Abstract
Human embryonic stem (ES) cells can be induced to differentiate into hematopoietic precursor cells via two methods: the formation of embryoid bodies (EBs) and co-culture with mouse bone marrow (BM) stromal cells. In this study, the above two methods have been combined by co-culture of human ES-cell-derived EBs with human BM stromal cells. The efficacy of this method was compared with that using EB formation alone. The undifferentiated human ES cell line SNUhES3 was allowed to form EBs for two days, then EBs were induced to differentiate in the presence of a different serum concentration (EB and EB/high FBS group), or co-cultured with human BM stromal cells (EB/BM co-culture group). Flow cytometry and hematopoietic colony-forming assays were used to assess hematopoietic differentiation in the three groups. While no significant increase of CD34+/CD45- or CD34+/CD38- cells was noted in the three groups on days 3 and 5, the percentage of CD34+/CD45- cells and CD34+/CD38- cells was significantly higher in the EB/BM co-culture group than in the EB and EB/high FBS groups on day 10. The number of colony-forming cells (CFCs) was increased in the EB/BM co-culture group on days 7 and 10, implying a possible role for human BM stromal cells in supporting hematopoietic differentiation from human ES cell-derived EBs. These results demonstrate that co-culture of human ES-cell-derived EBs with human BM stromal cells might lead to more efficient hematopoietic differentiation from human ES cells cultured alone. Further study is warranted to evaluate the underlying mechanism.
Human embryonic stem (ES) cells have the capacity to differentiate into multiple tissue lineages, showing the presence of pluripotent differentiating ability.1-4 These characteristics suggest that human ES cells have the potential to provide an unlimited supply of different cell types for tissue replacement.5 However, human ES cells have not been introduced to clinical practice due to a multitude of biological and ethical limitations. Since the first bone marrow transplantation, hematopoietic stem cells have been introduced for the treatment of incurable hematologic disorders.6,7 However, the sources for hematopoietic stem cells have been limited to bone marrow (BM), umbilical cord, and mobilized peripheral blood tissues.8 Recently, human ES cells have been investigated as an alternative source for hematopoietic stem cells because of their pluripotency and capacity to supply an unlimited number of normal cells. However, in contrast to other organ systems such as neuronal differentiation, the mechanism and optimal methods for hematopoietic differentiation from human ES cells have not been clearly defined.
At present, there are two reported methods for the induction of hematopoietic differentiation from human ES cells. One is the embryoid bodies (EBs) formation method,9 and the other is a co-culture method of human ES cells with a BM stromal cell line.10 EBs are a cluster of human ES cells, and removal of human ES cells from culture conditions containing basic fibroblast growth factor (bFGF) results in the aggregation of human ES cells into EBs.11 These EBs have the capacity to differentiate into all three primary germ layers: endoderm, ectoderm, and mesoderm.12-14 Diverse lineages of cells can develop inside EBs, and the addition of cytokines facilitates hematopoietic differentiation.15,16 On the other hand, co-culture of human ES cells with a BM stromal cell line, such as OP9 (OP9 system), has been reported to be an efficient system for inducing hematopoietic differentiation because the microenvironment surrounding stem cells is important for the differentiation of stem cells into specific lineages.10,17
In this study, BM stromal cells were used instead of OP9 stromal cells for co-culture with human ES cells. When human ES cell-derived EBs were co-cultured with human BM stromal cells, better hematopoietic differentiation was observed than with co-culture of human ES cells with human BM stromal cells.18 However, it is not clear whether hematopoietic differentiation from the co-culture of EBs with human BM stromal cells is due to the intrinsic differentiation potential of EBs or an effect from the microenvironment provided by the co-culture of EBs with human BM stromal cells. Although the hematopoietic cell fate of human ES cells is thought to originate during the period of EB development, there has been no report verifying this question.19 Therefore, this study has attempted to clarify the role of human BM stromal cells in the induction of hematopoietic differentiation from EBs. The SNUhES3 human ES cell line, which was established from Seoul National University and recently described, was used for these studies.20
The human ES cell line SNUhES3 (obtained from Seoul National University, Seoul, Korea) was maintained as undifferentiated cells by culture on feeder layers of irradiated rat embryonic fibroblasts (STO cells, ATCC, USA) in DMEM/F-12 supplemented with 20% Knockout Serum Replacer (Invitrogen Corporation, Carlsbad, CA, USA), 0.1 mM nonessential amino acids (Invitrogen), 0.1 mM β-merc aptoethanol, 1% penicillin-streptomycin (Sigma, St. Louis, MO, USA), and 4 ng/mL recombinant human bFGF (Invitrogen). Undifferentiated SNUhES3 cells were passaged weekly by dissociation under a microscope and cultured at 37℃, 5% CO2, and 95% humidity. Human bone marrow stromal cells were obtained from healthy persons who voluntarily donated their bone marrow stem cells for stem cell transplantation. Written informed consent was obtained from all the donors before bone marrow harvest. The institutional review board of Korea University Medical Center approved this study protocol. Human bone marrow stromal cells were grown and maintained before starting co-culture with embryoid bodies in low glucose DMEM (Invitrogen) containing 10% fetal bovine serum (FBS), and 1% penicillin-streptomycin. For co-culture with embryoid bodies, human bone marrow stromal cells were irradiated (1500 cGy) before being transferred to plates.
Undifferentiated SNUhES3 cells were scraped off and transferred to low-adherence tissue culture plates (Corning Costar Corporation, Acton, MA, USA) to prevent attachment. Embryoid bodies (EBs) were formed two days after transferred SNUhES3 cells were cultured in serum-containing differentiation media: DMEM/F12 supplemented with 20% non-heat inactivated FBS, 1% nonessential amino acids, 0.1 mM β-mercaptoethanol, and 1% penicillin-streptomycin (Sigma). Formed EBs were either maintained in the above serum-containing differentiation media (EB group) or transferred into differentiation media containing a high concentration of serum (EB/high FBS group): DMEM/F12 supplemented with 40% FBS, 1% nonessential amino acids, 0.1 mM β-mercaptoethanol, and 1% penicillin-streptomycin. Media were changed every 2 to 3 days in both groups until the 10th days of culture.
After two days of culture in serum-containing differentiation media, EBs were transferred onto irradiated human bone marrow stromal cell layers and cultured with differentiation media composed of IMDM (Invitrogen Corporation, Carlsbad, CA, USA) supplemented with 12.5 FBS, 12.5% horse serum, L-glutamine, and 1% penicillin-streptomycin (EB/BM co-culture group). Media were changed every 2 to 3 days until the 10th days of culture. After the indicated days from the start of EB formation culture (days 3, 5, 7 and 10), EB-derived differentiated cells were harvested, made into single cell suspensions, and analyzed by flow cytometry and colony-forming cell assays. Hematopoietic differentiation was compared among these three groups: EB, EB/high FBS and the EB/BM co-culture group (Fig. 1).
The mixture of EB-derived differentiated cells was dissociated with 1 mg/mL collagenase type IV (Invitrogen Corporation, Carlsbad, CA, USA) followed by 0.05% trypsin/0.53 mM EDTA supplemented with 2% chick serum (Invitrogen). Dissociated cells were filtered through 85 µM nitex mesh to remove remaining clumps. Single- cell suspensions of EB-derived cells were washed with phosphate-buffered saline (PBS) supplemented with 2% FBS and 0.1% sodium azide. The cells were then incubated with antibodies against human blood cell antigens. First, the single-cell suspensions were stained with CD34- FITC and CD45-PE monoclonal antibodies (Beckman Coulter, Fullerton, CA, USA). After incubation in the dark at 4℃ for 25 minutes, the fraction of CD34+/CD45-cells was detected and analyzed with Cell-Quest Pro Software (Becton-Dickinson, San Jose, CA, USA). Second, the single-cell suspensions were stained with CD34-FITC and CD38-PE monoclonal antibodies (Beckman Coulter, Fullerton, CA, USA). The percentages of CD34+/CD45- and CD34+/CD38-cells were compared among the three groups at each time point.
After culturing for the indicated number of days (7 and 10), single-cell suspensions were prepared from EB-derived differentiated cells in the three groups as described above. Colony-forming assays were performed by culturing 1 × 105 of these cells in semi-solid Methocult® H4444 media (StemCell Technologies, BC, Canada) composed of 1% methylcellulose, 30% FBS, 1% bovine serum albumin (BSA), 50 ng/mL human stem cell factor, 10 ng/mL human granulocyte-macrophage colony-stimulating factor, 10 ng/mL human interleukin-3, and 3 units/mL erythropoietin. After 14 days of culture in a humidified incubator at 37℃ and 5% CO2, the plates were scored for colony-forming cells (CFCs) using inverted microscope and gridded scoring dishes based on the standard criteria of manufacturer's atlas.
There was no significant difference in the percentage of CD34+/CD45-cells among the three groups on days 3 and 5. However, on day 7, an increase in the percentage of CD34+/CD45- cells was found in the EB/BM co-culture group. On day 10, the percentage of CD34+/CD45- cells (3.80% ± 0.58) was significantly higher in EB/BM co-culture group than in EB and EB/high FBS groups (p < 0.05, Fig. 2). Even after 10 days of culture, the percentage of CD34+/CD45- cells was not significantly changed in EB and EB/high FBS groups (0.28% ± 0.23 and 0.35% ± 0.11, respectively). In the three groups, the percentage of CD34-/CD45+ cells and CD34+/CD45+ cells were less than 0.10% regardless of culture duration.
The number of CD34+/CD38- cells increased on day 5 in the EB/BM co-culture group (Fig. 3). The percentage of CD34+/CD38- cells in EB/BM co-culture group (5.81% ± 1.19) was significantly higher than the EB and EB/high FBS groups on days 5, 7, and 10 (p < 0.05, Fig. 2). There was no significant change in the percentage of CD34+/CD38- cells in the EB and EB/high FBS groups throughout the period of culture. In all of the three groups, the percentage of CD34-/CD38+ cells and CD34+/CD38+ cells was also less than 0.10% on the indicated days of culture (days 3, 5, 7, and 10). This time course analysis showed the correlation between CD34+/CD45- cells and CD34+/CD38- cells and also demonstrated that co-culture with human BM stromal cells might increase the hematopoietic differentiation of human ES cells. On days 7 and 10, when a significant increase of CD34+/CD45-/CD38- cells was observed, cultured cells were harvested for colony-forming assays. In the EB and EB/high FBS groups, the mean number of colony-forming cells (CFCs) per 105 cells was not significantly changed on days 7 and 10 (Fig. 4). However, the number of CFCs per 105 cells was increased in EB/BM co-culture on days 7 and 10 (11.0 ± 5.14, 20.6 ± 7.40, respectively), implying a possible role of human BM stromal cells for supporting hematopoietic differentiation from human ES-cell-derived EBs.
The advancement of cell culture techniques has allowed various kinds of in vitro studies and a better understanding of stem cell biology.21-23 In spite of substantial biological and ethical limitations, human ES cells might be a useful candidate for the source of normal cells in clinical practice because of their pluripotency and the presence of established human ES cell lines. In our previous report, a greater number of CD34+/CD45- cells was found when EBs were co-cultured with human BM stromal cells than when undifferentiated human ES cells were co-cultured with human BM stromal cells.18 However, this report had not defined whether the observed hematopoietic differentiation was mainly due to EB formation itself or due to an effect of the co-culture of EBs with human BM stromal cells. Moreover, there has been a recent paper reporting that a subpopulation responsible for hematopoietic and endothelial development was demonstrated inside EBs, suggesting that EBs possess hemangioblastic properties.19
To discriminate between the co-culture effect of EBs with human BM stromal cells and the effect of embryoid body itself on hematopoietic differentiation, we compared the expression of CD34, CD38 and CD45 in the co-culture of EBs and human BM stromal cells (EB/BM co-culture group) and EBs alone (EB group) after the same planned period of culture. In addition, considering that additional FBS was added to the media for co-culture with human BM stromal cells in ours and other previous studies, EBs were also cultured with the addition of 20% FBS (EB/high FBS group).24,25 In this comparison, the percentage of CD34+/CD45- cells was higher in the EB/BM co-culture group than in the EB and EB/high FBS groups, and there was no significant difference between the EB and EB/high FBS groups. Furthermore, the percentage of CD34+/CD38- cells was also higher in the EB/BM co-culture group, consistent with the results of the CD34+/CD45- staining experiments.
In colony-forming assays, a significant increase in the number of colony-forming cells was observed in the cells derived from co-culture of EBs with human BM stromal cells (EB/BM co-culture group), especially on days 7 and 10. These results suggest that an appropriate microenvironment provided by co-culture with human BM stromal cells may be more important for human ES cell-derived hematopoietic differentiation than the differentiation potential of EBs. In the past, the stromal cells used, such as OP9, originated from animals. However, murine stromal cells may provoke unwanted effects in human ES cells because of species differences. Thus, co-culture with human BM stromal cells seems the best plan, but has rarely been tried. This study has demonstrated that the use of human BM stromal cells might be as effective of a culture condition as murine BM stromal cells that were previously reported.26 Our study seems to be the first report showing that human BM stromal cells can offer the correct microenvironment for hematopoietic differentiation of EBs.
In summary, the microenvironment provided by co-culture with human BM stromal cells may play a role inducing the hematopoietic differentiation of human EBs. Therefore, co-culture of EBs with human BM stromal cells might be a feasible way for promoting hematopoietic differentiation of human ES cells. However, because we have used only one human ES cell line, further studies are warranted with different human ES cell lines.
Figures and Tables
Fig. 1
Schematic for the comparison of the EB, EB/high FBS, and EB/BM co-culture groups. Undifferentiated human ES cells at confluence were scraped off and transferred to low-attachment plates in differentiation medium. In the EB group, human ES cells were cultured for EB formation in differentiation medium for 10 days. The EB/high FBS group was cultured for 10 days in the same culture media supplemented with 20% FBS. The EB/BM co-culture group was a co-culture of EBs with human BM stromal cells.
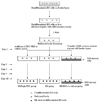
Fig. 2
The percentage of CD34+/CD45- cells (top) and CD34+/CD38- cells (bottom) was significantly higher in the EB/BM co-culture group than in the EB and EB/FBS groups (p < 0.05).
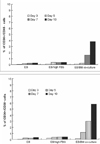
ACKNOWLEDGEMENT
We would like to thank Dr. Shin Young Moon and Sun Kyung Oh for provision of the human ES cell line SNUhES3 and scientific insights for the culture of human ES cells.
References
1. Thomson JA, Itskovitz-Eldor J, Shapiro SS, Waknitz MA, Swiergiel JJ, Marshall VS, et al. Embryonic stem cell lines derived from human blastocysts. Science. 1998. 282:1145–1147.
2. Porada GA, Porada C, Zanjani ED. The fetal sheep: a unique model system for assessing the full differentiative potential of human stem cells. Yonsei Med J. 2004. 45:7–14.
3. Reubinoff BE, Pera MF, Fong CY, Trounson A, Bongso A. Embryonic stem cell lines from human blastocysts: somatic differentiation in vitro. Nat Biotechnol. 2000. 18:399–404.
4. Amit M, Carpenter MK, Inokuma MS, Chiu CP, Harris CP, Waknitz MA, et al. Clonally derived human embryonic stem cell lines maintain pluripotency and proliferative potential for prolonged periods of culture. Dev Biol. 2000. 227:271–278.
5. Suh H. Tissue restoration, tissue engineering and regenerative medicine. Yonsei Med J. 2000. 41:681–684.
6. Blume KG, Beutler E, Bross KJ, Chillar RK, Ellington OB, Fahey JL, et al. Bone-marrow ablation and allogeneic marrow transplantation in acute leukemia. N Engl J Med. 1980. 302:1041–1046.
7. Appelbaum FR, Herzig GP, Ziegler JL, Graw RG, Levine AS, Deisseroth AB. Successful engraftment of cryopreserved autologous bone marrow in patients with malignant lymphoma. Blood. 1978. 52:85–95.
8. Korbling M, Anderlini P. Peripheral blood stem cell versus bone marrow allotransplantation: does the source of hematopoietic stem cells matter? Blood. 2001. 98:2900–2908.
9. Wiles MV. Embryonic stem cell differentiation in vitro. Methods Enzymol. 1993. 225:900–918.
10. Nakano T, Kodama H, Honjo T. Generation of lymphohematopoietic cells from embryonic stem cells in culture. Science. 1994. 265:1098–1101.
11. Xu C, Inokuma MS, Denham J, Golds K, Kundu P, Gold JD, et al. Feeder-free growth of undifferentiated human embryonic stem cells. Nat Biotechnol. 2001. 19:971–974.
12. Itskovitz-Eldor J, Schuldiner M, Karsenti D, Eden A, Yanuka O, Amit M, et al. Differentiation of human embryonic stem cells into embryoid bodies compromising the three embryonic germ layers. Mol Med. 2000. 6:88–95.
13. Levenberg S, Golub JS, Amit M, Itskovitz-Eldor J, Langer R. Endothelial cells derived from human embryonic stem cells. Proc Natl Acad Sci USA. 2002. 99:4391–4396.
14. Schuldiner M, Yanuka O, Itskovitz-Eldor J, Melton DA, Benvenisty N. Effects of eight growth factors on the differentiation of cells derived from human embryonic stem cells. Proc Natl Acad Sci USA. 2000. 97:11307–11312.
15. Chadwick K, Wang L, Li L, Menendez P, Murdoch B, Rouleau A, et al. Cytokines and BMP-4 promote hematopoietic differentiation of human embryonic stem cells. Blood. 2003. 102:906–915.
16. Cerdan C, Rouleau A, Bhatia M. VEGF-A165 augments erythropoietic development from human embryonic stem cells. Blood. 2004. 103:2504–2512.
17. Nakano T. In vitro development of hematopoietic system from mouse embryonic stem cells: a new approach for embryonic hematopoiesis. Int J Hematol. 1996. 65:1–8.
18. Kim SJ, Kim BS, Ryu SW, Sung HJ, Park KH, Choi IK, et al. Differentiation culture of hematopoietic stem cells from embryonic stem cells. Korean J Hematol. 2004. 39:78–85.
19. Wang L, Li L, Shojaei F, Levac K, Cerdan C, Menendez P, et al. Endothelial and hematopoietic cell fate of human embryonic stem cells originates from primitive endothelium with hemangioblastic properties. Immunity. 2004. 21:31–41.
20. Oh SK, Kim HS, Ahn HJ, Seol HW, Kim YY, Park YB, et al. Derivation and characterization of new human embryonic stem cell lines: SNUhES1, SNUhES2, and SNUhES3. Stem Cells. 2005. 23:211–219.
21. Park JC, Park BJ, Suh H, Park BY, Rah DK. Comparative study on motility of the cultured fetal and neonatal dermal fibroblasts in extracellular matrix. Yonsei Med J. 2001. 42:587–594.
22. Kyba M, Perlingeiro RC, Daley GQ. HoxB4 confers definitive lymphoid-myeloid engraftment potential on embryonic stem cell and yolk sac hematopoietic progenitors. Cell. 2002. 109:29–37.
23. Liu L, DiGirolamo CM, Navarro PA, Blasco MA, Keefe DL. Telomerase deficiency impairs differentiation of mesenchymal stem cells. Exp Cell Res. 2004. 294:1–8.
24. Cho SK, Webber TD, Carlyle JR, Nakano T, Lewis SM, Zuniga-Pflucker JC. Functional characterization of B lymphocytes generated in vitro from embryonic stem cells. Proc Natl Acad Sci USA. 1999. 96:9797–9802.
25. Kaufman DS, Thomson JA. Human ES cells--haematopoiesis and transplantation strategies. J Anat. 2002. 200:243–248.
26. Kaufman DS, Hanson ET, Lewis RL, Auerbach R, Thomson JA. Hematopoietic colony-forming cells derived from human embryonic stem cells. Proc Natl Acad Sci USA. 2001. 98:10716–10721.