Abstract
Purpose
Methionine synthase (MTR) and 5,10-methylenetetrahydrofolate reductase (MTHFR) are the main regulatory enzymes for homocysteine metabolism. The present case-control study was conducted to determine whether there is an association between the MTR 2756A > G or MTHFR 677C > T polymorphism and plasma homocysteine concentration in Korean subjects with ischemic stroke.
Materials and Methods
DNA samples of 237 patients who had an ischemic stroke and 223 age and sex-matched controls were studied. MTR 2756A > G and MTHFR 677C > T genotypes were determined by polymerase chain reaction-restriction fragment length polymorphism (PCR-RFLP).
Results
Frequencies of mutant alleles for MTR and MTHFR polymorphisms were not significantly different between the controls and cases. The patient group, however, had significantly higher homocysteine concentrations of the MTR 2756AA and MTHFR 677TT genotypes than the control group (p = 0.04 for MTR, p = 0.01 for MTHFR). The combined MTR 2756AA and MTHFR 677TT genotype (p = 0.04) and the homocysteine concentrations of the patient group were also higher than those of the controls. In addition, the genotype distribution was significant in the MTHFR 677TT genotype (p = 0.008) and combined MTR 2756AA and MTHFR 677TT genotype (p = 0.03), which divided the groups into the top 20% and bottom 20% based on their homocysteine levels.
Methionine synthase (MTR) plays an important catalytic role in a methylcobalamin-dependent reaction, along with 5,10-methylenetetrahydrofolate reductase (MTHFR), for the remethylation of homocysteine to methionine.1,2 Many studies have shown that differences in the plasma's total homocysteine (tHcy) and folate levels are associated with variation of the MTHFR genotype.3-9 The MTHFR polymorphism, which is associated with a predisposition for elevated plasma concentrations of homocysteine, has been reported to represent a genetic risk factor for occlusive vascular diseases, carotid atherosclerosis, silent brain infarction and small artery occlusion with ischemic stroke, although these associations remain controversial.9-22
A mild deficiency in MTR activity may be associated with mild hyperhomocysteinemia, a risk factor for vascular diseases and possibly neural tube defects (NTD). The gene encoding human MTR has been cloned and sequenced.23 The human MTR gene has been mapped to chromosome 1q43 and encodes a product of 1265 amino acids. The 2756A > G mutation was identified for the first time in patients with the cblG complementation group of inherited homocysteine/folate disorders; it also exists in the general population.23
Recent reports have suggested that there is a strong positive association between MTR 2756GG genotype and coronary heart disease (CHD) risk in the Dutch population,24 although other studies have reported that the 2756GG genotype has a protective rather than an adverse effect on CHD.25-27 The MTR 2756A > G polymorphism has also been found to be associated with a lower risk of colorectal cancer among individuals with low alcohol consumption.28 Infants who carry mutant alleles for both methionine synthase reductase (MTRR 66A > G) and MTR 2756A > G polymorphisms have an associated increased risk for NTDs29 and thrombotic events.30 In contrast, other investigators have reported no association between the MTR polymorphism and ischemic stroke, CHD, or NTD in case-control studies.2,31-33 Ischemic stroke is a multifactorial disorder, in which genetic and environmental factors, including defects in homocysteine metabolism, play a major role. Investigations of the MTR 2756A > G polymorphism, in association with a variety of disorders, has been reported most commonly in Caucasian populations, while few investigations have focused on non-Caucasian groups.
In the present study, we investigated whether the MTR 2756A > G and MTHFR 677C > T polymorphisms are associated with the homocysteine concentration and whether there is an increased risk for ischemic stroke associated with these polymorphisms.
The study population was enrolled from November 2000 to May 2002 at Bundang CHA Hospital, College of Medicine, Pochon CHA University. Ischemic stroke was defined as a clinical syndrome characterized by rapidly developing clinical symptoms and signs of focal and, at times, global loss of brain function with evidence of a cerebral infarction in the clinically relevant area of the brain identified by brain imaging studies.10 The diagnosis of ischemic stroke was made when neurological deficits were accompanied by corresponding abnormal magnetic resonance imaging (MRI) findings of the brain, interpreted by two independent experienced neurologists. Ischemic stroke was excluded when researchers did not agree; patients with cerebral hemorrhage were excluded in advance.
For the control subjects, we selected healthy individuals matched for gender and age from individuals attending wellness examinations at Bundang CHA Hospital during the same period, who were without a history of cerebrovascular disease or myocardial infarction. Baseline demographic data and a history of conventional vascular risk factors were obtained for each control subject. Some of the participants, both ischemic stroke patients and controls, were diagnosed with hypertension or diabetes mellitus at the time of enrollment. The institutional review board at Bundang CHA Hospital approved this study in June 2000. All patients and controls gave written informed consent.
Total genomic DNA was prepared from whole blood after lysis of red blood cells.34 The areas spanning the polymorphic sites of MTR 2756A > G and MTHFR 677C > T were amplified by the polymerase chain reaction (PCR) from genomic DNA using the primers and reaction conditions described previously.6,23 The polymorphisms were identified following the digestion of amplified DNA with the endonucleases HaeIII and HinfI for MTR and MTHFR genes. Alleles of each polymorphic site were classified depending on the presence or absence of a restriction enzyme cutting site.
Specimens were collected to measure levels of homocysteine, folate, and vitamin B12 within 48 hrs after the onset of a stroke. Blood was collected in a tube containing anticoagulant 12 hours after the patient's last meal. The tube was centrifuged for 15 minutes at 1,000 g and the plasma separated. The concentration of homocysteine in the plasma was measured by fluorescent polarizing immunoassay (FPIA) with IMx (Abbott, USA). The plasma concentration of folate was determined using a radioassay kit.35
All statistical analyses were performed using the Statistical Analysis System software (SAS Institute, Inc., Cary, NC, USA). To analyze baseline characteristics, we used a two-sample t test for continuous data (age and fasting plasma homocysteine and folate concentrations) when comparing patient and control baseline data. The distribution of genotypes was tested for Hardy-Weinberg equilibrium and for selection against a particular genotype by using the Chi-square test. Stratification analysis was used to subgroup age and gender. One-way analysis of variance (ANOVA) was performed to compare the mean concentrations of plasma homocysteine and folate among three genotypes. Statistical significance was accepted at a p = 0.05 level.
As shown in Table 1, the ischemic stroke group had a significantly higher homocysteine concentration than the controls (p = 0.03). There were, however, no significant differences between cases and controls for age and folate concentration. The genotype distribution for the MTR 2756A > G polymorphic locus did not deviate significantly from Hardy-Weinberg equilibrium in both groups (Table 2). The allele frequencies were not significantly different between the controls and cases. The odds ratios for the MTR 2756AG and the 2756GG genotypes with 2756AA were 0.94 (95% CI, 0.59-1.48; p = 0.78) and 1.43 (95% CI, 0.23-8.71; p = 0.70) in the controls and cases, respectively.
We compared the combined genotypes of MTR 2756A>G and MTHFR 677C > T polymorphisms between the control and ischemic stroke groups (Table 2). The frequencies of the combined genotypes were not significantly different in comparisons between the cases and controls. The odds ratio of the combined MTR 2756AG/MTHFR 677CC genotype and with the combined MTR 2756AA/MTHFR 677CC genotype was 1.20 (95% CI, 0.54-2.69; p = 0.69). The odds ratio of MTR 2756AA/MTHFR677CT and MTR 2756AG/MTHFR 677CT combined genotypes was 0.85 (95% CI, 0.53-1.36; p = 0.55) and 0.71 (95% CI, 0.36-1.39; p = 0.39), respectively.
We examined whether the 2756A > G polymorphism of the MTR gene was associated with plasma homocysteine concentration in the control and ischemic stroke groups (Table 3). The 2756AA genotype of the ischemic stroke group was associated with a higher homocysteine concentration compared to the control group (p = 0.04). The folate concentration did not show significant differences among genotypes of the MTR 2756A > G polymorphism (data not shown).
In contrast, the MTHFR 677C > T polymorphism had no significant differences in genotype frequencies between cases and controls (Table 2). The frequency of the mutant 677T allele in cases and controls was 0.42 and 0.44, respectively. The 677TT genotype in the patient group was associated with a significantly higher homocysteine concentration than that of the control group (p = 0.01). The 677CT genotype in cases showed higher homocysteine concentrations than that of the controls, although this finding was not significant (p = 0.06). For the homocysteine concentration of the combined MTR 2756AA/MTHFR 677TT genotype, the patient group was significantly higher than the control group (p = 0.04; Table 3). However, the folate concentration did not show a significant difference among combined genotypes of MTR 2756A > G/MTHFR 677C > T polymorphisms (data not shown).
When the top and bottom 20% of measured homocysteine levels in patients with ischemic stroke were compared to each other, the frequency of the MTHFR 677TT genotype was significant, although there was no significant difference in the MTR 2756A > G polymorphism (Table 4). Interestingly, the combined genotype frequency of MTR 2756AA and MTHFR 677TT was significantly different between the top 20% and the bottom 20% of measured homocysteine levels (p = 0.03).
A number of epidemiological studies have shown that in addition to environmental factors, genetic mechanisms may play a role in determining susceptibility to vascular disease. In particular, abnormalities in the enzymes that control homocysteine metabolism have been shown to cause vascular disease. MTHFR is the main regulatory enzyme for homocysteine metabolism. Our previous reports have suggested that the homozygous 677C > T mutation in the MTHFR gene is associated with ischemic stroke.9,10 MTR is also involved in homocysteine metabolism. Thus the potential role of MTR and MTHFR genotypes in the regulation of homocysteine metabolism has attracted considerable interest.
We therefore studied the relationship between genetic variations of the MTR and MTHFR genes and ischemic stroke in Korean subjects with a case-control study. Because the genotype distributions of MTR 2756A > G and MTHFR 677C > T in ischemic stroke were not significantly different in our data (Table 2), we compared the top 20% and bottom 20% of Hcy levels. The MTR polymorphism in the patient group had no significant difference between the top 20% and bottom 20% (Table 4). However, the MTHFR 677TT genotype was associated with risk for hyperhomocysteinemia. The MTHFR 677TT, inpatients with ischemic stroke, had a higher ratio, by 5.8 fold, compared to the top of 20% and bottom of 20% of tHcy (OR = 5.82; Table 4). When compared to MTR 2756AA/MTHFR 677CC, the 2756AA/677TT genotype was only significantly different when associated with an elevated homocysteine level (OR = 4.57, Table 4). These results support that MTHFR 677TT and the combined MTR 2756AA/MTHFR 677TT genotypes are risk factors for hyperhomocysteinemia in patients with ischemic stroke.
The G allele frequency of the MTR 2756A > G polymorphism was rare (0.11) in the healthy Korean population. This is in contrast to findings from previous studies where the rare allele frequencies were shown to be 0.15-0.21 in healthy Caucasians (Table 5). Among other Asian populations studied, the G allele frequency of the MTR polymorphism in the Chinese population was similar to this study's data.33 The Japanese population, however, had a higher G allele frequency than the Korean or Chinese populations.26,36,37 This finding is difficult to explain on the basis of differences in genetic, environmental, or other factors among populations.
One possible explanation could be differences in genetic drift or selection against a particular allele in the comparisons between Korean and Caucasian populations. In addition, the differences in study results may be caused by the differences in sample size or bias resulting from sample selection of the populations studied.12,38 Another possibility is that it may be, at least in part, due to the differences in ischemic stroke prevalence and homocysteine concentrations among Korean, Caucasian, and Japanese populations. To compare the allele frequency of the MTR polymorphism among different populations, further studies including different racial and ethnic groups are needed.
In this study, homocysteine concentrations in cases with the MTR 2756AA genotype were significantly higher than those of the control group (Table 3). There was also a significantly higher homocysteine concentration in the patient group versus the control group for the combined MTR 2756AA/MTHFR 677TT genotype. Multiple logistic regression analysis for homocysteine level was carried out by using selection strategy with a continuous variable (age) and categorical variables (gender, smoking, hypertension, diabetes mellitus, hyperlipidemia, MTHFR genotype, MTR genotype). The most important independent risk factor for hyperhomocysteinemia in ischemic stroke were age (p = 0.000), MTHFR 677TT (p = 0.001) and gender (p = 0.026). MTR genotype, hypertension, and diabetes mellitus were not significant predictors for hyperhomocysteinemia in ischemic stroke patients (p > 0.05).
To date, many MTR 2756A > G polymorphism studies have been performed in a variety of populations. Among these populations, the MTR 2756A > G polymorphism has been associated with clinical symptoms in some populations, although the results have not always been confirmed in all populations. For example, it has been reported that the MTR 2756GG genotype is associated with a protective effect against the development of CHD in three populations,3,31,33 while the 2756GG genotype has an adverse effect on the CHD development in the Dutch population.14 In addition, the MTR polymorphism was associated with a lower risk of colorectal cancer among individuals with low alcohol consumption.28 The combined genotypes for the MTR/MTRR polymorphisms were associated with an elevated risk for NTD in Americans29 and thrombotic events in the British population.30
In contrast, some case-control studies have reported no association between the MTR polymorphism and ischemic stroke, CHD, or NTD. For example, Zhang and Dai33 reported that there was no association between the MTR polymorphism and ischemic stroke or CHD risk in a Chinese population study. Similar trends were seen in Australian and Japanese populations. There was no association between the MTR polymorphism and CHD in an Australian population32 and NTD susceptibility in Italian31 and British2 populations. Therefore, association studies of the MTR polymorphism have shown conflicting results among the populations studied. Therefore, to date, the results of MTR polymorphism studies have not been race or ethnic-specific.
In conclusion, in the present study, high homocysteine concentration in patients who had ischemic stroke appeared to be associated with the MTHFR 677TT genotype or combined MTR 2756AA and MTHFR 677TT genotype. However, in light of the differences of the association of the MTR 2756A > G polymorphism and clinical symptoms among human populations, further work is needed to examine the effects of mutations in the MTR gene. Therefore, the findings of the MTR polymorphism and association with disease, obtained in a variety of populations, may not yet be conclusive. In addition, the precise biochemical mechanisms of the MTR and MTHFR alleles that affect homocysteine concentration require further research in order to determine the associations between the MTR and MTHFR polymorphisms and homocysteine concentration.
Figures and Tables
Table 3
Plasma Homocysteine Concentrations of Ischemic Stroke Patients and Controls according to MTR 2756A > G and MTHFR 677C > T Genotypes
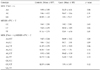
References
1. Harmon DL, Shields DC, Woodside JV, McMaster D, Yarnell JW, Young IS, et al. Methionine synthase D919G polymorphism is a significant but modest determinant of circulating homocysteine concentrations. Genet Epidemiol. 1999. 17:298–309.
2. Lucock M, Daskalakis I, Briggs D, Yates Z, Levene M. Altered folate metabolism and disposition in mothers affected by a spina bifida pregnancy: influence of 677c → t methylenetetrahydrofolate reductase and 2756a → g methionine synthase genotypes. Mol Genet Metab. 2000. 70:27–44.
3. Castro R, Rivera I, Ravasco P, Jakobs C, Blom HJ, Camilo ME, et al. 5,10-Methylenetetrahydrofolate reductase 677C→T and 1298A→C mutations are genetic determinants of elevated homocysteine. QJM. 2003. 96:297–303.
4. Chango A, Boisson F, Barbe F, Quilliot D, Droesch S, Pfister M, et al. The effect of 677C→T and 1298A→C mutations on plasma homocysteine and 5,10-methylenetetrahydrofolate reductase activity in healthy subjects. Br J Nutr. 2000. 83:593–596.
5. Friedman G, Goldschmidt N, Friedlander Y, Ben-Yehuda A, Selhub J, Babaey S, et al. A common mutation A1298C in human methylenetetrahydrofolate reductase gene: association with plasma total homocysteine and folate concentrations. J Nutr. 1999. 129:1656–1661.
6. Frosst P, Blom HJ, Milos R, Goyette P, Sheppard CA, Matthews RG, et al. A candidate genetic risk factor for vascular disease: a common mutation in methylenetetrahydrofolate reductase. Nat Genet. 1995. 10:111–113.
7. Perini F, Galloni E, Bolgan I, Bader G, Ruffini R, Arzenton E, et al. Elevated plasma homocysteine in acute stroke was not associated with severity and outcome: stronger association with small artery disease. Neurol Sci. 2005. 26:310–318.
8. Howard VJ, Sides EG, Newman GC, Cohen SN, Howard G, Malinow MR, et al. Changes in plasma homocyst(e)ine in the acute phase after stroke. Stroke. 2002. 33:473–478.
9. Kim NK, Choi BO, Jung WS, Choi YJ, Choi KG. Hyperhomocysteinemia as an independent risk factor for silent brain infarction. Neurology. 2003. 61:1595–1599.
10. Choi BO, Kim NK, Kim SH, Kang MS, Lee S, Ahn JY, et al. Homozygous C677T mutation in the MTHFR gene as an independent risk factor for multiple small-artery occlusions. Thromb Res. 2003. 111:39–44.
11. Girelli D, Martinelli N, Pizzolo F, Friso S, Olivieri O, Stranieri C, et al. The interaction between MTHFR 677 C→T genotype and folate status is a determinant of coronary atherosclerosis risk. J Nutr. 2003. 133:1281–1285.
12. Lievers KJ, Boers GH, Verhoef P, den Heijer M, Kluijtmans LA, van der Put NM, et al. A second common variant in the methylenetetrahydrofolate reductase (MTHFR) gene and its relationship to MTHFR enzyme activity, homocysteine, and cardiovascular disease risk. J Mol Med. 2001. 79:522–528.
13. Ma J, Stampfer MJ, Hennekens CH, Frosst P, Selhub J, Horsford J, et al. Methylenetetrahydrofolate reductase polymorphism, plasma folate, homocysteine, and risk of myocardial infarction in US physicians. Circulation. 1996. 94:2410–2416.
14. Spence JD, Malinow MR, Barnett PA, Marian AJ, Freeman D, Hegele RA. Plasma homocysteine concentration, but not MTHFR genotype, is associated with variation in carotid plaque area. Stroke. 1999. 30:969–973.
15. Zintzaras E. Association of methylenetetrahydrofolate reductase (MTHFR) polymorphism with genetic susceptibility to gastric cancer: a meta-analysis. J Hum Genet. 2006. 51:618–624.
16. Hur M, Park JY, Cho HC, Lee KM, Shin HY, Choi HI. Methylenetetrahydrofolate reductase A1298C genotypes are associated with the risks of acute lymphoblastic leukaemia and chronic myelogenous leukaemia in the Korean population. Clin Lab Haematel. 2006. 28:154–159.
17. Song KS, Song JW, Choi JR, Kim HK, Shin JS, Kim JH. Homozygous V/V (677C to T) and D/D (2756 G to A) variants in the methylenetetrahydrofolate and methionine synthase genes in a case of hyperhomocysteinemia with stroke at young age. Exp Mol Med. 2001. 33:106–109.
18. Kim NK, Chong SY, Jang MJ, Hong SH, Kim HS, Cho EK, et al. Association of the methylenetetrahydrofolate reductase polymorphism in Korean patients with childhood acute lymphoblastic leukemia. Anticancer Res. 2006. 26:2879–2881.
19. Kim JK, Kim S, Han JH, Kim HJ, Chong SY, Hong SP, et al. Polymorphisms of 5,10-methylenetetrahydrofolate reductase and risk of stomach cancer in a Korean population. Anticancer Res. 2005. 25:2249–2252.
20. Kim NK, Han JH, Oh D, Hwang SG, Chae KY, Kim HJ, et al. Methylenetetrahydrofolate reductase gene polymorphisms in patients with Down syndrome. Korean J Genet. 2004. 26:431–436.
21. Kim NK, Choi YK, Kang MS, Choi DH, Cha SH, An MO, et al. Influence of combined methylenetetrahydrofolate reductase (MTHFR) and thymidylate synthase enhancer region (TSER) polymorphisms to plasma homocysteine levels in Korean patients with recurrent spontaneous abortion. Thromb Res. 2006. 117:653–658.
22. Cronin S, Furie KL, Kelly PJ. Dose-related association of MTHFR 677T allele with risk of ischemic stroke: evidence from a cumulative meta-analysis. Stroke. 2005. 36:1581–1587.
23. Leclerc D, Campeau E, Goyette P, Adjalla CE, Christensen B, Ross M, et al. Human methionine synthase: cDNA cloning and identification of mutations in patients of the cblG complementation group of folate/cobalamin disorders. Hum Mol Genet. 1996. 4:1867–1874.
24. Klerk M, Lievers KJ, Kluijtmans LA, Blom HJ, den Heijer M, Schouten EG, et al. The 2756A > G variant in the gene encoding methionine synthase: its relation with plasma homocysteine levels and risk of coronary heart disease in a Dutch case-control study. Thromb Res. 2003. 110:87–91.
25. Chen J, Stampfer MJ, Ma J, Selhub J, Malinow MR, Hennekens CH, et al. Influence of a methionine synthase (D919C) polymorphism on plasma homocysteine and folate levels and relation to risk of myocardial infarction. Atherosclerosis. 2001. 154:667–672.
26. Morita H, Kurihara H, Sugiyama T, Hamada C, Kurihara Y, Shindo T, et al. Polymorphism of the methionine synthase gene: association with homocysteine metabolism and late-onset vascular diseases in the Japanese population. Arterioscler Thromb Vasc Biol. 1999. 19:298–302.
27. Tsai MY, Welge BG, Hanson NQ, Bignell MK, Vessey J, Schwichtenberg K, et al. Genetic causes of mild hyperhomocysteinemia in patients with premature occlusive coronary artery diseases. Atherosclerosis. 1999. 143:163–170.
28. Ma J, Stampfer MJ, Christensen B, Giovannucci E, Hunter DJ, Chen J, et al. A polymorphism of the methionine synthase gene: Association with plasma folate, vitamin B12, homocyst(e)ine, and colorectal cancer risk. Cancer Epidemiol Biomarkers Prev. 1999. 8:825–829.
29. Zhu H, Wicker NJ, Shaw GM, Lammer EJ, Hendricks K, Suarez L, et al. Homocysteine remethylation enzyme polymorphisms and increased risks for neural tube defects. Mol Genet Metab. 2003. 78:216–221.
30. Yates Z, Lucock M. Interaction between common folate polymorphism and B-vitamin nutritional status modulates homocysteine and risk for a thrombotic event. Mol Genet Metab. 2003. 79:201–213.
31. De Marco P, Calevo MG, Moroni A, Arata L, Merello E, Finnell RH, et al. Study of MTHFR and MS polymorphisms as risk factors for NTD in the Italian population. J Hum Genet. 2002. 47:319–324.
32. Wang XL, Duarte N, Cai H, Adachi T, Sim AS, Cranney G, et al. Relationship between total plasma homocysteine, polymorphisms of homocysteine metabolism related enzymes, risk factors and coronary artery disease in the Australian hospital-based population. Atherosclerosis. 1999. 146:133–140.
33. Zhang G, Dai C. Gene polymorphisms of homocysteine metabolism-related enzymes in Chinese patients with occlusive coronary artery or cerebral vascular diseases. Thromb Res. 2001. 104:187–195.
34. Lahiri DK, Nurnberger JI Jr. A rapid non-enzymatic method for the preparation of HMW DNA from blood for RFLP studies. Nucleic Acids Res. 1991. 19:5444.
35. te Poele-Pothoff MT, van den Berg M, Franken DG, Boers GH, Jakobs C, de-Kroon IF, et al. Three different methods for the determination of total homocysteine in plasma. Ann Clin Biochem. 1995. 32:218–220.
36. Le Marchand L, Donlon T, Hankin JH, Kolonel LN, Wilkens LR, Seifried A. B-vitamin intake, metabolic genes, and colorectal cancer risk (United States). Cancer Causes Control. 2002. 13:239–248.
37. Matsuo K, Suzuki R, Hamajima N, Ogura M, Kagami Y, Taji H, et al. Association between polymorphisms of folate- and methionine-metabolizing enzymes and susceptibility to malignant lymphoma. Blood. 2001. 97:3205–3209.
38. Goode EL, Potter JD, Bigler J, Ulrich CM. Methionine synthase D919G polymorphism, folate metabolism, and colorectal adenoma risk. Cancer Epidemiol Biomarkers Prev. 2004. 13:157–162.