Abstract
Purpose
Diabetic nephropathy is the most serious of complications in diabetes mellitus. Thiazolidinedione (TZD) is thought to ameliorate diabetic nephropathy; however, the mechanism underlying this effect has not been elucidated. We hypothesized that the vascular endothelial growth factor (VEGF) participates in the pathogenesis of diabetic nephropathy and that TZD may be beneficial for the treatment of diabetic nephropathy because of the effect it has on VEGF.
Materials and Methods
23 Otsuka-Long-Evans-Tokushima-Fatty (OLETF) rats and eight control Long-Evans-Tokushima-Otsuka (LETO) rats were divided into the following four groups: LETO group, control OLETF group, pioglitazone treated group (10 mg/kg/day), and rosiglitazone treated group (3 mg/kg/day).
Results
A progressive increase in urinary protein excretion was observed in the diabetic rats. Glomerular VEGF expression in the control OLETF rats was significantly higher than in the control LETO rats. However, there was a significant reduction in both the glomerular VEGF expression and the VEGF mRNA levels after treatment with pioglitazone and rosiglitazone. The twenty-four hour urine protein levels were significantly decreased in both groups of the treated OLETF rats.
Diabetic nephropathy is one of the most serious microvascular complications. The vascular endothelial growth factor (VEGF) pathway has recently been proposed to play a role in the development of diabetic renal changes in diabetic animal models.1,2 VEGF is a potent mitogen and chemoattractant for endothelial cells and acts as a key modulator of angiogenesis in physiological and pathological conditions. This particular growth factor also acts as a vascular factor that increases microvascular permeability.3 VEGF is increased during the early stages of diabetic nephropathy in type 2 diabetic rats.4 Inhibition of VEGF by the administration of neutralizing antibodies was observed to abolish hyperfiltration and suppress the urinary albumin excretion rate in diabetic rats.5
Thiazolidinediones (TZDs) are a new class of oral antidiabetic agents that improve insulin sensitivity and act as selective and potent agonists of the peroxisome proliferator-activated receptor-γ. Studies have reported other effects of TZDs that seem to be independent of their ability to lower plasma glucose levels. Several studies have reported that TZDs ameliorated urinary albumin excretion in type 2 diabetes.6-8 TZDs also have an inhibitory effect on VEGF expression in vitro.9
Based on these observations, we hypothesized that the elevated VEGF production in diabetes mellitus exacerbates proteinuria by increasing the glomerular vascular permeability and that TZDs may ameliorate diabetic nephropathy by inhibiting VEGF expression. In this study, we investigated VEGF expression in diabetic rat kidneys and determined whether TZDs inhibit renal VEGF expression and ameliorate diabetic nephropathy.
Twenty-three 8-week-old Otsuka-Long-Evans-Tokushima-Fatty (OLETF) male rats, a model of type 2 diabetes mellitus, were assigned to different treatment groups. Eight Long-Evans-Tokushima-Otsuka[EWE-DT21] (LETO) male rats served as the genetic control group. Both the diabetic and the control rats were further selected at random to receive pioglitazone, AD4833 (Eli Lilly & Co., IN, USA), at a dose of 10 mg/kg body weight/day or rosiglitazone at a dose of 3 mg/kg body weight/day, which was added to their drinking water. The remaining rats received untreated water. All rats were kept under an artificial light cycle at a controlled temperature (23 ± 2℃) and humidity (55 ± 5%) and were given free access to rat chow diet. Plasma glucose concentrations were checked every other week by using the glucose oxidase technique. Animals were caged individually, and their weights were checked at 10, 22, 30, 40 and 50 weeks. Twenty-four hour urine protein levels were measured by an Autokit MicroTP on a Hitachi 7170 analyzer (Hitachi, Japan)10 from each animal at 22, 30, 40 and 50 weeks. At 50 weeks, all rats were sacrificed and their kidneys were harvested.
The OLETF rats and the age-matched LETO rats were anesthetized with ketamine (60 mg/kg i.p. [PC3]). Kidneys were perfused with phosphate-buffered saline (pH 7.4) through the aorta, rapidly fixed in 4% paraformaldehyde for 24 hours, and embedded in paraffin. One kidney was processed for immunohistochemical study and histologic examination. The other kidney was immediately placed in liquid nitrogen for subsequent RNA extraction. Paraffin slices from the kidneys were stained with hematoxylin and eosin. The glomerular cross-sectional area was measured in 100 glomerular profiles per rat using a microcomputer imaging device (SIS, Munster, Germany), and the glomerular volume (VG) was calculated according to the method described by Weibel and Gomez11: VG=Area1.5 × (1.38/1.01), where 1.38 is the shape coefficient for a sphere and a 1.01 is the size distribution coefficient assuming a coefficient of variation of 10%.
For the immunohistochemical staining, the renal tissue was immediately fixed in 4% paraformaldehyde, cast in paraffin, sliced into 5 µm-thick sections, and placed on microscope slides. The sections were deparaffinized with xylene and rehydrated in alcohol at a graded series of concentrations. Endogenous peroxidase was inactivated by incubating the samples in a using 3% hydrogen peroxide in methyl alcohol for 10 min[M4]. The kidney sections were incubated for 1 hour at room temperature with the monoclonal anti-VEGF antibody (1 : 100) (#sc-7269, mouse antibody, Santa Cruz Biotechnology, CA, USA). The DAB Reagent kit was used to visualize the immunoreactivity (#87-8963, Zymed, South San Francisco, CA, USA) for 20 min at room temperature. The expression of VEGF was assessed by densitometric evaluation of the images by computer-assisted image analysis. Data are expressed as the percentage optical density relative to the control kidneys, which were assigned a value of 100. All analyses were performed with the observer blind to the animal study group.
Total RNA was extracted from renal cortical tissues with TRIzol reagent (Life Technologies. Gaithersburg, MD, USA), and the cDNA was synthesized by a reverse transcription reaction of 2 µg of total RNA with oligo-(dT) primers (Life Technologies). Next, 2 µL of cDNA was amplified by Taq DNA polymerase, and the PCR profile consisted of an initial denaturation at 94℃ for 3 min, followed by 35 cycles of denaturation at 94℃ for 30 s, annealing at 58℃ for 30 s, and an extension at 72℃ for 90 s, which was followed by a final extension at 72℃ for 7 min. Rat VEGF primers, which amplify three splicing variants of rat VEGF mRNA (VEGF120, VEGF164, and VEGF188), were used. The expected lengths of the resulting PCR products are 330 base pairs (bp) for VEGF120, 462 bp for VEGF164, and 514bp for VEGF188. The nucleotide sequences of each primer are as follows: sense 5'-GACCCTGGTGGACATCTTCCAGGA-3' and antisense 5'-GGTGAGAGGTCTAGTTCCCG A-3'. β2-microglobulin was also amplified as an internal control and the nucleotide sequences of these primers are as follows: sense 5'-CAGATCT GTCCTTCAGCAAG-3' and antisense 5'-GGAGTA AACTGGTCCAGATG-3'. Different numbers of PCR cycles were performed and plotted against the densitometry measurements of the resulting PCR products to define the range in which the cycle number was linearly related to the amount of PCR-amplified product. Next, 10 µL of the PCR reaction products were separated by electrophoresis on a 1.5% agarose gel stained with ethidium bromide. PCR product bands, visualized by ultraviolet light, were scanned at 300 dots per inch and densitometric analysis was performed with the National Institutes of Health Image 1.61 software which is available with the macro and linear regression analyzer. To confirm the identity of the VEGF cDNA product, each of the electrophoresed PCR bands was extracted with a DNA extraction kit (Qiagen, Valencia, CA, USA) and sequenced using an ABI automated DNA sequencing system (ABI Genetic Analyzer 310; PRISM, Branchburg Park, NJ, USA). Quantitation of the VEGF mRNA expression was corrected by the β2-microglobulin.
The body weights of the age-matched OLETF rats independent of TZD treatment were significantly higher than the LETO rats throughout the study period (Table 1). Plasma glucose levels were higher in the OLETF rats during the study periods, and there was a statistically significant difference after 30 weeks of age between the age-matched OLETF rats and the LETO rats, independent of TZD treatment (Table 1). In the control OLETF rats, 24-hour urine protein levels were significantly higher than in the LETO rats at 40 and 50 weeks. In the OLETF rats treated with pioglitazone and rosiglitazone, the 24-hour urine protein levels were significantly decreased compared to the control OLETF rats (Table 1).
Whereas hematoxylin and eosin staining revealed normal glomeruli in the LETO rats, glomerular expansions were observed in control OLETF rats (Fig. 1). The glomerular volumes of control OLETF rats were significantly increased when compared to the control LETO rats. The glomerular volumes were significantly decreased in the OLETF rats treated with pioglitazone and rosiglitazone when compared to the control OLETF rats (pioglitazone 6.2% vs. rosiglitazone 5.8%) (Fig. 2).
The immunohistochemical stain for VEGF in the glomerular area showed a darker brown pigmentation in the control OLETF rats than in the other groups (Fig. 3). The glomerular VEGF expression in control OLETF rats was significantly increased when compared to the LETO rats. The glomerular VEGF expression was significantly decreased in the OLETF rats treated with pioglitazone and rosiglitazone when compared to the control OLETF rats (pioglitazone 6.2% vs. rosiglitazone 7.5% decrease, which was marginal but statistically significant) (Fig. 4).
Both of the glomerular VEGF and β2-MG mRNA expression were analyzed by RT-PCR. The mRNA expressions of the VEGF isoforms (VEGF 120, VEGF164, and VEGF188) were significantly increased in the control OLETF rats compared to the LETO rats. The OLETF rats treated with pioglitazone and rosiglitazone had decreased mRNA levels of the VEGF isoforms (Fig. 5). Expression of the β2-MG was identified at nearly constant levels.
Our study suggests that quantitative and qualitative changes in the glomerular VEGF expression are present in diabetic rats and that increased renal VEGF expression and urinary protein excretion are abolished by in vivo treatment with TZDs.
Diabetic nephropathy is the leading cause of end-stage renal disease. In addition, patients with type 2 diabetes undergoing maintenance dialysis have a poor prognosis because of the increased risk for cardiovascular events.12 To prevent the development and progression of diabetic nephropathy, a comprehensive understanding of the pathophysiology of diabetic nephropathy is needed. Although it was initially thought that renal injury in diabetic nephropathy is caused primarily by hemodynamic alterations, such as hyperfiltration and hyperperfusion,13 there is now clear evidence that these changes are only one aspect of a complex series of pathophysiological alterations caused by disturbed glucose homeostasis. Recently, better insights into the pathogenesis of diabetic nephropathy on a molecular level have been provided. High glucose levels stimulate the synthesis of angiotensinogen and angiotensin II.14 These polypeptides exert hemodynamic, inflammatory and trophic effects on renal cells. On the other hand, shear stress and mechanical strain, resulting from altered glomerular hemodynamics and glomerular hypertension, induce the autocrine and/or paracrine release of cytokines and growth factors,15 which in turn plays a central role in the development of diabetic nephropathy. Of these growth factors, VEGF was implicated in the pathogenesis of diabetic nephropathy because many studies have reported that the VEGF mRNA expression is increased in diabetic nephropathy,1,4,16 and that chronic inhibition of VEGF by anti-VEGF antibodies was associated with amelioration in albuminuria and diabetic glomerular lesions.5,17 Although the role of VEGF in diabetic nephropathy has not yet been identified, clinical and experimental data indicate that the VEGF may be involved in both the stimulation of collagenase production18 and the nitric oxide production in endothelial cells.19 VEGF also alters glomerular permeability20,21 and increases the glomerular filtration surface area by augmenting the glomerular capillary endothelial cell growth.22
In this study, significantly increased 24-hour urine protein levels and glomerular expansion were observed in diabetic rats. Increased glomerular VEGF immunostain and VEGF mRNA expression were also observed in the diabetic rats. These findings suggested that the VEGF may play a causal role in the pathogenesis of diabetic nephropathy.
TZDs are drugs that decrease insulin resistance by enhancing the action of insulin at the receptor cell level.23 Yoshimoto et al.24 reported that pioglitazone was effective in correcting not only glucose metabolism, but also cardiovascular and renal complications in rats. Moreover, TZDs improved microalbuminuria in diabetic nephropathy.7,8,25,26 However, the precise mechanisms have not yet been elucidated. Recently, Isshiki et al.27 suggested that TZDs prevent the activation of the diacylglycerol (DAG)-protein kinase C pathway and activated the DAG kinase under high glucose conditions. Pistrosch et al.8 reported that TZDs ameliorated renal glomerular endothelial dysfunction, and Agarwal28 suggested that TZDs affected diabetic nephropathy through anti-inflammatory reactions. Interestingly, there was evidence that high glucose concentrations promoted renal mesangial cell proliferation by the increased production of VEGF and that TZDs had inhibitory effects on renal VEGF expression in renal mesangial cells in vitro.9
In our study, there was no significant difference in serum glucose levels between the control and the TZD treated OLETF rats. The 24-hour urine protein excretion and the renal VEGF expression were significantly decreased in the OLETF rats treated with TZDs. These results suggest that the TZDs ameliorate diabetic nephropathy independent of glycemic control by reducing both the renal VEGF expression and the urinary albumin excretion.
Peroxisome proliferator activated receptors (PPARs) are nuclear receptors that function as transcriptional regulators of glucose and lipid metabolism.29 PPARγ is the molecular target for thiazolidinediones such as pioglitazone and rosiglitazone[PC5] used in this study. The pioglitazone is almost ten times less potent as an activator of the human PPARγ than the rosiglitazone,30 but both of these agents are similarly potent in their antiatherogenic effects.31 To date there have been no studies that have compared the effects of pioglitazone and rosiglitazone on diabetic nephropathy. In our study, pioglitazone and rosiglitazone had similar effects on both the renal VEGF expression and the urinary protein excretion in the OLETF rats. These findings suggest that pioglitazone and rosiglitazone have similar effects on diabetic nephropathy.
In conclusion, VEGF is overexpressed in diabetic glomeruli and is associated with proteinuria. The treatment with TZDs, either with pioglitazone or rosiglitazone, abolished the VEGF overexpression in diabetic rats. These findings suggest that VEGF may play a crucial role in the pathogenesis of diabetic nephropathy and that TZDs may have beneficial effects on diabetic nephropathy by reducing the renal VEGF expression.
Figures and Tables
Fig. 1
Hematoxylin and eosin staining of the glomeruli from LETO rats (A), control OLETF rats (B), pioglitazone treated OLETF rats (C), and rosiglitazone treated OLETF rats (D). Scale bar, 100 µm. The glomerular expansions were observed in the control OLETF rats.
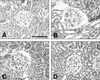
Fig. 2
Changes in the glomerular volumes. The glomerular volumes of the control OLETF rats were significantly increased when compared with the LETO rats. After treatment with pioglitazone and rosiglitazone, the glomerular volumes significantly decreased in the control OLETF rats. [PC6]*p < 0.05 vs. control OLETF.
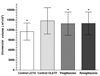
Fig. 3
Immunohistochemical stain for glomerular VEGF from LETO rats (A), control OLETF rats (B), pioglitazone treated OLETF (C), and rosiglitazone treated OLETF (D). Scale bar, 100 µm. In the control OLETF rats, the brown pigmentation was darker than in the other groups.
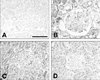
Fig. 4
Changes in the optical density of the glomerular VEGF immunoreactivity. Compared with LETO rats, glomerular VEGF expression of the control OLETF rats was significantly increased. Glomerular VEGF expression was significantly decreased when compared to the control OLETF group after treatment with pioglitazone and rosiglitazone. *p < 0.05 vs. control OLETF.
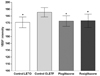
Fig. 5
Comparison of the glomerular VEGF mRNA expression. The expected lengths of the PCR products are 330 base pairs (bp) for VEGF120, 462bp for VEGF164, and 514bp for VEGF188. The mRNA expressions of VEGF isoforms (VEGF120, VEGF164, and VEGF188) were significantly increased in the control OLETF rats when compared to the expression levels in the LETO rats. After treatment with pioglitazone and rosiglitazone, the levels of VEGF isoforms decreased to levels observed in LETO rats. Expression of β2-MG was identified at nearly constant levels.
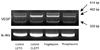
References
1. Cooper ME, Vranes D, Youssef S, Stacker SA, Cox AJ, Rizkalla B, et al. Increased renal expression of vascular endothelial growth factor (VEGF) and its receptor VEGFR-2 in experimental diabetes. Diabetes. 1999. 48:2229–2239.
2. Tsuchida K, Makita Z, Yamagishi S, Atsumi T, Miyoshi H, Obara S, et al. Suppression of transforming growth factor beta and vascular endothelial growth factor in diabetic nephropathy in rats by a novel advanced glycation end product inhibitor, OPB-9195. Diabetologia. 1999. 42:579–588.
3. Ferrara N. Role of vascular endothelial growth factor in the regulation of angiogenesis. Kidney Int. 1999. 56:794–814.
4. Cha DR, Kang YS, Han SY, Jee IH, Han KH, Han JY, et al. Vascular endothelial growth factor is increased during early stage of diabetic nephropathy in type II diabetic rats. J Endocrinol. 2004. 183:183–194.
5. de Vriese AS, Tilton RG, Elger M, Stephan CC, Kriz W, Lameire NH. Antibodies against vascular endothelial growth factor improve early renal dysfunction in experimental diabetes. J Am Soc Nephrol. 2001. 12:993–1000.
6. Imano E, Kanda T, Nakatani Y, Nishida T, Arai K, Motomura M, et al. Effect of troglitazone on microalbuminuria in patients with incipient diabetic nephropathy. Diabetes Care. 1998. 21:2135–2139.
7. Nakamura T, Ushiyama C, Osada S, Hara M, Shrimada M, Koide H. Pioglitazone reduces urinary podocyte excretion in type 2 diabetes patients with microalbuminuria. Metabolism. 2001. 50:1193–1196.
8. Pistrosch F, Herbrig K, Kindel B, Passauer J, Fischer S, Gross P. Rosiglitazone improves glomerular hyperfiltration, renal endothelial dysfunction, and microalbuminuria of incipient diabetic nephropathy in patients. Diabetes. 2005. 54:2206–2211.
9. Onozaki A, Midorikawa S, Sanada H, Hayashi Y, Baba T, Katoh T, et al. Rapid change of glucose concentration promotes mesangial cell proliferation via VEGF: inhibitory effects of thiazolidinedione. Biochem Biophys Res Commun. 2004. 317:24–29.
10. Lynch KM, Sellers TS, Gossett KA. Evaluation of an automated pyrogallol red-molybdate method for the measurement of urinary protein in rats. Eur J Clin Chem Clin Biochem. 1996. 34:569–571.
11. Lane PH, Steffes MW, Mauer SM. Estimation of glomerular volume: a comparison of four methods. Kidney Int. 1992. 41:1085–1089.
12. Remuzzi G, Schieppati A, Ruggenenti P. Clinical practice: nephropathy in patients with type 2 diabetes. N Engl J Med. 2002. 346:1145–1151.
13. Ziyadeh FN. The extracellular matrix in diabetic nephropathy. Am J Kidney Dis. 1993. 22:736–744.
14. Wang TT, Wu XH, Zhang SL, Chan JS. Effect of glucose on the expression of the angiotensinogen gene in opossum kidney cells. Kidney Int. 1998. 53:312–319.
15. Wolf G, Ziyadeh FN. The role of angiotensin II in diabetic nephropathy: Emphasis on nonhemodynamic mechanisms. Am J Kidney Dis. 1997. 29:153–163.
16. Lee EY, Shim MS, Kim MJ, Hong SY, Shin YG, Chung CH. Angotensin II receptor blocker attenuates overexpression of vascular endothelial growth factor in diabetic podocytes. Exp Mol Med. 2002. 36:65–70.
17. Flyvbjerg A, Dagnaes-Hansen F, De Vriese AS, Schrijvers BF, Tilton RG, Rasch R. Amelioration of long-term renal changes in obese type 2 diabetic mice by a neutralizing vascular endothelial growth factor antibody. Diabetes. 2002. 51:3090–3094.
18. Unemori EN, Ferrara N, Bauer EA, Amento EP. Vascular endothelial growth factor induces interstitial collagenase expression in human endothelial cells. J Cell Physiol. 1992. 153:557–562.
19. van der Zee R, Murohara T, Luo Z, Zollmann F, Passeri J, Lekutat C, et al. Vascular endothelial growth factor/vascular permeability factor augments nitric oxide release from quiescent rabbit and human vascular endothelium. Circulation. 1997. 95:1030–1037.
20. Shulman K, Rosen S, Tognazzi K, Manseau EJ, Brown LF. Expression of vascular permeability factor (VPF/VEGF) is altered in many glomerular diseases. J Am Soc Nephrol. 1996. 7:661–666.
21. Horita Y, Miyazaki M, Koji T, Kobayashi N, Shibuya M, Razzaque MS, et al. Expression of vascular endothelial growth factor and its receptors in rats with protein overload nephrosis. Nephrol Dial Transplant. 1998. 13:2519–2528.
22. Nyengaard JR, Rasch R. The impact of experimental diabetes mellitus in rats on glomerular capillary number and sizes. Diabetologia. 1993. 36:189–194.
23. Henry RR. Thiazolidinediones. Endocrinol Metab Clin North Am. 1997. 26:553–573.
24. Yoshimoto T, Naruse M, Nishikawa M, Naruse K, Tanabe A, Seki T, et al. Antihypertensive and vasculo- and renoprotective effects of pioglitazone in genetically obese diabetic rats. Am J Physiol. 1997. 272:E989–E996.
25. Nakamura T, Ushiyama C, Suzuki S, Shimada N, Sekizuka K, Ebihara L, et al. Effect of troglitazone on urinary albumin excretion and serum type IV collagen concentrations in Type 2 diabetic patients with microalbuminuria or macroalbuminuria. Diabet Med. 2001. 18:308–313.
26. Bakris G, Viberti G, Weston WM, Heise M, Porter LE, Freed MI. Rosiglitazone reduces urinary albumin excretion in type II diabetes. J Hum Hypertens. 2003. 17:7–12.
27. Isshiki K, Haneda M, Koya D, Maeda S, Sugimoto T, Kikkawa R. Thiazolidinedione compounds ameliorate glomerular dysfunction independent of their insulin-sensitizing action in diabetic rats. Diabetes. 2000. 49:1022–1032.
28. Agarwal R. Anti-inflammatory effects of short-term pioglitazone therapy in men with advanced diabetic nephropathy. Am J Physiol Renal Physiol. 2006. 290:F600–F605.
29. Martens FM, Visseren FL, Lemay J, de Koning EJ, Rabelink TJ. Metabolic and additional vascular effects of thiazolidinediones. Drugs. 2002. 62:1463–1480.
30. Sakamoto J, Kumura H, Moriyama S, Odaka H, Momose Y, Sugiyama Y, et al. Activation of human peroxisome proliferator-activated receptor (PPAR) subtypes by pioglitazone. Biochem Biophys Res Commun. 2000. 278:704–711.
31. Hirakata M, Tozawa R, Imura Y, Sugiyama Y. Comparison of the effects of pioglitazone and rosiglitazone on macrophage foam cell formation. Biochem Biophys Res Commun. 2004. 323:782–788.