Abstract
The objectives of this study were to explore whether ovarian vascular endothelial growth factor (VEGF) expression in mice can be regulated by IL-6 (interleukin-6), angiotensin II, FSH, and hCG; and to test whether the mouse ovarian VEGF expression can result in angiogenesis. The ICR mice were sacrificed, and their ovaries were recovered. Recovered ovaries were treated with IL-6, angiotensin II, FSH, and hCG separately and incubated for 24 hours in α-MEM. Expression of mRNA and protein of VEGF were assessed by RT-PCR and immunohistochemistry. The resulting angiogenesis was evaluated through immunohistochemical analysis for CD34. Treatment of mice ovaries with IL-6, FSH, and hCG resulted in a significant increase of VEGF mRNA, and IL-6 was the most potent inducer of VEGF. IL-6 and FSH resulted in increased neovascularization in the follicular phase of mouse ovaries. In contrast, angiotensin II could not increase VEGF expression or neovascularization. We documented an in vitro increase in VEGF expression by IL-6, FSH, and hCG; and reaffirmed that the proliferative response of murine ovarian endothelial cells paralleled an increase of VEGF expression.
The female reproductive system undergoes a number of programmed angiogenic processes, coupled with cyclic changes in the ovarian and endometrial structures. Furthermore, it is well known that a number of female reproduction system disorders are associated with alteration of physiologic angiogenesis or with pathologic angiogenesis. Dysfunction in ovarian angiogenesis may contribute to a variety of disorders including anovulation, infertility, pregnancy loss, ovarian hyperstimulation syndrome, and ovarian neoplasms.1
Angiogenesis of the ovary is initiated early during follicular development and continues throughout follicular growth.2 The preovulatory follicle acquires a complex vascular network and the granulosa cells receive a rich vascular supply from the thecal capillaries penetrating the basement membrane. This dynamic change in angiogenesis differs from one follicle to another, and the selection of the dominant follicle may depend on the differential formation of this rich vascular supply.1,3
Vascular endothelial growth factor (VEGF) is a specific mitogen for vascular endothelial cells and has a central role in ovarian angiogenesis. It has been suggested that several factors are involved in the dynamic pattern of VEGF expression during follicular growth. Chistenson et al demonstrated a role for a midcycle surge of gonadotropin in primates to promote VEGF production by granulosa cells in the periovulatory follicle of Rhesus monkeys.4 The treatment of various cell lines with IL-6 resulted in a significant induction of VEGF mRNA,5 and angiotensin II has been shown to stimulate the expression of angiogenic factors in bovine luteal cells.6 These results suggest the presence of regulators of VEGF expression in the ovary. Most of these studies were performed during late follicular or luteal phase. However, little is known about the VEGF regulation during follicular phase.
The aim of this study is to address this issue, first by identifying several factors such as IL-6, angiotensin II, hCG, and FSH which regulate the VEGF expression during the follicular phase; and second, by identifying the changes of VEGF expression, if any, resulting from these factors, which may contribute to neovascularization.
Thirty 6-week-old ICR mice were killed and their ovaries were removed. Ten mice were used for RT-PCR, another 10 mice for immunohistochemistry for VEGF, and the remaining 10 mice for immunohistochemistry for CD34. Out of the 2 ovaries of each mouse, the larger ovary was removed and sliced into 5 pieces using an operative microscope. The ovaries were incubated for 24 hours in α-MEM medium containing 1 µM angiotensin II (Human angiotensin II acetate, Sigma, Germany), 50 ng/mL IL-6 (Recombinant mouse IL-6, Sigma, Germany), 7.5 mIU/mL FSH (Gonal-F, Serono, Switzerland), or 500 mIU/mL hCG (Profasi, Serono, Switzerland) for each group.
Ovarian tissue was homogenized in 1 mL TRIzol reagent (Gibco BRL, Life Sciences, Carlsbad, CA, USA). Total RNA was separated from DNA and proteins by adding chloroform and precipitated with the use of isopropanol. The precipitate was washed twice in 75% ethanol, air dried, and rediluted in DEPC-treated distilled water. The amount and the purity of the isolated RNA were quantified by spectrophotometry.
One µg of total RNA was used to synthesize cDNA using a 1st strand cDNA synthesis kit (Roche, Diagnostics Corp. Indianapolis, IN, USA). Mastermix per sample was prepared as follows: 2 µL of 10 × reaction buffer, 2 µL of 10-3 M dNTP mixture, 2 µL of 2.5×10-3 M oligo-p(dt)15, 4 µL of 5×10-3 M MgCl2, 0.8 µL of 50 U RNase inhibitor, and 1 µL of 20 U AMV-reverse transcriptase. The mixture was incubated at room temperature for 10 minutes, 42℃ for 1 hour, then at 95℃ for 5 minutes using a PCR thermal cycler. To amplify cDNA, 2 µL total cDNA from each RT tube were mixed with Mastermix containing 50×10-3 M KCl, 10×10-3 M Tris-HCl (pH 9.0), 0.1% Triton X-100, 2×10-3 M MgCl2, 10×10-3 M dNTP mixture, 1.25 IU Taq polymerase, and 50 pmol of each primer for VEGF or β-actin.
The VEGF primers were designed to amplify a region common to all VEGF isoforms. The sense VEGF primer (5'-GAA GTC CCA TGA AGT GAT CAA G-3') and primer 3' of each isoforms were used (Table 1). The PCR products for 5 isoforms, VEGF 120, VEGF 144, VEGF 164, VEGF 188, and VEGF 205 would be 331, 404, 333, 407, and 425bp, respectively.
PCR reactions were carried out with the following program, first heated to 94℃ for 5 minutes, then 28 cycles of 94℃ for 30 seconds, 62℃ for 30 seconds, and 72℃ for 30 seconds, with a final elongation step at 72℃ for 10 minutes. The electrophoresis of the PCR product was carried out in 2% agarose gel stained with 0.5% ethidium bromide. After completion of electrophoresis, the bands were analyzed on an image analyzer.
Ovarian tissues were fixed in 10% formalin in PBS and then embedded in paraffin. Paraffin-embedded ovaries were sectioned in 4 µm thickness. The tissue sections were deparaffinized in xylene and dehydrated in a graded series of ethanol. An immunohistochemistry for VEGF was performed with streptoavidin-biotin-peroxidase complex method using goat antimouse VEGF polyclonal antibody (Santa Cruz Biotechnology, Santa Cruz, CA, USA). The endogeneous peroxidase was quenched with 3% hydrogen peroxide at room temperature for 5 minutes, then the tissue sections were rinsed three times for 5 minutes each time in TBS. The sections were incubated with normal serum, then incubated with goat antimouse VEGF polyclonal antibody at a dilution of 1:200 in PBS/BSA overnight at 4℃. After three washes with TBS for 15 minutes each, the sections were incubated with biotinylated secondary antibody for 60 minutes at room temperature and washed three times. The samples were incubated with streptavidin-peroxidase conjugate in PBS for 30 min at room temperature, washed three times, and incubated with 3.3'Diaminibenzidine chromogen in Tris buffer containing H2O2 for 5 minutes. Immunohistochemistry for CD34 was performed by the avidin-biotin-peroxidase complex method using mouse anti-CD34 monoclonal antibody (Santa Cruz Biotechnology, Santa Cruz, CA, USA). Counterstaining was performed with Mayer's hematoxylin. The results were assessed by one pathologist as -, +, ++, and +++. In CD34 immunostaining, only stromal staining was regarded as neovascularization.
Data obtained for VEGF mRNA expression are presented as means and standard error. RT-PCR data were analyzed by a one-way analysis of variance (ANOVA) with a significance level set at p < 0.05. Data of immunohistochemistry were evaluated by Friedman's two-way ANOVA and p values < 0.05 were considered significant.
The predominant isoforms of VEGF were VEGF 120 and VEGF 164. VEGF 144 was expressed in very low levels (Fig. 1, 2). As VEGF 188 was not expressed in vivo and VEGF 144 was expressed in very low levels, we excluded these isoforms from the data analysis.
The treatment with IL-6 increased the VEGF mRNA expression. The ratio between VEGF 120 and actin increased from 0.75 to 1.17 (p = 0.012), and the ratio between VEGF 164 and actin increased from 0.65 to 0.92 (p = 0.036, Fig. 1).
The exposure of mouse ovary to FSH resulted in induced VEGF 120 mRNA (p = 0.009) and VEGF 164 (p = 0.015). Similarly, the hCG treated mouse ovary resulted in increased VEGF 120 mRNA (p = 0.023) and VEGF 164 (p = 0.054, Fig. 1).
Although there was an increasing trend in the expression of VEGF mRNA with treatment of angiotensin II, it did not reach statistical significance.
In the IL-6 and FSH treated mouse ovaries, VEGF immunostaining seemed to have increased even though it did not reach statistical significance (p = 0.0845, Table 2, Fig. 3). The result of CD34 immunostaining revealed an increase of small sprouting capillaries when IL-6 was used. A similar trend was observed with VEGF staining (p = 0.0458, Table 3, Fig. 4).
In most adult tissues, angiogenesis occurs only rarely during tissue repair. Pathological angiogenesis is associated with various disorders, including tumor growth,7 diabetic retinopathy,8 rheumatoid arthritis,9 and atherosclerotic lesions.10 The female reproductive tract is unique because this is the only site in the uninjured adult body where angiogenesis occurs in a repetitive cyclic fashion, modulated by various factors.11 Furthermore, these dramatic changes in the ovarian vasculature are associated with follicle growth, atresia, and corpus luteal development and regression. This ovarian angiogenesis is a complex process in which a delicate balance between promoters and inhibitors is maintained. Disturbance of this balance may result in a disrupted physiologic state or various pathologic conditions such as polycystic ovarian syndrome, ovarian hyperstimulation syndrome, and benign and malignant neoplasms.11
A critical regulator of ovarian angiogenesis is VEGF. VEGF is a mitogen for vascular endothelium and exists in five isoforms resulting from alternative splicing of the same gene.1 VEGF expression varies during the ovarian cycle. The VEGF mRNA and protein are initially absent from the granulosa cell of the primordial follicle and the preantral follicles but become evident in the theca cells of antral follicles and in the granulose cells nearest the oocyte in preovulatory follicles. During the periovulatory interval, VEGF levels in the follicular fluid increase markedly.3,11 After ovulation, VEGF mRNA and protein expression are observed in the granulosa and theca-derived luteal cells.12
The dramatic changes in ovarian angiogenesis, especially VEGF expressions, are associated with follicle growth and atresia.12 However, elevated follicular fluid levels of VEGF are observed in poor responders, which is related to reduced oxygen delivery, resulting from an increased resistance at the level of the perifollicular arteries.13
VEGF is expressed not only in follicles but also in the stroma of the ovary, which provide nutritional support for primordial and primary follicles that survive in large proportion during cryopreservation and transplantation. Thus, it is likely that the regulation of VEGF in the ovarian follicular phase produces benefits in ovarian stimulation of poor responders and also in ovarian tissue grafted protocols.
Several mechanisms are involved in the regulation of VEGF gene expression. VEGF mRNA is highly expressed by the induction of hypoxia via hypoxia-inducing factor-1 (HIF-1). Many cytokines and growth factors, such as transforming growth factor (TGF)-α, basic fibroblast growth factor (bFGF), intelerukin-6, and angiotensin II, could also induce VEGF mRNA expression and protein secretion in various tissues.7 Besides, lysophospholipidic acid (LPA) and cell differentiation also play important roles in the regulation of VEGF expression.1 There is a suggestion that different regulatory pathways are involved in VEGF regulation. However, what exactly causes the regulation of this critical angiogenic factor in the ovary remains to be established. Moreover, the molecular mechanisms that regulate the VEGF expression during the early follicular phase are not elucidated.
To identify the respective angiogenic factors in the follicular phase of the ovary, the present study was focused on VEGF, a major angiogenic factor that is responsible for cyclic angiogenesis of the female reproductive tract, and its regulators in the follicular phase.
We evaluated whether IL-6 could induce VEGF expression. IL-6 is a cytokine that acts on various cell types. Motro et al. demonstrated that IL-6 mRNA is transiently expressed during angiogenesis that accompanies folliculogenesis, suggesting its angiogenic role.14 When IL-6 was added to the culture media, we found that the VEGF mRNA expression increased significantly. Our data suggest that IL-6 might be considered as an indirect inducer of angiogenesis that exerts its activity through the induction of VEGF. Moreover, it is noteworthy that, among the other factors included in our study, IL-6 was the most potent inducer of VEGF expression.
Several studies suggest that FSH and LH/hCG modulate the expression of VEGF. Christenson demonstrated that the midcycle surge of FSH and LH/hCG in primates promotes VEGF production by granulosa cells in the periovulatory follicle.4 As expected from previous studies, our study supports the evidence of gonadotropin's role in angiogenesis.4,15 VEGF production stimulated by gonadotropin leads to more blood flow and more input of gonadotropin, in particular, to the dominant follicle of the natural cycle.16 Although a possible role of protein kinase C is suggested, cAMP-dependent protein kinase A plays a key role in the gonadotropin-dependent increase in VEGF production. The latter is supported by one particular study, which demonstrated the stimulatory effect of both gonadotropin and 8-Br-cAMP on VEGF production by Leydig cells.17
Therefore, our observations are indicative of important features in determining that gonadotropin is a potent stimulator of angiogenic growth factor, which might be of benefit in the challenging field of ovarian grafting and in vitro maturation. As shown in our study, gonadotropins could up-regulate VEGF, and exogeneous gonadotropin could increase the number of surviving follicles in ovarian tissue grafts.18 This result was supported by the observation that use of gonadotropins may be beneficial in vitro maturation of oocytes.19
Although several studies indicated the proangiogenic role of angiotensin II, which exerts effects through two distinct subpopulations of angiotensin II receptors, AT1 and AT2,20,21 our study could not observe any correlation between the expression of angiotensin II and VEGF. This is in contrast to an earlier finding showing that angiotensin II might regulate VEGF expression. Tamarat et al demonstrated that angiotensin II induced an increase of VEGF in mice.21 Hayashi et al suggested that angiotensin II might regulate angiogenesis in the developing bovine corpus luteum.6 This discrepancy may be explained by several studies indicating the variation in angiotensin II receptor distribution and concentration among species. In rat ovaries, the AT1 receptor was primarily found in the stroma, whereas AT2 was expressed on the granulosa cells of atretic follicles.22 In contrast, rabbit granulose cells express AT2 receptors in the preovulatory follicle.23 Further studies are necessary to gain insights as to how angiotensin II induces neovascularization in different species, in particular in human ovaries.
In addition, we evaluated whether the increase in VEGF mRNA by several factors resulted in VEGF protein production through immunohistochemistry. Although we could not obtain a statistical significance, there was an increasing tendency in VEGF staining (Fig. 3). However, we did observe more CD34 positive cells in IL-6 and FSH, in which VEGF mRNA levels were increased (Fig. 4). The discrepancy between mRNA expression and immunohistochemisty of VEGF might have resulted because of the small number of mouse ovaries. Another possible explanation is that some modification in regulation at the posttranscriptional level might exist, resulting in differences between mRNA and protein expression. These data, however, strongly argue in favor of the important influence of IL-6 and FSH in mediating the induction of the VEGF protein and finally ovarian angiogenesis during the follicular phase of the ovary.
CD34 is known to be a specific marker for endothelial cells and is used to study ovarian blood vessels.24 The endothelial cell layer of small vessels in the superficial ovarian stroma tended to be positive for CD34 in IL-6 and FSH treated ovaries, in contrast to the control and angiotensin II-treated ovaries for which that was the case in only a few blood vessels.
Because perifollicular angiogenesis supplies gonadotropins and many growth factors to the granulosa cells of follicles, angiogenesis of stroma is associated with the promotion of follicular development. While Shimizu et al. demonstrated that VEGF-treated ovaries of miniature gilts promoted perifollicular vascularization,25 our data might support the concept that VEGF also promote perifollicular angiogenesis that is essential for follicles in the early stage.
In summary, we demonstrated that IL-6, FSH, and hCG are angiogenic factors that act indirectly by inducing VEGF expression in mouse ovaries. This evidence, supplemented by immunohistochemical data indicative of VEGF activity, provides the possibility that ovarian angiogenesis might be regulated through the induction of VEGF expression by IL-6, FSH, and probably hCG.
This issue should be a subject for further clinical investigation because induced neovascularization by the regulation of VEGF would be a challenging therapeutic approach in female reproductive diseases. Furthermore, in the future, this VEGF regulation may assist in the application of antiangiogenic medications in the therapy of conditions including polycystic ovary syndrome, ovarian hyperstimulation, and ovarian carcinomas.
Figures and Tables
Fig. 1
The values on the Y-axis represent mean values of the ratio between VEGF and actin. *p = 0.012 §p = 0.036 †p = 0.009 ∥p = 0.015 ‡p = 0.023 ¶p = 0.054.
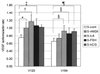
Fig. 2
The semiquantitative RT-PCR analysis of the gene expressions of the specific VEGF isoforms were normalized with the expression of the housekeeping gene, β-actin. M: Molecular weight marker, Cont: control, IL-6: interleukin-6, FSH: Follicle stimulating hormone, hCG: Human chorionic gonadotrophin, Ang II: Angiotnesin II.
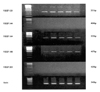
Fig. 3
(A) Immunohistochemical staining showing VEGF expression of a mouse ovary. (B) VEGF expression in a mouse ovary treated with IL-6. (C) VEGF expression in a mouse ovary treated with FSH. (D) VEGF expression in a mouse ovary treated with hCG. (E) VEGF expression in a mouse ovary treated with Angiotensin II.
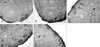
Fig. 4
(A) Immunohistochemical staining showing CD 34 of a mouse ovary. (B) CD34 expression in a mouse ovary treated with IL-6. (C) CD34 expression in a mouse ovary treated with FSH. (D) CD34 expression in a mouse ovary treated with hCG. (E) CD34 expression in a mouse ovary treated with Angiotensin II.
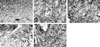
References
1. Geva E, Jaffe RB. Role of vascular endothelial growth factor in ovarian physiology and pathology. Fertil Steril. 2000. 74:429–438.
2. Wulff C, Wiegand SJ, Saunders PT, Scobie GA, Fraser HM. Angiogenesis during follicular development in primate and its inhibition by treatment with truncated Flt-1-Fc. (vascular endothelial growth factor Trap(A40)). Endocrinology. 2001. 142:3244–3254.
3. Redmer DA, Reynolds LP. Angiogenesis in the ovary. Rev Reprod. 1996. 1:182–192.
4. Christenson LK, Stouffer RL. Follicle stimulating hormone and luteinizing hormone/chorionic gonadotropin stimulation of vascular endothelial growth factor production by macaque granulosa cells from pre- and periovulatory follicles. J Clin Endocrinol Metab. 1997. 82:2135–2142.
5. Cohen T, Nahari D, Cerem LW, Neufeld G, Levi BZ. Interleukin 6 induces the expression of vascular endothelial growth factor. J Biol Chem. 1996. 271:736–741.
6. Hayashi K, Miyamoto A, Berisha B, Kosmann MR, Okuda K, Schams D. Regulation of angiotensin II production and angiotensin receptors in microvascular endothelial cells from bovine corpus luteum. Biol Reprod. 2000. 62:162–167.
7. Song KH, Song J, Jeong GB, Kim JM, Jung SH, Song J. Vascular endothelial growth factor- its relation to neovascularization and their significance as prognostic factors in renal cell carcinoma. Yonsei Med J. 2001. 42:539–546.
8. Stehouwer CD, Lambert J, Donker AJ, van Hinsbergh VW. Endothelial dysfunction and pathogenesis of diabetic angiopathy. Cardiovasc Res. 1997. 34:55–68.
9. Koch AE. Review: angiogenesis: implications for rheumatoid arthritis. Arthritis Rheum. 1998. 41:951–962.
10. Kang SM, Kwon HM, Hong BK, Kim D, Kim IJ, Choi EY, et al. Expression of leptin receptor (Ob-R) in human atherosclerotic lesions: potential role intimal neovascularization. Yonsei Med J. 2000. 41:68–75.
11. Anteby EY, Hurwitz A, Korach O, Revel A, Simon A, Finci-Yeheskel Z, et al. Human follicular nitric oxide pathway: relationship to follicular size, oestradiol concentrations and ovarian blood flow. Hum Reprod. 1996. 11:1947–1951.
12. Stouffer RL, Martinez-Chequer JC, Molskness TA, Xu F, Hazzard TM. Regulation and action of angiogenic factors in the primate ovary. Arch Med Res. 2001. 32:567–575.
13. Battaglia C, Genazzani AD, Regnani G, Primavera MR, Petraglia F, Volpe A. Perifollicular Doppler flow and follicular fluid vascular endothelial growth factor concentrations in poor responders. Fertil Steril. 2000. 74:809–812.
14. Motro B, Itin A, Sachs L, Keshet E. Pattern of interleukin 6 gene expression in vivo suggests a role for this cytokine in angiogenesis. Proc Natl Acad Sci USA. 1990. 87:3092–3096.
15. Wang TH, Horng SG, Chang CL, Wu HM, Tsai YJ, Wang HS, et al. Human Chorionic gonadotropin-induced ovarian hyperstimulation syndrome is associated with up-regulation of vascular endothelial growth factor. J Clin Endocrinol Metab. 2002. 87:3300–3308.
16. Anasti JN, Kalantaridou SN, Kimzey LM, George M, Nelson LM. Human follicle fluid vascular endothelial growth factor concentrations are correlated with luteinization in spontaneously developing follicles. Hum Reprod. 1998. 13:1144–1147.
17. Anand RJ, Paust HJ, Altenpohl K, Mukhopadhyay AK. Regulation of vascular endothelial growth factor production by Leydig cells in vitro: the role of protein kinase A and mitogen-activated protein kinase cascade. Biol Reprod. 2003. 68:1663–1673.
18. Imthurn B, Cox SL, Jenkin G, Trounson AO, Shaw JM. Gonadotrophin administration can benefit ovarian tissue grafted to the body wall: implications for human ovarian grafting. Mol Cell Endocrinol. 2000. 163:141–146.
19. Einspanier R, Schnfelder M, Muller K, Stojkovic M, Kosmann M, Wolf E, et al. Expression of the vascular endothelial growth factor and its receptors and effects of VEGF during in vitro maturation of bovine cumulusoocyte complexes(COC). Mol Reprod Dev. 2002. 62:29–36.
20. Williams B, Baker AQ, Gallacher B, Lodwick D. Angiotensin II increases vascular permeability factor gene expression by human vascular smooth muscle cells. Hypertension. 1995. 25:913–917.
21. Tamarat R, Silvestre JS, Duriez M, Levy BI. Angiotensin II angiogenic effect in vivo involves vascular endothelial growth factor and inflammation related pathways. Lab Invest. 2002. 82:747–756.
22. Kotani E, Sugimoto M, Kamata H, Fujii N, Saitoh M, Usuki S, et al. Biological roles of angiotensin II via its type 2 receptor during rat follicle atresia. Am J Physiol. 1999. 276:E25–E33.
23. Yoshimura Y, Karube M, Aoki H, Oda T, Koyama N, Nagai A, et al. Angiotensin II induces ovulation and oocyte maturation in rabbit ovaries via the AT2 receptor subtype. Endocrinology. 1996. 137:1204–1211.
24. Gaytan F, Morales C, Garcia-Pardo L, Reymundo C, Bellido C, Sanchez-Criado JE. A quantitative study of changes in the human corpus luteum microvasculature during the menstrual cycle. Biol Reprod. 1999. 60:914–919.
25. Shimizu T, Jiang JY, Iijima K, Miyabayashi K, Ogawa Y, Sasada H, et al. Induction of follicular development by direct single injection of vascular endothelial growth factor gene fragments into the ovary of miniature gilts. Biol Reprod. 2003. 69:1388–1393.