Abstract
The brain is particularly vulnerable to oxygen free radicals, and these radicals have been implicated in the pathology of several neurological disorders. In this study, the modulation of TNF-related apoptosis-inducing ligand (TRAIL) expression by oxidative stress was shown in LN215 cells, an astroglioma cell line. Hydrogen peroxide (H2O2) treatment increased TRAIL expression in LN215 cells and H2O2-induced TRAIL augmented apoptosis in Peer cells, a cell line sensitive to TRAIL-mediated cell death. Our findings suggest that the upregulation of TRAIL in astroglial cells may abrogate immune cell effector functions.
TNF-related apoptosis-inducing ligand (TRAIL), a member of death ligand/receptor pairs, acts independently of Fas-mediated apoptosis. Like FasL, TRAIL induces rapid apoptosis in transformed cell lines of diverse origins.1,2 Death receptors (DR) 4 and DR5, which are receptors for TRAIL, mediate apoptosis by recruiting Fas-associated death domain (FADD) indirectly via the TNF receptor-associated death domain (TRADD) and by the subsequent activation of capsase 8.3,4 TRAIL and DR4 are expressed in many human tissues, including the spleen, lung, and prostate.5 The human TRAIL promoter was cloned, and a number of transcription factor binding sites were identified, namely, the NFAT, AP-1, and Sp1 sequences, which are important for the expression of other TNF family members.6 DR4 and DR5 were expressed in normal brain tissue and glioma.7 Although TRAIL expression is detected in many human tissues, TRAIL is not always cytotoxic due to the presence of decoy receptors. The decoy receptors, DcR1 and DcR2, have also been found in many types of normal cells. whereas many tumor cell lines do not express DcR1,2,5 which abrogates apoptotic signals by binding TRAIL, because DcR1 does not have death domains. DcR2 has a truncated form of cytoplasmic tail, which cannot transduce cell death signals.8
The relationship between reactive oxygen intermediate (ROIs) products and central nervous system (CNS) tumors is a current and important topic in light of their involvement in pathological conditions and possible therapeutic interventions. Hydrogen peroxide (H2O2) has been implicated in cellular and tissue injury during pathologic conditions, such as ischemia-reperfusion injury, hyperoxia, and inflammation.9 In addition, the stimulation of various cells with either cytokines or phorbol ester increases the secretion of H2O2 into the extracellular space in vitro.10,11 High concentrations of this diffusible ROI exert toxic effects on susceptible cells. However, low concentrations of H2O2 alter cellular functions by modulating signal transduction in certain cells, including endothelial cells.12 Oxidative injury occurs in various ways and has been studied in several diverse models, using different eliciting agents and biological preparations. The common feature of these models is that the primary activation involves radical formation; those radicals and related products then generate a potent oxidant, which subsequently inactivates macromolecules. ROI-mediated damage has been generally associated with necrosis; however, some observations suggest that it has a role in apoptosis.13 Recent observations show that ROIs modulate the expression of Fas and FasL. The expression of Fas was upregulated in human endothelial cells under oxidative stress,14 and the upregulation of FasL has been reported in hepatoma cells,15 microglial cells,16 and glioma cells17,18 under conditions of oxidative stress.
In order to address the effects of oxidative stress in the brain, this study investigated the expression levels of TRAIL in human astroglial cells after H2O2 treatment.
LN215, an astroglioma cell line, was cultured in 10% FCS (Gibco BRL, Grand Island, NY, USA)-DMEM (Gibco BRL) containing 1% nonessential amino acids (Sigma Chemicals Co., St. Louis, MO, USA). The LN215 cell line was kindly provided by Dr. E. G. Van Meir (Department of Neurosurgery, Laboratory of Tumor Biology and Genetics, Lausanne, Switzerland). The cells were incubated with 200, 400, 600, and 800 µM of H2O2 (Sigma) for various times (1-24 hrs). Cyclosporin A (CsA, Sandoz, stock solution 50 mg/mL in DMSO) was added at the conc. of 10, 100 ng, 1, 5, and 10 µg for 30 min before adding H2O2.
1 × 106 cells were cultured in a 75 cm2 flask and washed twice with PBS. H2O2 was added in serum free RPMI medium. Total RNA was isolated with an RNeasy kit (Qiagen, Santa Claris, CA, USA) and 5 µg of this RNA was used to synthesize cDNA with 0.1 O.D. of random hexamer (Pharmacia, Uppsala, Sweden) and 200 U of M-MLV reverse transcriptase (Gibco BRL). The cDNA produced was kept at -20℃ until used.
RT-PCR was performed using the following primer sets: TRAIL primers (forward: 5'-CCC AAT gAC gAA gAg AgT ATg A -3'; reverse: 5'-ggA ATA gAT gTA gTA AAA CCC T-3. PCR conditions were as follows: denaturation at 94℃ for 30s, annealing at 55℃ for 30s, and extension at 72℃ for 30s for TRAIL. The buffer used for PCR was 10 mM Tris-HCl (pH 10), 2.0 mM MgCl2, 50 mM KCl with 1.25 U of Taq polymerase (Takara, Tokyo, Japan). After 27 cycles, an additional extension at 72℃ for 10 min was carried out.
To detect TRAIL expressions, an RNase protection assay was done using the RiboQuant multiprobe RNase protection assay system (Pharmingen, San Diego, CA, USA). Briefly, 15 µg of total RNA was hybridized overnight with the in vitro-translated 32P-labeled RNA probe; hAPO-3C kit and samples were treated with RNase A and proteinase.
A total of 1 × 106 LN215 cells were used for protein purification. Protein was extracted by ultrasonication and centrifuged to remove cell debris. The resulting supernatant was quantified using the Bradford method (Pierce, Rockford, IL, USA). Protein (50 µg) was then loaded onto 12% Tris-HCL SDS-polyacrylamide gel (BioRad, Hercules, CA, USA), and transferred to a nitrocellulose transfer membrane (Amersham Pharmacia Biotech, Piscataway, NJ, USA). TRAIL expression was determined using anti-TRAIL monoclonal Ab (Pharmingen) diluted 1:500 and anti-mouse rabbit Ab (Jackson Immuno Research, Baltimore, MD, USA) was used as a secondary Ab. Proteins were visualized using the ECL technique (Amersham Pharmacia Biotech.).
Astroglial cells (2 × 105 cells/well) were plated in six-well (35-mm2) plates (Costar, Cambridge, MA, USA) and grown to 90% confluency. For analysis of TRAIL protein expression, cells were trypsinized, suspended in PBS containing 5% fetal bovine serum and 0.02% azide, incubated with PE-conjugated anti-human TRAIL antibody (Pharmingen), washed twice, fixed in 1% paraformaldehyde, and then analyzed with FACStar/(Becton Dickinson, Mountain View, CA, USA). Negative controls were incubated with goat anti-mouse IgG antibody conjugated to PE. Ten thousand cells were analyzed for each sample.
To study the expression of functional TRAIL in astroglial cells, 2 × 105 LN215 cells were pretreated with 800 µM of H2O2 for 18 h in serum free medium. Astroglial cells were washed to remove H2O2 and reacted with Peer cells, which were sensitive to TRAIL,19 for 24 hrs. Effector cells (LN 215 cells) and target cells (Peer cells) were then co-cultured at a ratio of 4 : 1. Cell death was measured by staining with annexin V and PI. Control LN215 cells were cultured for 18 hrs in serum-free media without H2O2. Annexin V-FITC and PI (Trevigen, Gaithersburg, MD, USA) were used to detection of cell death.
We hypothesized treatment with H2O2 would modulate the apoptosis-related gene expression of astroglial cells in central nerve system. To address this, human LN215 astroglioma cells were treated with H2O2 and the expression of TRAIL was detected by RT-PCR, RPA, western blot and flow cytometry.
We experimented to determine whether treatment with H2O2 induced TRAIL expression in human astroglial cells. LN215 cells were treated with varying concentrations of H2O2 (0-800 µM) for 4 hrs (for mRNA) or 18 hrs (for protein). H2O2-mediated TRAIL induction was observed by RT-PCR (Fig. 1A) and western blot (Fig 2A). TRAIL expression was induced treatment of H2O2 and reached maximum expression at 800 µM of H2O2 (Fig. 1A, 2A). LN215 cells were treated with 800 µM of H2O2 for various times (0 - 24 hrs). The time course of the TRAIL expression was then determined by RPA (Fig. 1B) or western blot (Fig 2C). The gene expression of TRAIL was induced at 1 h and continued until 24 hrs (Fig. 1B). TRAIL protein expression reached its maximum at 8 hrs and continued until 24 hrs (Fig. 2C). Also H2O2-mediated TRAIL induction was observed by flow cytometry (Fig. 2B).
Cyclosporin A (CsA), an immunosuppressive drug that targets calcineurin, blocked both RNA and protein expression of TRAIL in LN215 cells after treatment with H2O2 (Fig. 3A, 3B). The most effective concentration of CsA started at 100 ng/mL.
To address the biological function of TRAIL in LN215 cells after H2O2 treatment, LN215 cells were co-cultured with Peer cells, a cell line sensitive to TRAIL mediated apoptosis. Annexin V staining of Peer cells showed an increase in apoptotic cells after H2O2 treatment, 64.2% versus 47.5% in the control group (Fig. 4). Peer cells treated with both CsA and H2O2 inhibited TRAIL induced apoptosis down to 49.8%, a level similar to control (Fig. 4).
Our study demonstrates that oxidative stress modulates the expression of TRAIL, which is involved in apoptosis. The up-regulation of FasL and Fas by IFN-γ or TNF-α has been reported in primary astrocytes,20 and H2O2 increased Fas and FasL expression in astroglioma cell lines.18 Although Furuke et al. reported that H2O2 inhibits CD16-induced FasL expression21 and that reactive oxygen species (ROS) suppress events upstream of FasL expression, another report suggested that oxidative stress induces FasL expression in hepatoma and microglial cells.15,16 In other cell types, H2O2 may induce FasL expression by activating NF-κB; H2O2 is known to be a potent activator of NF-κB. Moreover, the CD95L promoter has been described to contain NF-κB binding sites, which suggests that NF-κB may regulate FasL expression.
In contrast to the limited expression of FasL, TRAIL mRNA has been detected in nearly all tissues. However, malignant astoroglioma cells express both TRAIL mRNA and protein.22,23 In our study, TRAIL was detected constitutively in LN 215 cells, an astroglioma cell line, and its expression was increased by H2O2.
In this study, CsA, a pharmacological inhibitor of the phosphatase calcineurin, blocked TRAIL expression at concentrations between 100 and 10 µg/mL. These concentrations are reported to be nontoxic to primary rat astrocytes,24 suggesting that NFAT might be involved in TRAIL expression in human astrocytes. NFAT is known to be expressed in T cells and other immune cells. However, several data show that cells of the nervous system, such as a neuronal cell line and rat astrocytes, express NFAT.24,25 Moreover, the CsA-sensitive signaling pathway has been reported to regulate the survival of reactive astrocytes.24 Involvement of ROS in NFAT has been demonstrated in T cells.27,28 Also, 1.6 kb of the human TRAIL promoter was identified to have binding sites for such factors as NFAT, AP-1, and Sp1.6 Therefore, NFAT, under conditions of oxidative stress, may act as a transcription factor, signaling induction of TRAIL. Although H2O2 has been known to activate NF-κB,29,30 it is not certain whether the expression of TRAIL and its receptors is regulated by H2O2-activated NF-κB. Although basal ROI in tumors can be lower than in normal brain tissue,31 it is important to keep in mind the effects of drug-induced apoptosis on tumor cells by ROI-producing chemotherapies.32
In our study, the upregulation of TRAIL suggests that some astroglial cells up-regulate TRAIL and may abrogate lymphocytes or other immune cells, thereby escaping immune surveillance. Further investigations into the regulation of TRAIL and TRAIL receptors are necessary to answer critical questions related to whether a specific gain of decoy receptors and death receptors in astroglioma cells, as compared with non-neoplastic cells allows the selective therapeutic elimination of tumor cells in vivo.
Collectively our findings suggest that TRAIL may be inducible by hypoxia and other forms of oxidative stress, such as tumor-associated hypoxia or chemotherapy-induced hypoxia. Therefore, oxidative stress leading to the upregulation of TRAIL presumably plays an apoptotic role in pathological conditions of the brain.
Figures and Tables
Fig. 1
Gene expression of TRAIL in human astroglial cells after treatment with hydrogen peroxide (H2O2) in a dose or time-dependent manner. (A) LN215 cells were treated with varying doses of hydrogen peroxide (0 to 800 µM) for 4 hrs. (B) LN215 cells were incubated with hydrogen peroxide 800 µM for various times (0 to 24 hrs). Total RNA was measured for TRAIL mRNA by RT-PCR (A) or RNase protection assay (B). Cells not treated with H2O2 were used as control (CNTL). The data are representative of three experiments.
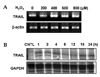
Fig. 2
Expression of TRAIL in human astroglial cells after treatment with H2O2 in a dose or time-dependent manner. (A) LN215 cells were treated with varying doses of hydrogen peroxide (0 to 800 µM) for 18 hrs. (C) LN215 cells were incubated with hydrogen peroxide 800 µM for various times (0 to 24 hrs). For western blot, 20 µg of total protein was loaded in 12% gel and anti-TRAIL monoclonal Ab was used as a primary Ab. (B) FACS analysis of TRAIL expression on LN215 were treated with 800 µM of hydrogen peroxide for 18 hrs. The data are representative of three independent experiments.
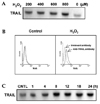
Fig. 3
Inhibition of TRAIL expression by cyclosporin A (CsA) in human astroglial cells after treatment with H2O2. (A) LN215 cells were pre-incubated with CsA (0.01 to 10 µM) for 30 min, were treated with hydrogen peroxide (800 µM) for 4 hrs and then gene expression of TRAIL were measured by RT-PCR. Cells not treated with H2O2 were used as a control. β-actin was used as the internal control. The fold induction was calculated as follows: (TRAIL/β-actin intensity in the study group)/(TRAIL/β-actin intensity in the control group). The fold induction value represents mean ± SD of triplicate experiments. (B) LN215 cells were pre-incubated with CsA (1 µM) for 30 min, were treated with hydrogen peroxide (800 µM) for 18 hrs and then expression of TRAIL were measured by western blot. The data are representative of three independent experiments.
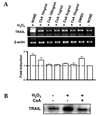
Fig. 4
Induction of apoptosis in Peer cells by human astroglial cells treated with H2O2. LN215 cells were incubated with 800 µM of H2O2 for 18 hrs in serum free medium. LN215 cells were washed to remove H2O2 and then co-cultured with Peer cells for 24 hrs. Effector cells (LN215 cells) and target cells (Peer cells) were co-cultured at a ratio of 4 : 1. Cell death was measured by staining with annexin V. Control LN215 cells were not treated with H2O2, but maintained in serum free condition for 18 hrs. LN215 were pre-incubated for 30 min with CsA (1 µg/mL), and incubated with H2O2. The fold induction value represents a mean ± SD of triplicate experiments.
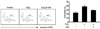
References
1. Wiley SR, Schooley K, Smolak PJ, Din WS, Huang CP, Nicholl JK, et al. Identification and characterization of a new member of the TNF family that induces apoptosis. Immunity. 1995; 3:673–682.
2. Pan G, Ni J, Wei YF, Yu G, Gentz R, Dixit VM. An antagonist decoy receptor and a death domain-containing receptor for TRAIL. Science. 1997; 277:815–818.
3. Mariani SM, Matiba B, Armandola EA, Krammer PH. Interleukin 1 beta-converting enzyme related proteases/caspases are involved in TRAIL-induced apoptosis of myeloma and leukemia cells. J Cell Biol. 1997; 137:221–229.
4. Wajant H, Johannes FJ, Haas E, Siemienski K, Schwenzer R, Schubert G, et al. Dominant-negative FADD inhibits TNFR60-, Fas/Apo1- and TRAIL-R/Apo2-mediated cell death but not gene induction. Curr Biol. 1998; 8:113–116.
5. Pan G, O'Rourke K, Chinnaiyan AM, Gentz R, Ebner R, Ni J, et al. The receptor for the cytotoxic ligand TRAIL. Science. 1997; 276:111–113.
6. Wang Q, Ji Y, Wang X, Evers BM. Isolation and molecular characterization of the 5'-upstream region of the human TRAIL gene. Biochem Biophys Res Commun. 2000; 276:466–471.
7. Frank S, Kohler U, Schackert G, Schackert HK. Expression of TRAIL and its receptors in human brain tumors. Biochem Biophys Res Commun. 1999; 257:454–459.
8. Sheridan JP, Marsters SA, Pitti RM, Gurney A, Skubatch M, Baldwin D, et al. Control of TRAIL-induced apoptosis by a family of signaling and decoy receptors. Science. 1997; 277:818–821.
9. Halliwell B. Reactive oxygen species and the central nervous system. J Neurochem. 1992; 59:1609–1623.
10. Lee YW, Kuhn H, Hennig B, Neish AS, Toborek M. IL-4-induced oxidative stress upregulates VCAM-1 gene expression in human endothelial cells. J Mol Cell Cardiol. 2001; 33:83–94.
11. Zhang Z, Oliver P, Lancaster JR Jr, Schwarzenberger PO, Joshi MS, Cork J, et al. Reactive oxygen species mediate tumor necrosis factor alpha-converting, enzyme-dependent ectodomain shedding induced by phorbol myristate acetate. FASEB J. 2001; 15:303–305.
12. Suhara T, Fukuo K, Sugimoto T, Morimoto S, Nakahashi T, Hata S, et al. Hydrogen peroxide induces up-regulation of Fas in human endothelial cells. J Immunol. 1998; 160:4042–4047.
13. Jacobson MD. Reactive oxygen species and programmed cell death. Trends Biochem Sci. 1996; 21:83–86.
14. Bauer MK, Vogt M, Los M, Siegel J, Wesselborg S, Schulze-Osthoff K. Role of reactive oxygen intermediates in activation-induced CD95 (APO-1/Fas) ligand expression. J Biol Chem. 1998; 273:8048–8055.
15. Hug H, Strand S, Grambihler A, Galle J, Hack V, Stremmel W, et al. Reactive oxygen intermediates are involved in the induction of CD95 ligand mRNA expression by cytostatic drugs in hepatoma cells. J Biol Chem. 1997; 272:28191–28193.
16. Vogt M, Bauer MK, Ferrari D, Schulze-Osthoff K. Oxidative stress and hypoxia/reoxygenation trigger CD95 (APO-1/Fas) ligand expression in microglial cells. FEBS Lett. 1998; 429:67–72.
17. Husain N, Chiocca EA, Rainov N, Louis DN, Zervas NT. Co-expression of Fas and Fas ligand in malignant glial tumors and cell lines. Acta Neuropathol (Berl). 1998; 95:287–290.
18. Kwon D, Choi C, Lee J, Kim KO, Kim JD, Kim SJ, et al. Hydrogen peroxide triggers the expression of Fas/FasL in astrocytoma cell lines and augments apoptosis. J Neuroimmunol. 2001; 113:1–9.
19. Kayagaki N, Yamaguchi N, Nakayama M, Kawasaki A, Akiba H, Okumura K, et al. Involvement of TNF-related apoptosis-inducing ligand in human CD4+ T cell-mediated cytotoxicity. J Immunol. 1999; 162:2639–2647.
20. Choi C, Park JY, Lee J, Lim JH, Shin EC, Ahn YS, et al. Fas ligand and Fas are expressed constitutively in human astrocytes and the expression increases with IL-1, IL-6, TNF-α, or IFN-γ. J Immunol. 1999; 162:1889–1895.
21. Furuke K, Shiraishi M, Mostowski HS, Bloom ET. Fas ligand induction in human NK cells is regulated by redox through a calcineurin-nuclear factors of activated T cell-dependent pathway. J Immunol. 1999; 162:1988–1993.
22. Weller M, Frei K, Groscurth P, Krammer PH, Yonekawa Y, Fontana A. Anti-Fas/APO-1 antibody-mediated apoptosis of cultured human glioma cells. Induction and modulation of sensitivity by cytokines. J Clin Invest. 1994; 94:954–964.
23. Rieger J, Ohgaki H, Kleihues P, Weller M. Human astrocytic brain tumors express APO2L/TRAIL. Acta Neuropathol (Berl). 1999; 97:1–4.
24. Pyrzynska B, Lis A, Mosieniak G, Kaminska B. Cyclosporin A-sensitive signaling pathway involving calcineurin regulates survival of reactive astrocytes. Neurochem Int. 2001; 38:409–415.
25. Ho AM, Jain J, Rao A, Hogan PG. Expression of the transcription factor NFATp in a neuronal cell line and in the murine nervous system. J Biol Chem. 1994; 269:28181–28186.
26. Plyte S, Boncristiano M, Fattori E, Galvagni F, Paccani SR, Majolini MB, et al. Identification and characterization of a novel nuclear factor of activated T-cells-1 isoform expressed in mouse brain. J Biol Chem. 2001; 276:14350–14358.
27. Martinez-Martinez S, Gomez del Arco P, Armesilla AL, Aramburu J, Luo C, Rao A, et al. Blockade of T-cell activation by dithiocarbamates involves novel mechanisms of inhibition of nuclear factor of activated T cells. Mol Cell Biol. 1997; 17:6437–6447.
28. Saccani S, Saccani A, Varesio L, Ghosh P, Young HA, Sica A. Divergent effects of dithiocarbamates on AP-1-containing and AP-1-less NFAT sites. Eur J Immunol. 1999; 29:1194–1201.
29. Schieven GL, Kirihara JM, Myers DE, Ledbetter JA, Ucken FM. Reactive oxygen intermediates activate NF-κB in a tyrosine kinase-dependent mechanism and in combination with vanadate activate the p56lck and p59fyn tyrosine kinases in human lymphocytes. Blood. 1993; 82:1212–1220.
30. Barchowsky A, Munro SR, Morana SJ, Vincenti MP, Treadwell M. Oxidant-sensitive and phosphorylation-dependent activation of NF-kB and AP-1 in endothelial cells. Am J Physiol. 1995; 13:L829–L836.
31. Leaver HA, Williams JR, Ironside JW, Miller EP, Gregor A, Su BH, et al. Dynamics of reactive oxygen intermediate production in human glioma: n-6 essential fatty acid effects. Eur J Clin Invest. 1999; 29:220–231.
32. Lee YS, Wurster RD. Mechanism of potentiation of LY83583-induced growth inhibition by sodium nitroprusside in human brain tumor cells. Cancer Chemother Pharmacol. 1995; 36:341–344.