Abstract
This study examined the effectiveness of Holmium-166 (Ho-166) chitosan complex therapy for a malignant glioma. Cultured C6 glioma cells (100,000 in 5 µl) were injected into the caudate/putamen of 200 - 250 gram Wistar rats. Five days later, a Ho-166 chitosan complex was injected into the same site of the glioma injection. Four injection doses were administered: the control group received PBS 10 µl, group 1 received an injection of 100 µCi (10 µl), group 2 received an injection of 50 µCi (5 µl), and group 3 received an injection of 10 µCi (1 µl). The average tumor volume for each group was 1.385 mm3 for the control group, 0.036 mm3 for group 1, 0.104 mm3 for group 2, and 0.111 mm3 for group 3. Compared with the control group, the size of the tumors in groups 1, 2 and 3 was reduced by an average of 97.4%, 92.5% and 91.9%, respectively. The Kaplan-Meier survival curve of group 2 was the longest, followed by groups 3, group 1 and the control. The mean survival was 22.8, 59, 60, and 44.6 days for the control group and groups 3, 2 and 1, respectively. H-E staining revealed that group 2 yielded the best results in the destruction of the malignant glioma. TUNEL staining and immunohistochemical studies indicated apoptotic features. The Ho-166 chitosan complex proved to be effective in destroying the malignant glioma.
A malignant glioma that occurs in the brain tends to invade the surrounding tissue. Consequently, this tumor cannot be removed completely by a simple resection using a craniotomy. Moreover, it does not respond well to chemotherapy or radiotherapy, which means that such treatment methods serve merely as a supplementary role in the prolongation of life. Despite the advances in modern medicine, the growth of a tumor cannot be effectively repressed or controlled with surgery, chemotherapy, radiotherapy or recent immunotherapy and gene therapy especially for malignant gliomas.1-3
Nevertheless, radiotherapy is the treatment that offers temporary relief. External beam radiation is most widely used, and radiosurgery using a linear accelerator or a Gamma knife has recently been tried. Theoretically, the use of stereotactic techniques may play a role in escalating the radiation dose safely. However, such a high dose is associated with a higher risk of unacceptable normal tissue toxicity.
In order to reduce the side effects of radiotherapy and to enhance the treatment effects of malignant brain tumors, it is necessary to localize the radiation on the tumor area precisely, for which a treatment method using a radionuclide with weak penetration power within the tissues would be useful i.e. a β-emitting radionuclide. When using radiation with a weak penetration power, its isotopes should be inserted into the tumor tissues. This method is known as brachytherapy using isotopes such as irridum-192, palladium-103, and iodine-125.4-6 However, brachytherapy for brain tumors requires complicated surgical procedures, which involve the insertion and fixation of the radioactive isotopes for a certain period of time and then removing them. This procedure has a high chance of recurring complications such as infection, hemorrhage, and tumor metastasis of7,8 In order to overcome these limitations, radiopharmaceuticals that are injected as liquids, diffuse into the tissues, and become gelatinized after a certain period of time in order to be retained in the tissue would be ideal. Ho-166 emits β rays with a high energy and a short half-life of 26.83. It decays to stable daughter products and has a higher energy corresponding to Y-90 by emitting 94% β rays [average 0.61 MeV, maximum 1.766 MeV (48%), 1.855 MeV (51%)] and 6% γ rays [0.081 MeV (6.24%), 1.379 MeV (0.93%)]. Because Ho-166 emits a small proportion of γ rays, it can generate an image on the gamma camera. The penetration range of Ho-166 into the soft tissues is 1.23 mm on average, with a maximum of 8.6 mm. When it is injected into the tumor, 90% of the energy is deposited within the range of 2.1 mm, and the remaining 10% is deposited within the range of 2.1 - 8.6 mm, suggesting that it is intensively irradiated on the tumor only, whilst preserving the surrounding normal tissues. Therefore, Ho-166 has been successfully used in animal experiments using internal radiotherapy.9-11 In addition, chitosan, as a chelating agent, has been attracting increasing attention as a biomaterial and as a pharmaceutical excipient for drug delivery owing to its favorable biological properties. Chitosan is obtained by the N-deacetylation from chitin, which is the second most common natural polymer. The N-deacetylation of chitin leads to a cationic polymer, which has a pH-dependent solubility in water. It exists in liquid form when the acidity is 4.0 or lower, and after it is injected into the body and meets the neutral body fluid, it becomes gelatinized as the pH increases to 6.5. Because of these characteristics, chitosan has the potential to be retained at the injected site for a long period of time.12-14 Therefore, Ho-166-chitosan complex can theoretically prevent the side effects caused by the radioaction that can occur by using these radioisotopes internally, by not remaining in the area of the injection and by extending to other internal organs. Furthermore, it can avoid the limitation of having to remove the radioisotopes that have been inserted.14
The aim of this study was to identify the effects of a Ho-166-chitosan complex on the destruction of a malignant brain tumor and the repression of its growth in rats as a preliminary step prior to attempting clinical trials on patients with a malignant brain tumor. Primarily, the reactions of the brain tissues in the rats whose cell strain of C6 glioma had been xenografted were compared with the brain tissues of normal rats. At the same time, the survival rate of the rat tumor model was determined.15,16 Finally, the progression processes of apoptosis and necrosis in the residual tumor cells were examined using immunohistochemical stains such as transferase-mediated deoxyuridine triphosphate nick end labeling (TUNEL) stains, and Bcl-2 and Bax stains.
The cell strain of the C6 glioma was incubated in Dulbecco's modified Eagle's medium (DMED; Pharmacia, Peapack, NJ, USA) containing 10% fetal bovine serum (HyClone; Sigma, St. Louis, MO, USA). All the incubated substances included 2 mM L-glutamine and gentamycin per 50 µg/ml. All the cells were incubated at 37℃ in 5% CO2/ 95% air. The adhered cells were trypsinized in DMEM before being transplanted in the albino rats.
The rats were anesthetized with ketamine (75 mg/kg), acepromazine (0.75 mg/kg), and rompun (4 mg/kg), and the tumor cells were injected into the cranium using a Hamilton syringe (Fischer Scientific, Tustin, CA, USA) with 30-gauge oblique needles. The target coordinates were 2.5 mm left from the bregma and 5.5 mm deep from the dura mater. The total number of C6 glioma cells to be transplanted was 100,000 in 5 µl, and to prevent any leakage the injection needle was left in place for 4 minutes after the 5-minute injection, which was then slowly removed over a 1 minute period. Five days after the tumor cell transplantation, 10 µl of 100 µCi, 5 µl of 50 µCi, and 1 µl of 10 µCi of Ho-166 chitosan complex, and 5 µl of normal saline, in groups 1, 2, 3, and the control, respectively, were injected into the same area in the same manner as in the tumor cell transplantation for the four different groups, each of ten 200 - 250 gram Wistar rats. In order to calculate the survival rate, a follow-up observation was carried out until death on five of the ten rats in each group without obtaining tissue specimens.
Of the other five rats, four were anesthetized with ether and sacrificed 5 days after injecting the Ho-166 chitosan complex injection, and their chests were excised. The right atriums were incised and a cannula was inserted into the left ventricle, which was refluxed in 120 mM NaCl/2.7 mM KCL containing a 10 mM phosphate buffer of pH 7.4 and 3.7% paraformaldehyde. The brain was immersed in the same fixing fluid for 30 minutes, and frozen with dry ice after a low temperature treatment in 30% sucrose phosphate-buffered saline (PBS). The tissue sections were sliced at a 12 µm thickness, and mounted on slides coated with chrome alum/gelatin.
The cross-sectional area of the tumor region and the number of transplanted cells per tumor section were measured by image analysis using a Microcomputer imaging device. The estimated value for the entire tumor volume was induced from the tumor area with the largest cross-section. The degree of Ho-166 chitosan complex penetration into the tumor area was examined using histological tests, and the tissue was stained with Hematoxylin-Eosin (H&E) staining method and observed microscopically.
The brain tissue of the rat model with a brain tumor, which had been damaged by the Ho-166 chitosan complex embedded in the paraffin was sliced at a 4 mm thickness, mounted on a tissue slide, dried at room temperature, and the paraffin was removed using xylene. After proteinase K (DAKO Corp., Carpinteria, CA, USA) was dropped onto the tissue and reacted at room temperature, the target area that had been covered during fixation became exposed via proteolytic digestion. The tissue slide was soaked in 3% hydrogen peroxide in PBS for 5 minutes at room temperature in order to quench the endogenous peroxidase reaction, and washed in the normal saline solution. The other rat brain tissues were stained using a kit (ApopTag Peroxidase kit, Intergen Co., Purchase, NY, USA). An equilibration buffer was dropped onto the slide until the tissue was covered, reacted at room temperature, and lightly swept away, while the working strength TdT enzyme, which was made by combining the reaction buffer and TdT enzyme at a ratio of 7 : 3 was added and incubated in a 37℃ humidified chamber for 1 hour. After leaving the 1 hour-incubated tissue slide in the stop/wash buffer, the slide was incubated for 10 minutes at room temperature whilst being slightly shaken. Subsequently, the slides were washed with a normal saline solution, while an anti-digoxigenin peroxidase conjugate was applied, and incubated for 30 minutes in a humidified chamber at room temperature. After washing the slide with a normal saline solution, the color was developed with a DAB solution at room temperature. Within the damaged tissues, the number of stained cells was measured from the area where the TUNEL staining was most frequently detected by optical microscopy at a 200 x magnification.
Immunohistochemical staining was carried out
using the Bcl-2 antibody (Polyclonal rabbit anti-rat/mouse Bcl-2 antibody, Pharmingen Co., San Diego, CA, USA), which is known to repress apoptosis, and the Bax antibody (Polyclonal rabbit anti-mouse/rat Bax antibody, PharMingen Co., San Diego, CA, USA), which facilitates apoptosis in the Bcl-2 family on the cerebral tissue fixed in paraffin. The color was developed after reacting it with a biotin-combined second antibody.
The primary antibody (anti-Bcl-2 Ab, anti-Bax Ab) was applied to the tissue, which was incubated at 4℃ for 30 minutes and at room temperature overnight. After washing the tissue with PBS, it was incubated for 10 minutes after applying the biotinylated anti-rabbit/anti-mouse immunoglobulin provided in the kit (DAKO LSAB kit, DAKO Corp., Carpinteria, CA, USA). After rinsing with PBS, it was incubated for 10 minutes after applying streptavidin peroxidase. After further washing with PBS, the color was developed using DAB (3,3-diaminobenzidine, DAKO Corp., Carpinteria, CA, USA).
The tissues of the brain tumor were observed microscopically at a 200 × magnification, from the area where the Bcl-2 or Bax staining was most frequently detected.
After the injection of the Ho-166 chitosan complex on the fifth day after the transplantation of the cultured tumor cells, 10 µl of 100 µCi, 5 µl of 50 µCi, and 1 µl of 10 µCi of the Ho-166 chitosan complex, and 5 µl of PBS were injected into each group. After 5 days, 4 rats from each group were sacrificed to obtain the brain tissue specimens. It was verified that the tumor transplantation had been done successfully in all tissues (Fig. 1). The size of the tumor (mm3) was calculated using the formula of 4/3p width (a, µm) length (b, µm) and depth (c, µm).
The percentage reduction of the tumor volume relative to that in the control group was 97.4%, 92.5%, and 91.9% in groups 1, 2 and 3, respectively showing a decreasing rate (Table 1, Fig. 2). Furthermore, by injecting each dose into the normal brain and grossly observing it, group 1 showed cavitation involving extensive destruction around the area of the injection. Although groups 2 and 3 showed destruction of the brain tumor tissues around the area of the injection, there was no observation of cavitation as extensive as observed in group 1.
In order to calculate the survival rate, 5 experimental rats were kept alive until their natural death without obtaining tissue specimens. The Kaplan-Meier survival curve was the longest in group 2, which was followed by groups 3 and 1, and finally the control group. The median survival times were calculated for each group. The median survival time was 22 days in the control group, 60 days in group 3, 60 days in group 2, and 36.5 days in group 1. The difference in the survival rate of each group was statistically significant (p < 0.001, Fig. 3).
On the fifth day after injecting the Ho-166 chitosan complex, 4 rats in each group were sacrificed in order to obtain tissue specimens of the malignant cerebral glioma.
H-E staining of the cerebral glioma located in the putamen showed a malignant cerebral glioma with abundant cytoplasm and nuclei. Compared with the control group, group 1 showed cavitation where almost all the cells were destroyed and a coagulation necrosis of tumor cells was observed.
In contrast, group 2 did not show any cavitation at the center of injection but there was a coagulation necrosis of cells with hypochromasia and no observation of nuclei. The tumor cells that had become necrotic were arranged in the shape of a belt, and living tumor cells were observed in the surrounding area. In group 3, the range of coagulation necrosis was not as wide as observed in group 2, and more live tumor cells were observed than in group 2 (Fig. 4).
TUNEL staining indicated that all three of the experimental groups that had been injected with the Ho-166 chitosan complex showed a positive finding where the nucleus was positively stained, and the frequency of benign cells was higher than the capacity of the Ho-166 chitosan complex. This means that the average benign findings observed by optical microscopy at 200×magnification were 19.6%, 30.4%, and 36.7% in groups 1, 2 and 3, respectively. There was a positive reaction observed in the area that appeared to be a radionecrosis in the normal cells around the tumor cells in group 3 (Table 2, Fig. 5, 6).
For the Bax antibody, there was extensive cytoplasm staining observed around the area where the tumor had been destroyed in group 1 (Fig. 7). Although there was a similar finding in group 2, group 3 showed almost no staining. For Bcl-2, none of the groups showed any staining (data not shown).
More than half of the gliomas, which make up 40 - 50% of all primary brain tumors, were malignant. Although they do not metastasize to other organs, they infiltrate into the surrounding normal tissues around the area of occurrence. In addition, most of them relapse despite active treatments such as surgery, radiotherapy, and chemotherapy due to their very rapid speed of growth rate. The prognosis is very poor with an average survival time of 1 year or less.3,17
Local infiltration of the tumor is a special feature of a malignant glioma that makes a complete recovery impossible. The infiltration is known to extend up to 2 - 3 cm from the area of the contrast enhancement on diagnostic imaging.18
The primary treatment for a brain tumor is the surgery. The aim of surgery is to maximize the effects of the supplementary treatment by removing as much of the tumor as possible without causing neurological impairment and to assist in the histological diagnosis. Although diagnostic imaging tools, surgical tools, and surgical techniques have advanced by leaps and bounds, a complete recovery is impossible with surgery alone, and supplementary treatments such as radiotherapy, chemotherapy, and immunotherapy, in addition to gene therapy, which is in the early stage of research, are being performed. BCNU, ACNU, and PCV (procarbazine, lomustine, and vincristine) have been used for chemotherapy, but their effects are weaker than those for the tumors in other internal organs. This is because of the heterogeneity, the blood-brain barrier, the blood-tumor barrier, and the low blood stream volume of the tumor cells. In order to overcome the insufficient delivery of the drug as a result of the blood-brain barrier and the blood-tumor barrier, interstitial chemotherapies using an injection pump, catheter, and polymer have been introduced. 5-FU, methotrexate, bleomycin, cisplatin, and ACNU have been used, but without any improvement in the treatment effects.19
Immunotherapy and gene therapy for a cerebral glioma have been developed as a result of the poor specificity and selectivity of the previous treatment methods. The side effects are severe with high IL-2 or TNF-α doses. Interferon-β is being clinically used but its definite therapeutic effects have not been proven. Active studies on gene therapy are being carried out along with the recent development of molecular and cell biology.1,2
Radiotherapy has been proven to be the most effective supplementary treatment, and the survival rate of cerebral glioma patients has doubled over the last 10 years.2,3 However, such radiotherapeutic methods often cause radionecrosis as a result of irradiation on the surrounding normal tissues, resulting in various side effects such as a cerebral edema. Such side effects can aggravate the neurological symptoms in the patients, interrupt the patients' normal living for the rest of their lives, and can even lead to death if severe enough.20-23
Brachytherapy, which is performed to enhance the treatment effects and reduce the side effects associated with radiotherapy, is a treatment method that uses a toxic dosage of radiation to the specific tumor while minimizing the irradiation on the surrounding normal tissues. However, brachytherapy for cerebral tumors requires complicated surgical procedures that involve the insertion and fixation of the radioactive isotopes for a certain period of time and then removal of them. Accordingly, there is a high probability of complications such as infection, hemorrhage, and metastasis. In order to complement such limitations, isotopes that are injected as liquids, penetrate the tissues, and finally become gelatinized after a certain period of time would be ideal.5,6
Lee et al. reported the treatment effect of the Ho-166 chitosan complex by injecting it directly into the hepatoma (SK-HEP1) through a subcutaneous transplantation into the nude mouse, resulting in the radionecrosis of the hepatoma with almost no side effects on the other tissues or internal organs.24 The therapeutic effects of the Ho-166 chitosan complex have been proven using animal experiments and clinical studies in various types of skin cancers or Bowen's disease. In addition, it has also shown good therapeutic effects for squamous cell carcinoma, basal cell carcinoma, and Bowen's disease in clinical studies using a specially designed, Ho-166 chitosan complex-attached, skin patch.10,11 Good results for knee bursitis have also been shown in rheumatoid arthritis patients.25 Suzuki et al have reported the results of the biodistribution and kinetics of intrahepatically administered Ho-166-chitosan complex and 166Ho alone in rats. They found that most of the administered radioactivity was exclusively retained at the administration site for over 72 hr. On the other hand, the intrahepatic administration of 166Ho alone showed a higher radioactive concentration in the blood from the early period, and evidence that it had spread to many tissues indicated that the effective biological half-life at the administration site was much longer than that at the other tissues.26
In order to clinically use such isotopes for a malignant cerebral glioma, the presence or absence of the tumor necrosis by the Ho-166 chitosan complex, its range along with its appropriate injection volume should all be calculated. Lee et al. directly injected a Ho-166 chitosan complex into a hepatoma (SK-HEP1) through subcutaneous transplantation into nude mice. Despite the fact that the entire volume injected was small at 0.025 ml, 3.7 mCi was reported to achieve complete necrosis of a 1 cm diameter hepatoma, and only 10.94 mCi was needed for a 2 cm diameter tumor.25 It was separately reported that when 1 mCi was injected into a skin melanoma transplanted to a white rat, with an average tumor size of 1 cm, there was the possibility for complete elimination.12 In this study, the average tumor volume was 1.385 mm3 for the control group, 0.036 mm3 for the 100 µCi injection group, 0.104 mm3 for the 50 µCi injection group, and 0.111 mm3 for the 10 µCi-injection group. As noted previously, there was some variation in the tumor volume (Table 1), but this was not statistically significant. The percentage reduction for the tumor volume relative to that in the control group was 97.4%, 92.5%, and 91.9% in groups 1, 2 and 3, respectively, all showing a tumor-volume decrease rate of over 90%. However, group 1 showed extensive cavitation that involved the normal tissues resulting in a marked decrease in the survival rate compared with that of the other groups. This was considered to be not only due to the excessive radiation dose, but also because of the excessive injection dose (10 µl) and the fact that the injection dose was not quantified in each group. The survival rates in groups 2 and 3 were significantly higher than in the control group (p < 0.001). The therapeutic effects for a malignant cerebral glioma were shown at the 10 µCi and 50 µCi doses by decreasing the tumor volume and extending the survival rate. Group 2 appeared to be superior to group 1 in terms of the level of tumor destruction according to H-E and TUNEL staining, but there was no significant difference in the survival rate and the decreasing rate of the tumor volume.
Lee et al. have reported that for a skin melanoma, a Ho-166 chitosan complex destroyed the central area of the injection on the tumor as a result of necrosis, and that the peripheral area, where the radiation dose was low, showed a halt in growth.
Immunohistochemical staining indicated that apoptosis contributed to a halting of the tumor growth according to the RT-PCR and TUNEL-staining. However, the morphological findings, gross analyses and flow cytometry did not reveal any apoptosis, and morphologically, the cessation of tumor growth and secondary necrosis appeared to be caused by necrosis.12
This study also showed consistent findings of apoptosis according to the TUNEL staining results and the findings from immunohistochemical staining. However, according to the morphological findings, which showed cavitation, the mechanism of action for the Ho-166 chitosan complex on the tumor appears to be cytonecrosis. TUNEL staining indicated that the positive findings were temporary and reactions to DNA damage were observed within the area of tumor necrosis.
It is believed that the Ho-166 chitosan complex has the potential to be an additional tool for the treatment of a malignant cerebral glioma. However, research into the appropriate dose is needed before clinical trials on the human body can commence.
Through our study on the therapeutic effects of the Ho-166 chitosan complex in the malignant cerebral tumor model of rats, it was found that the Ho-166 chitosan complex can be used for treating malignant cerebral glioma when injected at the appropriate dose. The benefits of the Ho-166 chitosan complex are based on minimizing the irradiation dosage to the normal tissues around the tumor and intensively irradiating a toxic β rays dose to the tumor, thereby achieving necrosis of the malignant tumor without a direct impairment or side effects through dissociation into the other surrounding organs.
The Ho-166 chitosan complex is dose-dependently effective in the cytonecrosis of a malignant cerebral glioma, although at an excessive dosage of 100 µCi side effects extending to the surrounding normal tissues were observed. It also prolonged life substantially by destroying the cerebral glioma and halting its growth. However, its effect on prolonging life was not as great at an excessive injection dose.
The mechanism of action for destroying the glioma was coagulation necrosis, as reported in other studies. In future studies, the Ho-166 chitosan complex promises to be a supplementary tool in the treatment of cerebral glioma through an injection using a stereotactic method, and in the surgical treatment for recurring tumors through injection in the surgical field.
Overall, further studies on the appropriate injection dose relative to the tumor volume and the clinical efficacy will be needed.
Figures and Tables
Fig. 1
Gross images for each group. In the control group, a successful tumor implant in the left putamen can be observed. In group 1, cavitation (arrows) can be observed in spots where the Ho-166 chitosan complex was over-administered. In groups 2 and 3, the implanted tumors are substantially reduced in size due to destruction by Ho-166 chitosan complex.
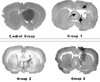
Fig. 2
Comparison of the tumor volume for each group. The average tumor volume for the control group was 1.385 mm3. For group 1, it was 0.036 mm3, for group 2, it was 0.104 mm3, and for group 3 it was 0.111 mm3. The ratio of the tumor volume against the control volume was 97.4% for group 1, 92.5% for group 2, and 91.1% for group 3, showing a gradual reduction.
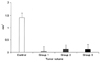
Fig. 3
Survival curve for each group. Curves for groups 2 and 3 extended further than the control and group 1, and this extension was statistically significant (p < 0.001).
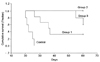
Fig. 4
H&E stain images. Under 100 × and 400 × magnification, malignant cerebral tumors with abundant nucleus and cytoplasm could be observed in the control group. In group 3, some tumor cells destroyed by Ho-166 chitosan complex could be observed, but the areas with active tumor cells were larger than in the other groups. In group 2, necrosis of the tumor cells were observed more extensively. In group 1, cavities (almost all cells completely destroyed with no structure) could be observed around the centers of administration (marked with arrow). Destruction spread even into normal tissues, and tumor cells showed the appearance of coagulation necrosis.

Fig. 5
TUNEL stain images. In all experimental groups with the Ho-166 chitosan complex administration, there were positive results with the nucleus being stained (arrowed). In group 3, positive reactions were observed even in the normal cells around a tumor cell where it appeared to be going through radionecrosis.

Fig. 6
Comparison of TUNEL-stain-positive rats. The average positive rates were 19.6% for group 3, 30.4% for group 2, 36.7% for group 1.
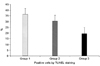
ACKNOWLEDGEMENT
We thank Ms. YH Cho and Professor HJ Ahn for their technical assistance and discussion for completion of this manuscript.
References
1. Chang JW, Lee H, Kim E, Lee Y, Chung SS, Kim JH. Combined antitumor effects of an Adenoviral Cytosine Deaminase/Thymidine Kinase fusion gene in rat c6 glioma. Neurosurgery. 2000. 47:931–939.
2. Brandes A, Soesan M, Fiorentino MV. Medical treatment of high grade malignant glioma in adults: An overview. Anticancer Res. 1991. 11:719–727.
3. Nieder C, Grosu AL, Molls M. A comparison of treatment results for recurrent malignant gliomas. Cancer Treat Rev. 2000. 26:397–409.
4. Bampoe J, Glen J, Hubbard SL, Salhia B, Shannon P, Rutka J, et al. Adenoviral vector-mediated gene transfer: timing of wild-type p53 gene expression in vivo and effect of tumor transduction on survival in a rat glioma brachytherapy model. J Neurooncol. 2000. 49:27–39.
5. Bampoe J, Laperriere N, Pintilie M, Glen J, Micallef J, Bernstein M. Quality of life in patients with glioblastoma multiforme participating in a randomized study of brachytherapy as a boost treatment. J Neurosurg. 2000. 93:917–926.
6. Patel S, Breneman JC, Warnick RE, Albright RE Jr, Tobler WD, van Loveren HR, et al. Permanent iodine-125 interstitial implants for the treatment of recurrent glioblastoma multiforme. Neurosurgery. 2000. 46:1123–1130.
7. Oppenheimer JH, Levy ML, Sinha U, El-Kadi H, Apuzzo ML, Luxton G, et al. Radionecrosis secondary to interstitial brachytherapy: correlation of magnetic resonance imaging and histopathology. Neurosurgery. 1992. 31:336–343.
8. Houston SC, Crocker IR, Brat DJ, Olson JJ. Extraneural metastatic glioblastoma after interstitial brachytherapy. Int J Radiat Oncol Biol Phys. 2000. 48:831–836.
9. Mumper RJ, Ryo UY, Jay M. Neutron-Activated Holmium-166-Poly (L-Lactic Acid) Microspheres: A Potential Agent for the Internal Radiation Therapy of Hepatic Tumors. J Nucl Med. 1991. 32:2139–2143.
10. Chung YL, Lee JD, Bang D, Lee JB, Park KB, Lee MG. Treatment of Bowen's disease with a specially designed radioactive skin patch. Eur J Nucl Med. 2000. 27:842–846.
11. Lee JD, Park KB, Lee MG, Kim EH, Rhim KJ, Lee JT, et al. Radionuclide Therapy of Skin Cancers and Bowen's Disease Using a Specially Designed Skin Patch. J Nucl Med. 1997. 38:697–702.
12. Lee JD, Yang WI, Lee MG, Ryu YH, Park JH, Shin KH, et al. Effective local control of malignant melanoma by intratumoral injection of a beta-emitting radionuclide. Eur J Nucl Med. 2002. 29:221–230.
13. Vila A, Sanchez A, Janes K, Behrens I, Kissel T, Jato JL, et al. Low molecular weight chitosan nanoparticles as new carriers for nasal vaccine delivery in mice. Eur J Pharm Biopharm. 2004. 57:123–131.
14. Lee WY, Moon EY, Lee J, Choi CH, Nam SC, Park KB, et al. Toxicities of 166 Holmium-chitosan in mice. Arzneimittelforschung. 1998. 48:300–304.
15. Bradford R, Darling JL, Thomas DGT. The development of an animal model of glioma for use in experimental neurooncology. Br J Neurosurg. 1989. 3:197–210.
16. Engebraaten O, Hjortland GO, Hirschberg H, Fodstad O. Growth of precultured human glioma specimens in nude rat brain. J Neurosurg. 1999. 90:125–132.
17. Chandler KL, Prados MD, Malec M, Wilson CB. Long-term survival in patients with glioblastoma multiforme. Neurosurgery. 1993. 32:716–720.
18. Ammirati M, Vick N, Liao YL, Ciric I, Mikhael M. Effect of the extent of surgical resection on survival and quality of life in patients with supratentorial glioblastomas and anaplastic astrocytomas. Neurosurgery. 1987. 21:201–206.
19. Levin VA. Chemotherapy for brain tumors of astrocytic and oligodendroglial lineage: the past decade and where we are heading. Neuro-oncol. 1999. 1:69–80.
20. Nwokedi EC, DiBiase SJ, Jabbour S, Herman J, Amin P, Chin LS. Gamma knife stereotactic radiosurgery for patients with glioblastoma multiforme. Neurosurgery. 2002. 50:41–47.
21. Park YG, Chung SS, Kim DI, Chang JW, Cho J. Complications following Gamma Knife radiosurgery. Stereotact Funct Neurosurg. 1995. 64:Suppl 1. 239–248.
22. Kureshi SA, Hofman FM, Schneider JH, Chin LS, Apuzzo ML, Hinton DR. Cytokine expression in radiation-induced delayed cerebral injury. Neurosurgery. 1994. 35:822–830.
23. Plowman PN. Stereotactic radiosurgery. VIII. The classification of postradiation reactions. Br J Neurosurg. 1999. 13:256–264.
24. Lee YH. Effect of Holmium-166 Injection into Hepatocellular Carcinomas (SK-HEP1) Heterotransplanted in Mice. Journal of the Korean Radiological Society. 1998. 38:83–92.
25. Song J, Suh CH, Park YB, Lee SH, Yoo NC, Lee JD, et al. A phase I/IIa study on intra- articular injection of Holmium-166 chitosan complex for the treatment of knee synovitis of rheumatoid arthritis. Eur J Nucl Med. 2001. 28:489–497.
26. Suzuki YS, Momose Y, Higashi N, Shigematsu A, Park KB, Kim YM, et al. Biodistribution and kinetics of Holmium-166-chitosan complexes in rats and mice. J Nucl Med. 1998. 39:2161–2166.