Abstract
This study was performed to assess the influence of the cryoinjury on the dynamics of wavefronts and to determine whether they can convert ventricular fibrillation (VF) to ventricular tachycardia (VT) in fibrillating right ventricular (RV) of swines using an optical mapping system. A cryoinjury with a diameter of 12 mm was created on the epicardium of perfused RV of swines (n = 6) and optical mapping were taken from baseline until 10 minutes after the cryoinjury. Out of 35 cryoinjuries, the images were possible to be interpreted in 32. The optical action potential could not be observed in either the cryoinjury or peri-injury sites at 1 and 3 minutes, was observed in only the cryoinjury site at 5 minutes, and recovered in both sites at 10 minutes. The cycle length of the tachycardia was 135.9 ± 23.6 msec at baseline, 176.2 ± 79.3 msec at 1 minute, 187.6 ± 97.9 msec at 3 minutes, 185.5 ± 19.2 msec at 5 minutes, and 152.1 ± 64.1 msec at 10 minutes. The cycle lengths at 1, 3, and 5 minutes after the cryoinjury were significantly more prolonged than that at baseline (p = 0.001, p = 0.006, p = 0.016). After the cryoinjury, the VF changed to VT in 9 (28.0%), and terminated in 2 (6.3%). These changes were observed mainly within 5 minutes after cryoinjury. The cryoinjury had anti-fibrillatory effects on the tissue with VF. This phenomenon was related to a decreasing mass and stabilizing wavefronts.
Coronary heart disease and its consequences account for at least 80 percent of sudden cardiac deaths in Western cultures.1 In myocardial infarction, an infarction region without any electrical activity and a border zone with slow conduction are produced, and serve as heterogeneous obstacles.2,3 Ventricular arrhythmias which produced by these obstacles are the main causes of sudden cardiac deaths. Therefore, various types of obstacles have been used extensively to study the mechanism of arrhythmias.4-7 Artificial obstacles may convert a meandering reentrant wavefront into a stable one in experimental and simulated two-dimensional tissue.6,7 However, the previous studies used homogenous obstacles and were insufficient in explaining the arrhythmias related to infarctions which serve as heterogeneous obstacles. Further, they used electrical mapping systems for the mapping. However, electrical mapping systems can not obtain information on the repolarization. Recently developed optical mapping systems can obtain data on both the depolarization and repolarization with a higher resolution.
The purpose of the present study was 1) to assess the influence of cryoinjury on the dynamics of multiple-wavelets during ventricular fibrillation (VF); 2) to determine whether an artificial obstacle can convert VF to ventricular tachycardia (VT) by stabilizing the reentrant wavefront; and 3) to determine the effects of an artificial obstacle on the critical mass for VF with an optical mapping system.
The study protocol was approved by the Institutional Animal Care and Use Committee. The experimental model has been previously described.8-10 Briefly, the chest was opened, and the heart was quickly removed. The right coronary artery was cannulated, and the right ventricle (RV) was isolated and continuously perfused with oxygenated Tyrode solution (37℃, pH 7.4).
The composition of the Tyrode solution was as follows (in mM): 125.0 of NaCl, 4.5 of KCl, 0.5 of MgCl2, 0.54 of CaCl2, 1.2 of NaH2PO4, 24.0 of NaHCO3, and 5.5 of glucose, and included 50 mg/L of albumin. The RVs were stained for 20 min with di-4-ANEPPS (20 min; 1 mmol/L) through coronary perfusion. After the dye staining was completed, the patterns of the activation were mapped with optical mapping technique.
The optical mapping system was similar to the ones described previously.8-10 The quasi-monochromatic light (500 ± 30 nm) from two green LED lamps (LL-50R30-G25, Optronix, Korea) was used as the light source. Fluorescent and scattered light was collected through a 600-nm long-pass glass filter (R60, Nikon; Tokyo, Japan) with a 12-bit digital charge-coupled device camera (CA-D1-0256T, Dalsa; Ontario, Canada). The camera acquired data at 3.75-ms intervals from 96 ± 96 sites simultaneously over a 35 ± 35-mm2 area, resulting in a spatial resolution of 0.36 mm per pixel. The digital images were transferred to a personal computer with a frame grabber (IC-PCI-DIG16, Imaging Technology; Bedford, MA). Because all tissues had ventricular fibrillation at the beginning of optical mapping, there were little movement of tissues. To prevent the additional motion artifact, the margin of tissue was fixed with 4-5 pins to the base of the tissue chamber.
The fluorescence was converted to a pseudo-color animation for analysis. The maximal signal amplitude was coded yellow, representing a fully depolarized state. The minimum signal amplitude was coded black, representing a fully repolarized state. Each depolarizing pixel was then assigned a color out of 128 colors among black, red, and yellow and a repolarizing pixel was assigned a color out of 128 colors among yellow, green, and black. We defined the number of wavelets at a given instant of VF/VT as the number of activations that were independently excited over at least two frames in different areas of the mapped tissue that had different directions of propagation and were clearly separated from each other by recovered but nonactivated tissue. VF is a highly irregular and disordered rhythm. However, there might be different degrees of disorder among different episodes of VF.
A cyoinjury lesion was then created by attaching a 12-mm-diameter cryoinjury rod (homemade) for 30 seconds. The cryoinjury rod had been frozen with liquid nitrogen before creating the cryoinjury. The specific location of the cryoinjury within the tissue was determined by the need to avoid major epicardial arteries, but in general the lesion was created near the center of the tissue. Several recordings were acquired before and 1 min, 3 min, 5 min, and 10 min after the creation of the obstacle. After the electrical activity of tissue was fully recovered, the cryoinjury was repeated at the previous injury site.
The experiments were performed in 6 female swines with weight of 35 ± 3 kg, and optimal images were collected in all animals. Ventricular fibrillation was induced spontaneously without stimulation in all.
In six RVs with ventricular fibrillation, a total of 35 cyroinjuries, consisting of 3 to 11 cryoinjuries per RV tissue preparation, were made and it was possible to interpret the images from those 32 cryoinjuries (Table 1). No optical action potential was observed in either the cryoinjury or peri-injury sites at 1 minute. Although there were time differences in the electrical recovery of the tissue, the optical action potential was partially recovered in the peri-injury site at 3 and 5 minutes, and recovered in both the cryoinjury and peri-injury sites at 10 minutes (Fig. 1). Block of the electrical conduction was observed in the peri-injury and injury sites and the ventricular fibrillation was converted to ventricular tachycardia by the cryoinjury.
The electrical conduction of the tissue improved after the perfusion, and variable degrees of block and attachment were observed (Fig. 2). The reentry wavefronts were also observed with the longest reentry being 10 beats (Fig. 3). The electrical activity of the center of the reentry circuit also was decreased, and this finding was similar to that of normal tissue (data is not presented).
The cycle length of the tachycardia was 135.9 ± 23.6 msec at baseline, 176.2 ± 79.3 msec at 1 minute, 187.6 ± 97.9 msec at 3 minutes, 185.5 ± 19.2 msec at 5 minutes, and 152.1 ± 64.1 msec at 10 minutes. The cycle length at 1, 3, and 5 minutes after the cryoinjury was significantly more prolonged than that at baseline (p = 0.001, p = 0.006, p = 0.016) (Table 1, Fig. 4).
From the optical mapping recordings after creating the 35 cryoinjuries, the second and third optical maps from the 5th swine were not possible to be analyzed because of a data storage problem, and neither was the 1st optical map from the 1st swine because of the poor image quality. Out of the 32 optical maps, ventricular fibrillation persisted in 21 (65.6%) cryoinjuries (Table 1). However, no optical signals were observed at the cyroinjury sites in those cases, and block had occurred due to the cryoinjury.
Ventricular fibrillation converted to tachycardia in 9 (28.0%), and terminated in 2 (6.3%). Those conversions were observed mainly within 5 minutes after the cryoinjury (Table 1). In 2 cryoinjuries with termination of VF, idioventricular rhythm and ventricular fibrillation simultaneously occurred before the termination of the VF (Fig. 5A, B). The ventricular fibrillation was abolished by additional cryoinjuries (Fig. 5C, D).
There are several mechanisms for explaining ventricular fibrillation. Moe et al.11 hypothesized that the constant formation of new wavelets occurs through the process of wave splitting (wavebreak), resulting in multiple wavelet fibrillation. A competing hypothesis of VF is the Focal Source Hypothesis. This hypothesis proposes that a single, rapidly firing focal source (reentrant or automatic) is the fundamental driver of VF, and that the multiple wavelets that characterize VF are an epiphenomena caused by "fibrillatory conduction block" because impulses from the rapid focus are unable to conduct 1 : 1 into the surrounding tissue. Gray et al.12 first demonstrated that a single meandering spiral wave could underlie polymorphic VT resembling VF. Recently, Wu et al.13 suggested that 2 types of VF resulting from different mechanisms could coexist in the same heart under different conditions. Chen et al.14 suggested that type I VF is consistent with VF resulting from a multiple wavelet mechanism. On the other hand, type II VF is characterized by large and repeatable patterns of epicardial activations with occasional wavebreaks suggesting that an ongoing focal and relatively stable source is the engine of VF. If the different investigators have been studying different types of VF, this could account for their drastically different interpretations. However, the genesis and maintenance of fibrillation has not yet been completely revealed.
This study evaluated the influence of heterogeneous obstacles created by cryoinjury using an optical mapping system. Davidenko et al.15 and Pertsov et al.16 reported that naturally occurring obstacles, like arteries and other anatomical inhomogeneities, can attach a drifting spiral wave and make it stationary. Valderrábano et al.17 reported that a full-thickness artificial anatomical obstacle induces slowing and regularization of fibrillation, impairs the persistence of fibrillation as judged by an increase in the critical mass, and can convert VF to VT by serving as an attachment site to reentrant excitation, in a perfused swine right ventricular model with an electrical mapping system. We also previously reported similar results in isolated, perfused swine right ventricle (RV) with an optical mapping system, which had the benefit of a higher resolution and obtaining additional repolarization data as compared to electrical mapping systems.8 However, all previous studies used a homogeneous obstacle model. The heterogeneous obstacles created by cryoinjury in this study exhibited an injury site without any electrical activity and a peri-injury site with partial electrical activity that also exhibited conduction block, delay, and attachment. Therefore this obstacle model is closer to the clinical infarction model, and it will help investigate the influence of myocardial infarctions on ventricular fibrillation.
The conversion of VF to VT could result from the reduction in the mass of the myocardium or stablilization of the wavefronts around the obstacles. To demonstrate the stabilization of the wavefronts, stable reentry has to exist around the obstacles. Although more reentrant wavefronts were observed with larger obstacles, stable reentry was not observed in the previous studies.6,8,17 We previously suggested that a homogeneous obstacle model using a transmural punch biopsy, limited the survival of the tissue and could not make bigger obstacles because of the impairment of the circulation.8 The cryoinjury model used in this study could avoid this problem.
The size of the cryoinjury was 12 mm in this study. When we consider the peri-injury site, the size of the obstacle will be bigger than expected. However, we could not observe any stable reentry around the injury site. Moreover, the analysis of 9 conversions of VF to VT did not show any stable reentry around the obstacle. The interesting finding is that VTs were observed within 5 minutes of the cryoinjury. This suggests that the effect of the decreasing mass of the myocardium was important in the conversion of VF to VT. In particular, in the 5th swine, the coexistence of idioventricular rhythm and VF after the 5th cryoinjury was observed. The VF transiently disappeared with additional cryoinjuries and reappeared in the recordings performed at 10 minutes. This finding showed the close relationship between the mass and maintenance of VF. However, the wavefront stablilization might also be present due to the presence of a large obstacle. It is assumed that the time-dependent changes of VF cycle length could be due to the gradual recovery of some tissues from cryo lesions. The shrinking sizes of the lesion could gradually lose its effects of wavefronts anchoring, resulting gradually shortening cycle length toward baseline.
In conclusion, the important findings of this study were; i) cryoinjury can be used as an obstacle model in pig RV with ventricular fibrillation, ii) those obstacles can induce block, attachment, and reentry of wavefronts, and can prolong the cycle length of the optical signal, iii) those obstacles can convert VF to VT by stabilizing the RWF, and terminate the VF. The cryoinjury had anti-fibrillatory effects in the tissue with ventricular fibrillation. This phenomenon was related to decreasing mass and stabilizing wavefronts.
Kim et al.18 reported that reentry around papillary muscles was observed after a decrease in the myocardial mass in a swine RV perfusion model. Pak et al.19 also reported that reentry around papillary muscles was observed after beta blockers and that VT could be terminated by cyroinjury around the papillary muscle. Because we did not map from the endocardial side, we could not show the effect of papillary muscles on the reentry.
This study could not show the exact depth of lesion created by the cryoinjury. We performed optical mapping only on the epicardial side. Because we planned to evaluate the acute change of wavefronts by the cryoinjury, we analyzed optical mapping within 10 minutes of the cryoinjury. Therefore, the histological analysis could not show the exact extent of the cryoinjury.
Figures and Tables
Fig. 1
Optical fluorescent signal arranged by time sequences after the cryoinjury in pig No.5. (A. Raw image) The previous ventricular fibrillation (B. Baseline) was terminated and changed to ventricular tachycardia (C, D, E, F). The cycle length of tachycardia was increased after the cryoinjury and gradually reduced as time went on. The fluorescence signals of the periinjury zone (③) and cryoinjury site (④) were recovered after 5minutes (D) and 10 minutes (E) of the cryoinjury, respectively.
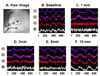
Fig. 2
Intermittent conduction block and attachment of wavefronts. (A) Consecutive optical map snapshots from Pig No. 2 after 3 minutes of the cryoinjury (circle). Wavefronts were initiated from the right upper region (①) and then propagated to the left lower region (④). Some waves were blocked (*) and attached at the cyoinjury site(†). (B) The local voltage signals (in fluorescence) at selected locations (circled numbers). Some signals were blocked. The signals that conducted were delayed at the peri- injury (②) or injury sites (③).
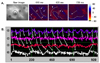
Fig. 3
A clockwise rotation of a wavefront after cryoinjury. (A) Consecutive optical map snapshots from pig No. 5 after 5 minutes of the cryoinjury (circle). A wavefront initated from the left upper region, then rotated clockwise for 10 beats. (B) The local voltage signals (in fluorescence) at selected locations (circled numbers). The signals were decreased at the cyoinjury site (②) as compared to the with reentry site (①). The shaded area represents the period during simultaneous optical mapping.
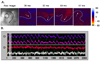
Fig. 4
Change in the cycle length according to the time after the cryoinjury (paired t-test with the Bonferroni correction). *p = 0.001 : change in the cycle length between that at baseline and 1 minute after cryoinjury. †p = 0.006. change in the cycle length between that at baseline and 3 minutes after cryoinjury. ‡p = 0.016 : change in the cycle length between that at baseline and 5 minutes after cryoinjury.
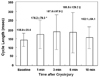
Fig. 5
Coexistance of idioventricular rhythm and ventricular fibrillation (A and B). Termination of ventricular fibrillation (C and D). A. C. Optical mapping of Pig No. 5 after 15 minutes of 4th cryoinjury (circle) showed wavefronts of idioventricular rhythm (white arrow) and ventricular fibrillation (yellow arrow). Optical fluorescent signal showed idioventricular rhythm (①) and ventricular fibrillation (②). Signal was not observed at the cryoinjury site (③). B. D. Optical mapping of pig No. 5 after 5 minutes of the 5th cryoinjury (circle). Idioventricular rhythm (white arrow) previously observed persisted. However, ventricular fibrillation was terminated. Optical florescent signal showed idioventricular rhythm (①). However, signal was not observed at previous ventricular fibrillation (②) and the cryoinjury site (③). Circular numbers represents sites where optical signals were taken in the raw image of upper panel. Shaded area represent the period of the simultaneous optical mapping.
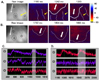
References
1. Myerburg RJ, Interian A Jr, Mitrani RM, Kessler KM, Castellanos A. Frequency of sudden cardiac death and profiles of risk. Am J Cardiol. 1997. 80:10F–19F.
2. De Bakker JM, Janse MJ. Zipes DP, Jalife J, editors. Pathophysiological correlates of ventricular tachycardia in hearts with a healed infarct. Cardiac Electrophysiology: From Cell to Bedside. 2000. 3rd ed. Philadelphia: WB Saunders;415–421.
3. Zheng ZJ, Croft JB, Giles WH, Mensah GA. Sudden cardiac death in the United States, 1989 to 1998. Circulation. 2001. 104:2158–2163.
4. Brugada J, Boersma L, Kirchhof CJ, Heynen VV, Allessie MA. Reentrant excitation around a fixed obstacle in uniform anisotropic ventricular myocardium. Circulation. 1991. 84:1296–1306.
5. Bernstein RC, Frame LH. Ventricular reentry around a fixed barrier. Resetting with advancement in an in vitro model. Circulation. 1990. 81:267–280.
6. Ikeda T, Yashima M, Uchida T, Hough D, Fishbein MC, Mandel WJ, et al. Attachment of meandering reentrant wave fronts to anatomic obstacles in the atrium. Role of the obstacle size. Circ Res. 1997. 81:753–764.
7. Girouard SD, Pastore JM, Laurita KR, Gregory KW, Rosenbaum DS. Optical mapping in a new guinea pig model of ventricular tachycardia reveals mechanisms for multiple wavelengths in a single reentrant circuit. Circulation. 1996. 93:603–613.
8. Joung BY, Koo BK, Xu ZZ, Kim IK, Lee MH, Kim SS. The effects of obstacles on the dynamics of ventricular fibrillation. Korean Circ J. 2005. 35:183–191.
9. Lee MH, Qu Z, Fishbein GA, Lamp ST, Chang EH, Ohara T, et al. Patterns of wave break during ventricular fibrillation in isolated swine right ventricle. Am J Physiol Heart Circ Physiol. 2001. 281:H253–H265.
10. Lee MH, Lin SF, Ohara T, Omichi C, Okuyama Y, Chudin E, et al. Effects of diacetyl monoxime and cytochalasin D on ventricular fibrillation in swine right ventricles. Am J Physiol Heart Circ Physiol. 2001. 280:H2689–H2696.
11. Moe GK. On the multiple wavelet hypothesis of atrial fibrillation. Arch Int Pharmacodyn Ther. 1962. 140:183–188.
12. Gray RA, Jalife J, Panfilov AV, Baxter WT, Cabo C, Davidenko JM, et al. Mechanisms of cardiac fibrillation. Science. 1995. 270:1222–1223. author reply 1224-5.
13. Wu TJ, Lin SF, Weiss JN, Ting CT, Chen PS. Two types of ventricular fibrillation in isolated rabbit hearts: importance of excitability and action potential duration restitution. Circulation. 2002. 106:1859–1866.
14. Chen PS, Wu TJ, Ting CT, Karagueuzian HS, Garfinkel A, Lin SF, et al. A tale of two fibrillations. Circulation. 2003. 108:2298–2303.
15. Davidenko JM, Pertsov AV, Salomonsz R, Baxter W, Jalife J. Stationary and drifting spiral waves of excitation in isolated cardiac muscle. Nature. 1992. 355:349–351.
16. Pertsov AM, Davidenko JM, Salomonsz R, Baxter WT, Jalife J. Spiral waves of excitation underlie reentrant activity in isolated cardiac muscle. Circ Res. 1993. 72:631–650.
17. Valderrabano M, Kim YH, Yashima M, Wu TJ, Karagueuzian HS, Chen PS. Obstacle-induced transition from ventricular fibrillation to tachycardia in isolated swine right ventricles: insights into the transition dynamics and implications for the critical mass. J Am Coll Cardiol. 2000. 36:2000–2008.
18. Kim YH, Xie F, Yashima M, Wu TJ, Valderrabano M, Lee MH, et al. Role of papillary muscle in the generation and maintenance of reentry during ventricular tachycardia and fibrillation in isolated swine right ventricle. Circulation. 1999. 100:1450–1459.
19. Pak HN, Oh YS, Liu YB, Wu TJ, Karagueuzian HS, Lin SF, et al. Catheter ablation of ventricular fibrillation in rabbit ventricles treated with beta-blockers. Circulation. 2003. 108:3149–3156.