronic phase, reactivation of the infection can be life-threatening for immunocompromised individuals.1 Thus, a vaccine against T. gondii would be valuable for preventing both fetal infection and reactivation in at-risk groups.
Surface antigens of T. gondii play an important role in attachment, signaling, invasion, transport, and interaction with the host's immune system.2 The representative major surface antigens of T. gondii tachyzoites are SAG1 (surface antigen 1 or P30), SAG2 (P22), and SAG3 (P43).3-5 The use of parasite antigens for vaccination remains controversial because it is difficult to standardize the methods of purification and the quality of purified antigens. To overcome these drawbacks, many researchers have recently begun using recombinant antigens. SAG1 represents 3-5% of the total protein of the parasite.2 Immunization with recombinant SAG1 induces protective immunity against lethal infection in mice, and numerous studies have supported the role of SAG1 in protection against T. gondii infection.6-9 However, Kasper et al.10 reported that vaccination with SAG1 provided insufficient protection.
SAG3 is very similar to SAG1 in structure and function. SAG1 and SAG3 antigens are anchored to the membrane via glycosylphosphatidylinositol groups,3,11 and these two surface proteins have been shown to participate in cellular invasion and attachment.11,12 However, few studies have been performed with SAG3.13 In this report, we produced recombinant SAG3 (rSAG3) in Escherichia coli as a hybrid protein fused to glutathione-S-transferase (GST). Mice were immunized with rSAG3- GST alone or in combination with saponin adjuvant Quil A, and protective immunity against murine toxoplasmosis was evaluated in vivo and in vitro.
Inbred BALB/c mice were obtained from the Korea Research Institute of Bioscience and Biotechnology, Daejeon, Korea. All mice were female, 6-8 weeks old, and documented to be specific-pathogen-free. Animal studies were carried out in a pathogen-free barrier zone at the College of Medicine, Chungnam National University, in accordance with the procedure outlined in the Guide for the Care and Use of Laboratory Animals. Two strains of T. gondii were used. The RH strain was used to prepare Toxoplasma lysate antigen (TLA) and to evaluate parasite multiplication in vitro, and mice were orally infected with the Me49 strain to evaluate survival time and parasite burden in vivo.
Recombinant SAG3 fusion protein was produced as previously described.4,5 Briefly, template DNA was extracted with phenol/chloroform/isoamylalcohol from tachyzoites pretreated with 1mM K buffer [0.1M Tris (pH 7.5), 12.5mM EDTA, 0.15M NaCl, 1% SDS, 20mg/mL proteinase K] at 58℃ for 1h and 37℃ for 16h. To produce recombinant SAG3, the corresponding genes were amplified by PCR using the following oligonucleotide primers: sense primer, 5'-GGGCCGGATCCCAGCTGTGGCGGCGCA-3', antisense primer, 5'-GGGCCGAATTCGCAGCCACATGCAC-3'. The forward primer contained a BamHI restriction site (bold nucleotides), and the reverse primer contained an EcoRI site. PCR products were digested with BamHI and EcoRI, cloned into the pGEX-4T-1 system (Amersham Pharmacia Biotech, Uppsala, Sweden), and the resulting clones were sequenced. GST fusion proteins were expressed in E. coli BL21 (DE3). Fusion proteins were present in both supernatant and pellet, and were purified from supernatant using prepacked GST-Sepharose 4B columns in accordance with the manufacturer's instructions (Amersham Pharmacia Biotech). The purity of the recombinant proteins was confirmed using SDS-PAGE. Endotoxins were removed using AffinityPak Detoxi-Gel Endotoxin Removing Gel (Pierce Biotechnology Inc., Rockford, IL, USA). After endotoxin removal, the lipopolysaccharide content was measured by the Limulus amoebocyte lysate assay. Less than 10pg/mL of LPS was detected in the protein preparations.
As a control protein, GST protein was expressed by E. coli transformed with only the expression vector and was purified using a GST affinity column.
BALB/c mice were intraperitoneally vaccinated with 50µg of rSAG3 alone, rSAG3 in combination with 20µg of Quil A (Accurate Chemical and Scientific Co., Westbury, NY, USA) or GST alone twice weekly for 4 weeks. Mice were divided into six groups of 25 mice: 10 to evaluate survival, 5 to measure the number of parasitic cysts in the brain, and 10 to evaluate immunologic characteristics (which were examined twice using 5 mice each). Experimental groups were as follows: mice immunized with rSAG3 in combination with Quil A (rSAG3/Quil A); mice immunized with rSAG3 (rSAG3), GST (GST), or Quil A alone (Quil A); mice immunized with GST and Quil A (GST/Quil A); and untreated mice (Control).
Mice were orally infected with an avirulent Me49 strain of T. gondii 3 weeks after the final immunization. A lethal dose (1,500 cysts per mouse) was used and mortality was checked every day for 4 weeks. A nonlethal dose (20 cysts per mouse) was used to assess brain cyst numbers and immunological characteristics. Age- and sex-matched, un-immunized control mice were infected with similar doses of parasite.
Three weeks after the final immunization, sera were collected and IgG subclasses measured through titration. 96-well plates were coated with TLA (10µg/mL) and incubated overnight at 4℃. After blocking, serum samples were diluted 1:100 in PBS containing 0.1% BSA and 0.05% Tween 20, and 100µL of diluted serum was added to each well. After 2h, HRP-conjugated goat anti-mouse IgG, IgG1, or IgG2a (Southern Biotechnology Associates Inc., Birmingham, AL, USA) was applied. After washing, freshly prepared O-phenylenediamine dihydrochloride was added, and the reaction was stopped by the addition of 4N H2SO4. OD at 492nm was read using an automatic ELISA plate reader.
Three weeks after the final immunization, spleens were harvested and homogenized, and erythrocytes were lysed using Tris-NH4Cl (pH 7.2). Splenocyte preparations containing 1 × 106 cells were incubated with 50µL of FITC-conjugated anti-mouse CD4, CD8α, or γδ monoclonal antibodies or an isotype-specific control (1:100 dilution in 0.1% BSA/PBS; PharMingen, San Diego, CA, USA) for 1 h at 4℃. Cells were washed three times in 0.1% BSA/PBS by centrifugation, fixed with 1%paraformaldehyde, and analyzed by flow cytometry (Becton Dickinson, Franklin Lakes, NJ, USA). Data were analyzed using Cell-Quest (Becton Dickinson).
Splenocytes were dispensed in 96-well culture plates at a concentration of 2 × 105 cells per well in RPMI 1640 containing 10% FBS. Cells were cultured for 72h with or without TLA (25µL/mL) at 37℃, 5% CO2. After incubation, wells were pulsed with 0.5µCi [3H]-thymidine (Amersham Belgium, Gent, Belgium) for 12h and harvested onto glass-fiber filters using an automatic cell harvester. [3H]-thymidine incorporation was assessed using a liquid scintillation counter, and results were expressed as counts per minute (CPM). Data reported represent the mean ± standard error (SE) of triplicate cultures.
Three weeks after the final immunization, cytokine mRNA expression in the spleens of vaccinated mice was assessed according to the method of Deckert-Schluter et al.14 Total RNA was isolated using a RNAgent kit (Promega, Madison, WI, USA). A preparation of cDNA was produced using a starting mixture containing 5µg of total RNA, 8µL of 5 × RT buffer, 4µL of dNTPs, 25µM oligo-dT20, and 1µL of AMV reverse transcriptase. PCR was performed with 2 to 13µL of cDNA reaction mixture containing 10 × polymerase buffer, 250µM dNTPs, 0.4µM of 3'- and 5'-primers, and 2.5U Taq polymerase at 94℃ for 1 min, 57℃ for 1 min, and 72℃ for 1 min in a thermal cycler (Applied Biosystems, Foster City, CA, USA). Cytokine-specific primer pairs were as follows: HPRT, 5'-GAGGGTAGGCTGGCCTATGGCT-3' and 5'-TTGGATACAGGCCAGACTTTGTTG-3'; IFN-γ, 5'-CTCATGGAATGCATCCTTTTTCG-3' and 5'-ACGCTACACACTGCATCTTGG-3'; TNF-α, 5'-ATCTCAAAGACAACCAACTAGTG-3' and 5'-CTCCAGCTGGAAGACTCCTCCCAG-3'; and IL-10, 5'-TGTCTAGGTCCTGGAGTCCAGCAGACTCAA-3' and 5'-CCAGTTTTACCTGGTAGAAGTGATG-3'. After 35 cycles, PCR products were separated by electrophoresis on 2% agarose gels. Quantification of mRNA was performed using an imaging densitometer (Bio-Rad, Hercules, CA, USA).
Three weeks after the last immunization, peritoneal cells were harvested by repeated lavage of the peritoneal cavity with Hanks' balanced salt solution (HBSS). After washing, cell pellets were resuspended in RPMI 1640 containing 10% heat-inactivated FBS and antibiotics and were cultured in a 13-mm coverslip (Nunc, Roskilde, Denmark) for 2h at 37℃ in 5% CO2. Nonadherent cells were removed by rinsing with warm HBSS. Cultures were then incubated for 3 days to obtain macrophage monolayers, after which an aliquot of supernatant was harvested for measurement of nitrite (NO2-) levels by the Griess assay.15 In brief, 100µL of supernatant was mixed with 600µL of a 1:1 mixture of 1.5% sulfanilamide in 1N HCl and 0.15% N-(1-naphthyl)ethylenediamide in distilled water. After a 30 min incubation at room temperature, absorbance was read at 540nm and NO2- concentration was calculated using a standard curve generated with NaNO2.
Results are expressed as the mean ± standard error (SE) for each group. Statistical evaluation of the difference in survival rates was checked by Kaplan-Meier test, and then a Log-Rank test was done. Statistical differences in parasite loads, antibody titers, phenotype profiles, mRNA levels, and nitrite levels were determined using the Kruskal Wallis test. Differences among the various groups were considered significant when p < 0.05.
With a set of primers used to amplify the ORF of the SAG3 gene, a 1,158-bp DNA fragment was obtained by RT-PCR. After EcoRI and BamHI digestion, each fragment was inserted into pGEX- 4T-1 (Promega). pGEX-4T-1 and recombinant plasmids were transformed into E. coli BL21. Immunoblot analysis using GST detection confirmed the expression of GST and GST fused SAG3 and the reaction of purified rSAG3 with GST detection (Fig. 1).
Mice were vaccinated with rSAG3 alone or in combination with Quil A according to the protocol. Three weeks after the final vaccination, mice were challenged with a lethal dose of T. gondii strain Me49 cysts, and survival rates were evaluated for 4 weeks after infection. As shown in Fig. 2, 20% of rSAG3-immunized mice survived the experimental period. This was a significant improvement in survival compared to the infection control, GST-, Quil A-, or GST/Quil A-immunized groups (p < 0.05). Moreover, immunization with rSAG3 in combination with Quil A resulted in a further increase in survival compared to rSAG3-immunization alone (p > 0.05).
Three weeks after the last immunization, mice were infected with a nonlethal dose of T. gondii Me49. Mice were killed 4 weeks after infection, and cysts in the brain were counted under a microscope. The average number of cysts ± SD was 980 ± 86 per mouse in the infection control group. The number of brain cysts was not significantly different in GST-(920 ± 86), Quil A-(860 ± 68), or GST/Quil A-(800 ± 71) immunized mice. However, rSAG3- and rSAG3/Quil A-immunized mice had significantly fewer brain cysts compared to the GST- or GST/Quil A-immunized mice (438 ± 39 in rSAG3, p < 0.05; 402 ± 34 in rSAG3/Quil A, p < 0.05). No significant difference was observed between rSAG3- and rSAG3/Quil A-immunized mice (Fig. 3).
Serum samples from vaccinated mice were assayed for IgG subclass by ELISA. The specific IgG, IgG1, and IgG2a titers of GST-, Quil A-, and GST/Quil A-immunized mice were similar to that of the control group. The specific IgG, IgG1, and IgG2a titers of rSAG3- and rSAG3/Quil A-immunized mice were significantly higher than those of GST-, Quil A-, or GST/Quil A-immunized mice (p < 0.05). However, the increase in IgG2 titer was greater than the increase in IgG1 titer (Fig. 4).
As shown in Fig. 5, thymidine incorporation by TLA-treated splenocytes from rSAG3- or rSAG3/Quil A-immunized mice significantly increased in comparison to the GST-, Quil A-, and GST/Quil A-immunized mice. DNA synthesis in GST-, Quil A-, and GST/Quil A-immunized mice was similar to that of the control group.
To evaluate the change in T cell subsets after rSAG3 vaccination, we performed a phenotype analysis of murine splenocytes. As shown in Fig. 6, the percentage of CD4+ T cells from rSAG3- and rSAG3/Quil A-immunized mice did not change significantly during the experimental period compared to the control, GST-, Quil A-, and GST/Quil A-immunized groups. However, the percentage of CD8+ T cells in rSAG3- and rSAG3/Quil A-immunized mice was significantly higher (p < 0.05). When compared to GST-immunized mice, the percentage of γδ T cells of rSAG3-, or rSAG3/Quil A-immunized mice had increased significantly (p < 0.05). However, there was no significant difference in the percentages of CD8 and γδ T cells in GST/Quil A- and rSAG3/Quil A-immunized mice.
The expression of HPRT mRNA in splenocytes was almost equal in all groups during the experimental period (data not shown). To determine whether rSAG3 immunization augments a Th1 or Th2 cytokine response, splenocytes were obtained from mice 3 weeks following the last immunization and assayed for IFN-γ, TNF-α, and IL-10 mRNA expression by RT-PCR. As shown in Fig. 7, the expression of IFN-γ mRNA in rSAG3- and rSAG3/Quil A-immunized mice significantly increased compared to the GST-, Quil A-, or GST/ Quil A-immunized groups (p < 0.05). However, there was no significant difference in TNF-α and IL-10 mRNA expression.
NO production by peritoneal macrophages from control mice was 3.4 ± 0.13µM. No statistically significant difference was found among the control, GST-, and Quil A-vaccinated groups. However, NO production in rSAG3- and rSAG3/Quil A-immunized mice was significantly higher (9.7 ± 0.54 and 11.9 ± 0.40µM, respectively; p < 0.05) compared to the GST- and GST/Quil A-immunized groups (Fig. 8).
We expressed the T. gondii SAG3 in E. coli as a GST fusion protein (rSAG3) by PCR amplifying the P43 coding sequence and cloning it into the plasmid pGEX-4T-1. The rSAG3 protein reacted with T. gondii-infected mouse serum in Western blots. Specific antibody titers and splenocyte proliferation also increased remarkably in rSAG3- and rSAG3/Quil A-immunized mice. These results indicate that our rSAG3 shares immunologically active epitopes with native T. gondii. In the present study, rSAG3-immunized mice showed significantly increased survival rates, serum IgG2a, IFN-γ mRNA expression, and percentage of CD8+ T cells as well as decreased cyst burdens in the brain. When Quil A was used as an adjuvant, the survival rate of rSAG3/Quil A-immunized mice increased further. Similar patterns have been shown in previous studies of Plasmodium yoelii infection.16 Quil A has an immunomodulatory ability17 and induces high levels of Thl-associated cytokines such as IL-2 and IFN-γ in malarial infection.16 Quil A also has the capacity to activate major histocompatibility complex (MHC) class-l-restricted cytotoxic T cells.17,18
We analyzed the various immune responses to rSAG3 vaccination to determine the mechanisms of protective immunity. IgG2a antibody levels of rSAG3- and rSAG3/Quil A-vaccinated mice significantly increased. IgG1 titers, however, were similar to those of uninfected control mice. The present data are similar to a previous report13 and suggest that rSAG3- or rSAG3/Quil A-vaccinated mice shift toward a more Th1-like immune response after immunization.19 Generally, in the immune response to T. gondii infection in mice, a Thl response is believed to play a key role in early protective immunity mainly by an IFN-γ driven CTL response.1,20 CD8+ T cells constitute the major cellular T cell subset involved in acquired immune protection against T. gondii.1,13,21 γδ T cells may play an important role in the early host response to infection with T. gondii.22 In this study, the percentage of CD8+ and γδ. T cells significantly increased in rSAG3- and rSAG3/Quil A-immunized mice, which suggests that rSAG3 alone or in combination with Quil A may provide an appropriate epitope for presentation on MHC-I, as well as stimulating the first line of host defense against intracellular pathogen infection.22,23 However, the percentage of CD4+ T cells after rSAG3 vaccination was similar to that of the control group. Efficient protection against T. gondii has been shown to be the result of synergy between CD8+ and CD4+ T cells.24,25 Therefore, this basal level of CD4+ T response may be one reason for the low level of protection in this experiment.
We observed the production of mRNA for selected Th1 (IFN-γ) and Th2 (IL-10) cytokines in splenocytes from each experimental group. It is well established that IFN-γ plays a predominant role in controlling both acute and chronic phases of T. gondii infection.1 IFN-γ mRNA production was higher in rSAG3/Quil A-immunized mice than in the rSAG3-immunized group, although both groups showed significant increases compared to the control group. However, IL-10 mRNA expression in rSAG3- and rSAG3/Quil A-immunized mice was not significantly different from the GST-, Quil A-, or GST/Quil A-immunized groups. These results indicate that immunization with rSAG3 alone can elicit an immune response,13 and that the addition of Quil A can accelerate this response toward Th1. Peritoneal macrophages are thought to serve as major effector cells against T. gondii. Murine peritoneal macrophages become activated after treatment with a combination of IFN-γ and TNF-α in vitro, and activated cells inhibit the intracellular replication of tachyzoites through the generation of NO by iNOS.26 In the present study, peritoneal macrophages from rSAG3- and rSAG3/Quil A-immunized mice produced significantly higher NO levels than the other groups. This suggests that rSAG3 alone or in combination with Quil A can stimulate antigen-presenting cells and that rSAG3- and rSAG3/Quil A-vaccinated mice are sufficiently primed by IFN-γ to inhibit the multiplication of parasites.17
Host immunity is likely related to various factors, including the route of challenge, mouse strain, antigen preparation, and adjuvant.27 The present study was undertaken to determine whether GST-fused T. gondii SAG3 protein (rSAG3) could elicit protective immunity against murine toxoplasmosis. Mice immunized with rSAG3 revealed significant changes in survival rate and parasite burdens. These findings may have been due to the significant increases in serum IgG2a antibody titers, IFN-γ mRNA expression, and percentage of CD8+ T cells in the spleens of rSAG3-vaccinated mice. Moreover, the vaccination of rSAG3 with Quil A further increased the survival rate after the T. gondii infection and parasite burdens in vivo and in vitro. These results indicate that vaccination with rSAG3 can induce partial immunity against T. gondii infection through the induction of a Th1-type immune response, and that this immunity is further increased by the immuno-modulating effects of Quil A.17,18
Figures and Tables
Fig. 1
SDS-PAGE and glutathione-S-transferase (GST) detection of the rSAG3 protein of T. gondii. Lane 1, isopropyl β-D-thiogalactopyranoside (IPTG) induced GST; lane 2, non-induced; lane 3, induced GST fusion SAG3 (rSAG3); lane 4, purified rSAG3.
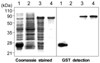
Fig. 2
Survival rates of BALB/c mice immunized with rSAG3 protein alone or in combination with Quil A after oral infection with a lethal dose of the T. gondii Me49 strain (1,500 cysts per mouse). Mice were observed daily until 4 weeks after T. gondii infection. Each group consisted of 10 mice.
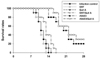
Fig. 3
Brain cyst load of mice immunized with rSAG3 alone or in combination with Quil A. Three weeks after the last immunization, mice were orally challenged with a nonlethal dose of the T. gondii Me49 strain (20 cysts per mouse) and evaluated 4 weeks after infection. IC, infection control group; G, GST-immunized group; Q, Quil A-immunized group; G/Q, GST/Quil A-immunized group; rS3, GST fusion SAG3 (rSAG3)-immunized group; rS3/Q, rSAG3/Quil A-immunized group. Data are represented as the mean ± SE of 5 mice.
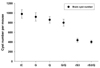
Fig. 4
IgG, IgG1 and IgG2a titers of sera from mice immunized with rSAG3 alone or in combination with Quil A. Sera from vaccinated mice were obtained and IgG subclasses measured by ELISA. C, untreated control group. The data are represented as the mean ± SE of 5 mice and are representative one of two separate experiments.
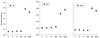
Fig. 5
Proliferative responses of splenocytes from mice immunized with rSAG3 protein alone or in combination with Quil A. Spleen cells were cultured in the presence of Toxoplasma lysate antigen (TLA) for 72h. Proliferation was assayed by [3H]-thymidine incorporation. The data are represented as the mean ± SE of 5 mice and are representative of two separate experiments.
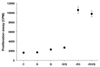
Fig. 6
Percentages of T cell subsets in splenocytes from mice immunized with rSAG3 protein alone or in combination with Quil A. Splenocytes were stained with FITC-conjugated CD4, CD8 or γδ mAb, and then analyzed by FACScan. The data are represented as the mean ± SE of 5 mice and are representative of two separate experiments.
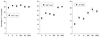
Fig. 7
Relative IFN-γ, TNF-α and IL-10 mRNA expression levels in splenocytes from mice immunized with rSAG3 alone or in combination with Quil A. Differences in the transcriptional level of all genes are expressed relative to the control group (assigned as 1). The data are represented as the mean ± SE of 5 mice and are representative of two separate experiments.
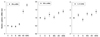
Fig. 8
Nitric oxide production in peritoneal macrophages from mice immunized with rSAG3 alone or in combination with Quil A. Culture supernatants were obtained from macrophage monolayers, and nitrite levels were measured by Griess assay. The data are represented as the mean ± SE of 5 mice and are representative of two separate experiments.
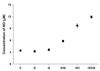
References
1. Filisetti D, Candolfi E. Immune response to Toxoplasma gondii. Ann 1st Super Sanita. 2004. 40:71–80.
2. Lekutis C, Ferguson DJ, Grigg ME, Camps M, Boothroyd JC. Surface antigens of Toxoplasma gondii: variations on a theme. Int J Parasitol. 2001. 31:1285–1292.
3. Kasper LH, Crabb JH, Pfefferkorn ER. Purification of a major membrane protein of Toxoplasma gondii by immunoabsorption with a monoclonal antibody. J Immunol. 1983. 130:2407–2412.
4. Parmley SF, Sgarlato GD, Mark J, Prince JB, Remington JS. Expression, characterization, and serologic reactivity of recombinant surface antigen P22 of Toxoplasma gondii. J Clin Microbiol. 1992. 3:1127–1133.
5. Cesbron-Delauw MF, Tomavo S, Beauchamps P, Fourmaux MP, Camus D, Capron A, et al. Similarities between the primary structures of two distinct major surface proteins of Toxoplasma gondii. J Biol Chem. 1994. 269:16217–16222.


6. Letscher-Bru V, Villard O, Risse B, Zauke M, Klein JP, Kien TT. Protective effect of vaccination with a combination of recombinant surface antigen 1 and interleukin-12 against toxoplasmosis in mice. Infect Immun. 1998. 66:4503–4506.


7. Couper KN, Nielsen HV, Petersen E, Roberts F, Roberts CW, Alexander J. DNA vaccination with the immunodominant tachyzoite surface antigen (SAG-1) protects against adult acquired Toxoplasma gondii infection but does not prevent maternofoetal transmission. Vaccine. 2003. 21:2813–2820.


8. Mohamed RM, Aosai F, Chen M, Mun HS, Norose K, Belal US, et al. Induction of protective immunity by DNA vaccination with Toxoplasma gondii HSP70, HSP30 and SAG1 genes. Vaccine. 2003. 21:2852–2861.


9. Mevelec MN, Bout D, Desolme B, Marchand H, Magne R, Bruneel O, et al. Evaluation of protective effect of DNA vaccination with genes encoding antigens GRA4 and SAG1 associated with GM-CSF plasmid, against acute, chronical and congenital toxoplasmosis in mice. Vaccine. 2005. 23:4489–4499.


10. Kasper LH, Currie KM, Bradley MS. An unexpected response to vaccination with a purified major membrane tachyzoite antigen (P30) of Toxoplasma gondii. J Immunol. 1985. 134:3426–3431.
11. Jacquet A, Coulon L, De Neve J, Daminet V, Haumont M, Garcia L, et al. The surface antigen SAG3 mediates the attachment of Toxoplasma gondii to cell-surface proteoglycans. Mol Biochem Parasitol. 2001. 116:35–44.


12. Dzierszinski F, Mortuaire M, Cesbron-Delauw MF, Tomavo S. Targeted disruption of the glycosylphosphatidylinositol-anchored surface antigen SAG3 gene in Toxoplasma gondii decreases host cell adhesion and drastically reduces virulence in mice. Mol Microbiol. 2000. 37:574–582.


13. Caetano BC, Bruna-Romero O, Fux B, Mendes EA, Penido ML, Gazzinelli RT. Vaccination with replication-deficient recombinant adenoviruses encoding the main surface antigens of Toxoplasma gondii induces immune response and protection against infection in mice. Hum Gene Ther. 2006. 17:415–426.


14. Deckert-Schluter M, Albrecht S, Hof H, Wiestler OD, Schluter D. Dynamics of the intracerebral and splenic cytokine mRNA production in Toxoplasma gondii-resistant and -susceptible congenic strains of mice. Immunology. 1995. 85:408–418.
15. Hayashi S, Chan CC, Gazzinelli R, Roberge FG. Contribution of nitric oxide to the host parasite equilibrium in toxoplasmosis. J Immunol. 1996. 156:1476–1481.
16. Burns JM Jr, Dunn PD, Russo DM. Protective immunity against Plasmodium yoelii malaria induced by immunization with particulate blood-stage antigens. Infect Immun. 1997. 65:3138–3145.


17. Behboudi S, Morein B, Villacres-Eriksson MC. Quillaja saponin formulations that stimulate proinflammatory cytokines elicit a potent acquired cell-mediated immunity. Scand J Immunol. 1999. 50:371–377.


18. Morein B, Bengtsson KL. Immunomodulation by iscoms, immune stimulating complexes. Methods. 1999. 19:94–102.


19. Nguyen TD, Bigaignon G, Van Broeck J, Vercammen M, Nguyen TN, Delmee M, et al. Acute and chronic phases of Toxoplasma gondii infection in mice modulate the host immune responses. Infect Immun. 1998. 66:2991–2995.


20. Aliberti J. Host persistence: exploitation of anti-inflammatory pathways by Toxoplasma gondii. Nat Rev Immunol. 2005. 5:162–170.


21. Wang X, Claflin J, Kang H, Suzuki Y. Importance of CD8(+)Vβ8(+) T cells in IFNγ-mediated prevention of toxoplasmic encephalitis in genetically resistant BALB/c mice. J Interferon Cytokine Res. 2005. 25:338–344.


22. Abou-Bacar A, Pfaff AW, Letscher-Bru V, Filisetti D, Rajapakse R, Antoni E, et al. Role of gamma interferon and T cells in congenital Toxoplasma transmission. Parasite Immunol. 2004. 26:315–318.


23. Denkers EY. T lymphocyte-dependent effector mechanisms of immunity to Toxoplasma gondii. Microbes Infect. 1999. 1:699–708.


24. Casciotti L, Ely KH, Williams ME, Khan IA. CD8+-T-cell immunity against Toxoplasma gondii can be induced but not maintained in mice lacking conventional CD4+ T cells. Infect Immun. 2002. 70:434–443.


25. Rodrigues MM, Boscardin SB, Vasconcelos JR, Hiyane MI, Salay G, Soares IS. Importance of CD8 T cell-mediated immune response during intracellular parasitic infections and its implications for the development of effective vaccines. An Acad Bras Cienc. 2003. 75:443–468.


26. Luder CG, Algner M, Lang C, Bleicher N, Gross U. Reduced expression of the inducible nitric oxide synthase after infection with Toxoplasma gondii facilitates parasite replication in activated murine macrophages. Int J Parasitol. 2003. 33:833–844.
27. Dumont AR, Kalfayan LH, Sekaly RP. Modulation of immune responses-strategies for optimising vaccines. Expert Opin Biol Ther. 2004. 4:627–630.