Abstract
The aim of this study was to determine the usefulness of early magnetic resonance imaging findings in predicting neurologic recovery at or below the injured level in traumatic cervical spinal cord injuries. Thirty patients with traumatic cervical spinal cord injuries were included. All of the patients received a magnetic resonance imaging and a neurologic examination in the emergency room, within 7 days of injury and at 6 months following the injury. To quantify neurologic recovery below the injured level, we modified clinical scales, particularly the motor ratio and the sensory ratio. We used the neurologic level to quantify recovery around the injured level. We assessed neurologic recovery according to MRI patterns and lesion extents. The pure hemorrhagic MRI pattern was not observed. In edematous and mixed types, the improvement of neurologic levels was not significantly different. The motor ratio and sensory ratio improved significantly more in edematous type patients than in mixed type patients. Based on MRI lesion extent, the improvement of neurologic levels was not significantly different, and motor ratio and sensory ratio improved significantly more in those with one or two segments involved than in those with more than two segments involved. In conclusion, early MRI pattern and lesion extent after traumatic cervical spinal cord injury may provide important information to help predict neurologic recovery, especially below the injured level.
Traumatic spinal cord injuries result in significant, severe individual disabilities and social burden. Moreover, the number of incomplete lesions has increased recently1 because of improved management techniques and subsequent immediate care. Approximately half of all traumatic spinal cord injuries are cervical lesions. The neurologic level at which injury most often occurs is C5, followed by C4 and C6.1
One of the most difficult tasks facing medical staff that care for patients with spinal cord injuries may be predicting the neurologic prognosis and discussing it with the patients and their family. It is important that clinicians are familiar with recovery courses of traumatic spinal cord injuries, as this knowledge allows them to predict outcomes and develop realistic, comprehensive rehabilitation programs.2,3 In addition, knowledge of the expected recovery course helps determine the effectiveness of various interventions, such as pharmacologic treatment and rehabilitative treatment.
Previously, the prognosis and severity of spinal cord injuries have been assessed by standardized neurologic examinations, electrophysiologic data such as somatosensory evoked potentials 14,5 and motor evoked potentials,5-8 and radiological approaches such as computed tomography9,10 and myelography.11 The most accurate way of predicting recovery is by standardized physical examination, as endorsed by the International Standards for Neurologic and Functional Classification of Spinal Cord Injury Patients.12 However, neurologic examinations are limited, as prognostic predictions may be more accurate when based on examinations at 72 hours after acute injury than on initial emergency examinations. This is because the immediate examination may be affected by associated injuries, alertness, medications, and evolving deficits.13,14 Magnetic resonance imaging (MRI) is currently the technique that best visualizes the spinal cord and adjacent soft tissues.15-21 MRI has been used as an imaging modality for the diagnosis of spinal cord injury and is known to be helpful in determining the prognosis of neurologic recovery.22-31
Neurologic recovery following spinal cord injury occurs both below and in the vicinity of the injured spinal cord level.32
However, the majority of previous studies have not separately investigated neurologic outcomes at and below the injured level.22-31 Furthermore, they reported neurologic outcomes using crude and inappropriate scales for quantifying neurologic recovery, such as the Frankel classification or the American Spinal Injury Association (ASIA) classification.22,26,30 Other studies have used motor and sensory scores (such as the ASIA motor and sensory scores) and the motor index score as parameters.22,24,26,29 Motor and sensory scores are absolute scales of the whole body's motor and sensory systems and represent the neurologic status both at and below the neurologic level. However, they are inappropriate for measuring neurologic recovery below the injury level, especially in spinal cord injury patients with different initial neurologic levels. Moreover, the status of neurologic recovery has not been fully or quantitatively evaluated over a constant post-traumatic period because the majority of previous research has been retrospective in nature.
For these reasons, we modified two relative scales-the motor ratio and the sensory ratio-to allow us to evaluate the amount of neurologic recovery below the neurologic level regardless of differences in initial neurologic level.
The aim of this study was to determine the usefulness of early MRI findings in predicting neurologic recovery at and below the neurologic level by using a prospective approach with relative scales.
Our study consisted of 30 tetraplegic patients who had suffered a traumatic cervical spinal cord injury. There were 25 males and 5 females with ages ranging from 23 to 69 years (mean age: 39.4 years). Potential study subjects suspected of having another spinal cord injury or traumatic brain injury during initial evaluation and those in whom MRI was contraindicated were excluded from the study.
Neurologic evaluations were conducted at the department of emergency medicine by neurosurgeons or orthopaedic surgeons, followed by evaluation by physiatrists six months after the injury. Injured spinal cord neurologic status was evaluated at the neurologic level by ASIA classification and by using ASIA motor and sensory scores according to International Standards for the Neurologic and Functional Classification of Spinal Cord Injury Patients.12 ASIA motor and sensory scores were used to determine the motor ratio and sensory ratio, which minimizes ceiling effect in patients with different neurologic levels. We then used these indices to objectively compare neurologic changes below the level of injury. Motor ratio and sensory ratio were defined as follows:
Maximal ASIA motor score and sensory score below the neurologic level were defined as the ASIA motor (or sensory) score below the neurologic level; this can be obtained when the motor or sensory system was intact below the neurologic level. Thus, this score was different in subjects with the different neurologic level and was higher in patients with a higher neurologic level.
We also calculated the recovery rates of the motor and sensory ratios to compare the degree of improvement of the motor and sensory ratios based on the methods of Lucas and Ducker.33 The recovery rates were calculated as follows: [motor or sensory ratio (%) at follow-up-initial motor or sensory ratio (%)]/[100-initial motor or sensory ratio (%)] × 100.
MRI studies were undertaken within 7 days of injury in the acute stage. All scans were obtained at 1.5 T using sagittal and targeted axial T1 and T2-weighted sequences. A neuroradiologist who was blinded to clinical details reviewed all MRI data. Individual cases were classified by MRI in terms of lesion pattern and extent. Initially, we modified patterns described by Kulkarni17 by adding a transection type (previously described by Flanders et al.3). The criteria used to define these types were based on alterations in the sagittal intensity of the spinal cord as detected by T1 and T2-WI sequences with respect to the time elapsed since trauma. These criteria were as follows: Type I pattern (hemorrhagic type): the spinal cord on T1-WI is heterogeneous within the first 72 hours following trauma; on T2-WI, a large central area of low signal intensity surrounded by a thin, high intensity peripheral ring is visible. Seventy-two hours to one week after the injury, the spinal cord showed hyperintensity on T1 and T2-WI. Type II pattern (edematous type): normal image on T1-WI with high signal intensity on T2-WI. Type III pattern (mixed type): normal image on T1, but the spinal cord presents with a small central isointense area and a thick, high-intensity peripheral ring on T2. Type IV pattern (transaction type): sagittal discontinuity of the spinal cord. Kulkarni's classification was proved using an animal study.34 Ohta et al.34 reported on an experimental study conducted to evaluate MRI signal changes within injured cervical cords in rats. They observed a low T2 spinal cord signal soon after the injury (reflecting hemorrhage) and low T1/high T2 during the subacute to chronic period (indicating the presence of edema).
Secondly, according to lesion extent, cases were allocated to group I or group II. Cases were allocated to group I when one or two segments showed altered lesion intensity and to group II, when three or more segments showed altered lesion intensity.
We compared motor ratio and sensory ratio with respect to MRI pattern and extent using the Student's t-test. Initial neurologic levels were compared using the Mann-Whitney test. We compared the six-month follow-up motor and sensory ratios with the initial ratios using a paired t-test. The neurologic levels were compared using the Wilcoxon Signed Ranks test for non-parametric, paired data. The recovery rates of the motor and sensory ratios from the initial evaluation to the six-month follow-up between the groups were compared using the Student's t-test. Univariate ANOVA was used to compare the recovery rate of motor ratio and sensory ratio, according to the MRI pattern and extent, without effects of age. Statistical significance was defined as p < 0.05.
The initial neurologic levels in the 30 patients were as follows: C2, one patient; C3, five patients; C4, twelve patients; C5, seven patients; C6, two patients; C7, one patient; T1, two patients. According to ASIA classification, 22 were ASIA A, 3 were ASIA B, 3 were ASIA C, and 2 were ASIA D (Table 1).
The frequency of MRI patterns were as follows: type I (hemorrhagic type), not found; type II (edematous type), found in 15 patients; type III (mixed type), found in 14 patients; type IV (transection type), found in 1 patient. Based on MRI lesion extent, 13 patients fit into group I (below and two segments) and 16 patients fit into group II (above and three segments). A patient with transection could not be classified according to lesion extent and was excluded. Additional MRI findings are described in Table 2.
No patients of pure hemorrhagic type were observed. Only one patient was classified as transection type. Therefore, we compared the edematous and mixed types. Initially, no significant difference in neurologic level was found between the edematous and mixed type groups. Six months after injury, we did not find any cases that had worsened. The follow-up neurologic level had significantly improved compared to the initial neurologic level in both groups (p <0.05) (Table 3). In the edematous type patients, the neurologic level tended to improve more than in mixed type patients, but this difference was not significant (Table 3).
The mean motor ratio of the edematous type patients tended to be higher than those of the mixed type, and the mean sensory ratio of the edematous type was significantly higher than that of the mixed type at the initial evaluation. After six months, the mean motor ratio improved from 13.45% to 35.10% in the edematous type patients and from 4.12% to 6.35% in the mixed type patients (both were statistically significant, p < 0.05) (Table 4). Also, the mean sensory ratio significantly improved, increasing from 19.95% to 50.27% in patients of edematous type and from 7.27% to 17.14% in patients of mixed type (p < 0.05) (Table 4). The recovery rates of the motor and sensory ratios are shown in Fig. 1; these were significantly higher in edematous type patients than in mixed type patients (p < 0.05) (Fig. 1). Univariate ANOVA using age as a covariate showed a significant difference in motor recovery rate between the groups (F=22.3, p < 0.01). The same ANOVA showed a trend of difference in sensory recovery rate between the two groups (F=4.0, p=0.06). In the case with transaction, no changes were found at the neurologic level, while the recovery rates of the motor and sensory ratios were 2.25% and 2.25%, respectively.
At the initial evaluation, no significant differences were observed between the neurologic levels of the two groups. The six-month follow-up neurologic level improved significantly compared to the initial neurologic level in both groups (p < 0.05) (Table 5). In group I (one or two segments involved), the neurologic level had improved more than in group II (more than two segments involved), but this was not significant (Table 5).
The mean motor and sensory ratios of patients in group I tended to be higher than those of patients in group II at the initial evaluation; however, this was not significant. The mean motor ratio at the follow-up evaluation significantly improved compared to the initial evaluation, increasing from 13.92% to 33.18% in group I and from 4.91% to 11.50% in group II (p < 0.05) (Table 6). The mean sensory ratio also improved over the same period, increasing from 18.74% to 47.02% in group I and from 9.84% to 23.92% in group II (p < 0.05) (Table 6). The recovery rates of the motor and sensory ratios are shown in Fig. 2. The recovery rates of the motor and sensory ratios were significantly higher in group I than in group II (p < 0.05) (Fig. 2). Univariate ANOVA with age as covariate showed a significant difference in motor recovery rates between the two groups (F=5.3 p < 0.05) and showed a trend of difference in sensory recovery rate between the groups (F=3.1, p=0.09).
Restoration of neurologic function may be the result of the resolution of acute injuries such as hemorrhages or secondary injury processes such as ischemia, edema, or inflammation or the result of nervous tissue regrowth or regeneration.35 In addition, the recovery of neurologic function after SCI may occur at different anatomical structures. With respect to this anatomic basis of clinical recovery, neurologic recovery after SCI may be the result of nerve root regeneration at the level of the injury or of recovery of the spinal cord itself. In the latter case, cord recovery may be the basis for improved function of caudal structures innervated by long tracts at the injury site.32
Many studies have used the Frankel or ASIA classification, motor and sensory index scores, or ASIA motor and sensory scores to determine neurologic outcome.22,24,26,30 However, the Frankel classification and ASIA classification are inappropriate and crude and do not allow the detection of minimal neurologic changes. Motor and sensory index scores and ASIA motor and sensory scores contain all of the neurologic status around and below the neurologic level. Also, because spinal cord injuries occur at different levels, neurologic recovery following spinal cord injury cannot be measured exactly using the ASIA motor and sensory scores (which are faulty because of a ceiling effect). So, these are inappropriate for measuring neurologic recovery below the injured level, especially in spinal cord injury patients with injuries at different initial levels. For these reasons, we modified and used the motor and sensory ratios, which provide a quantitative measure of neurologic recovery below the injured spinal cord level in patients with different initial neurologic levels. In the present study, we quantitatively determined neurologic recovery below the injury level based on MRI findings.
Motor ratio and sensory ratio-measures of neurologic status below the injured level-improved by a significantly greater amount in edematous type patients than in mixed type patients; this also occurred to a significantly greater degree in group I than in group II. Our results agree with previous studies in terms of neurologic recovery with respect to MRI signal changes,3,22,25-31 and in terms of the closeness of the relationship between lesion extent and neurologic recovery.3,29,30
However, previous studies have not found neurologic recovery in patients with hemorrhage by MRI.15-17 In Ramon's retrospective study,28 patients with a hemorrhagic spinal cord injury showed complete spinal cord injury at admission; no patients showed neurologic changes at discharge or during follow-up. However, patients of edematous type showed incomplete spinal cord injury at admission and experienced favorable outcomes (ASIA D or E) at the final evaluation. In our study, we found significant neurologic recovery despite hemorrhage. Other researchers23,30,31,36 concur: for example, Seldon et al.30 studied the relationship between emergency MRI findings and prognosis and reported that three of these patients (14%) experienced functional motor recovery. Discrepancies between studies are probably due to differences in the timing of the MRI. An edematous or mixed type diagnosis after spinal cord injury was based on the existence or non-existence of hemorrhage. Consequently, prognosis is poorer in hemorrhagic cases, though a minimal recovery may be expected.
Interestingly, we found that the MRI pattern and lesion extent may influence neurologic recovery below the injury level, but not around the injury level. In our study, we could not find any difference in neurologic level improvement between the edematous and mixed type groups, nor between groups I and II. We found that the more central area of the injured spinal cord was more involved, regardless of the MRI pattern and extent. This finding agreed with Silberstein and Hennessy's report.20 They studied sequential changes in the spinal cord using early and follow-up MRIs after spinal cord injury. In their study, 5 of the 6 patients with cord contusion and a patient with a cord haematoma subsequently developed small, well defined cysts at the central area of the original abnormal site. Of the 5 patients with cord edema, 3 developed focal areas of myelomalacia located at the center of the original MRI abnormality. We suspect that medially placed fibers were more involved at initial injury regardless of initial MRI findings, possibly resulting in no observable significant difference in neurologic improvement around the injured level. On the other hand, improvement of injuries to more laterally placed fibers, that is, recovery below the neurologic level, may depend on the MRI pattern and extent. We suspected another possible mechanism to explain this. The recovery around the injured level may not depend on the changes in spinal cord itself, but on changes in the surrounding anatomical structures, such as nerve root. However, the pathophysiological mechanism remains to be established. Further studies on these issues are required.
No purely hemorrhagic MRI patterns, as has been described by several authors,4,5 were found in the present study. All cord hemorrhages in the present study were associated with surrounding edema. Moreover, Selden et al.30 reported that MRIs of the human spinal cord immediately after injury showed spinal cord edema in 98% of patients. Also, Weirich,37 who described histologic changes in rat spinal cord injuries, reported that surrounding edema was found whenever hemorrhage was observed. They dropped a variety of masses on to rat spinal cords and observed microscopic histologic changes. The heavier mass was, the larger the hemorrhagic area was. They found that hemorrhage was followed by necrosis and eventual cavitation in the region involved. They also found that edema was a result of vascular endothelial cell lining disruption. This damaged endothelium then allowed the passage of protein-rich plasma filtrate into the extracellular space, creating a perivascualar edema. As a result, myelin degeneration accompanied cord edema as spinal cord flow failed. Considering the inflammatory process involved, we are of the opinion that a hemorrhagic process without surrounding edema is unlikely. This is borne out by the results of the present study, i.e. hemorrhage was always accompanied surrounding edema and no pure hemorrhagic types were observed. We suspect that this finding was due to the fact that MRIs were taken before edema had resolved.
This study has some limitations. First, the evaluators who assessed the initial neurologic status were different from those who evaluated it six months later. Inaccurate evaluation of neurologic status can be evoked. To minimize this error, the injured spinal cord neurologic status was evaluated using the most popular and well-known assessment. This assessment was based on the International Standards for the Neurologic and Functional Classification of Spinal Cord Injury Patients.12 In addition, the blind exam by another evaluator at follow-up can reduce error. Second, we did not consider other factors which may influence neurologic recovery. We analyzed the effect of age on motor and sensory recovery below the neurologic level according to the MRI findings using univariate ANOVA. But, the effect of age on motor recovery rate according to MRI pattern and extent was not significant. However, we can find effects of age on sensory recovery rate. So, further studies considering the many potential factors which can influence the recovery and the MRI findings will be needed.
In conclusion, early MRI after spinal cord injury may provide additional important information to help predict neurologic recovery. In patients with spinal cord injuries, early MRI findings, such as the presence of hemorrhage and the extent of the lesion, may be very useful for predicting the neurologic prognosis. Neurologic recovery below the injured level may be more predictable than recovery around the injured level.
Figures and Tables
Fig. 1
Comparison of recovery rate of motor ratio and sensory ratio between edematous type and mixed type. The recovery rate of motor ratio and sensory ratio was significantly higher in the edematous type than in the mixed type (p < 0.05).
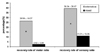
Fig. 2
Comparison of recovery rate of motor ratio and sensory ratio between groups. We classified as group I, when the alteration of intensity lies at one or two segments, and more than two segments as group II according to extent of lesion. The recovery rate of motor and sensory ratio was significantly higher in group I than in group II (p < 0.05).
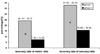
References
1. Go BK, DeVivo MJ, Richards JS. Stover SI, DeLisa JA, Whiteneck GG, editors. The epidemiology of spinal cord injury. Spinal cord Injury: clinical outcomes from the model systems. 1995. 1st ed. Gaithersberg: Aspen Publishers;21–51.
2. Kirshblum SC, O'Connor KC. Predicting neurologic recovery in traumatic cervical spinal cord injury. Arch Phys Med Rehabil. 1998. 79:1456–1466.
3. Flanders AE, Spettell CM, Tartaglino LM, Friedman DP, Herbison GJ. Forecasting motor recovery after cervical spinal cord injury: value of MR imaging. Radiology. 1996. 201:649–655.
4. York DH, Watts C, Raffensberger M, Spagnolia T, Joyce C. Utilization of somatosensory evoked cortical potentials in spinal cord injury. Prognostic limitations. Spine. 1983. 8:832–839.
5. Armin C. Significance of electrophysiological recording in predicting functional recovery of patients with spinal cord injury. Neurorehabil. 1998. 10:191–203.
6. Merton PA, Morton HB. Stimulation of the cerebral cortex in the intact human subject. Nature. 1980. 285:227.
7. Barker AT, Jalinous R, Freeston IL. Non-invasive magnetic stimulation of human motor cortex. Lancet. 1985. 1:1106–1107.
8. Rossini PM, Barker AT, Berardelli A, Caramia MD, Caruso G, Cracco RQ, et al. Non-invasive electrical and magnetic stimulation of the brain, spinal cord and roots: basic principles and procedures for routine clinical application. Report of an IFCN committee. Electroencephalogr Clin Neurophysiol. 1994. 91:79–92.
9. Post MJ, Green BA. The use of computed tomography in spinal trauma. Radiol Clin North Am. 1983. 21:327–375.
10. Brant-Zawadzki M, Miller EM, Federle MP. CT in the evaluation of spine trauma. Am J Roentgenol. 1981. 136:369–375.
11. Virapongse C, Kier EL. Metrizamide myelography in cervical spine trauma: a modified technique using lateral fluoroscopy. Radiology. 1982. 144:636–637.
12. Maynard FM Jr, Bracken MB, Creasey G, Ditunno JF Jr., Donovan WH, Ducker TB, et al. International standards for neurological and functional classification of spinal cord injury. American Spinal Injury Association. Spinal Cord. 1997. 35:266–274.
13. Blaustein DM, Zafonte R, Thomas D, Herbison GJ, Ditunno JF. Predicting recovery of motor complete quadriplegic patients. 24 hour v 72 hour motor index scores. Am J Phys Med Rehabil. 1993. 72:306–311.
14. Brown PJ, Marino RJ, Herbison GJ, Ditunno JF Jr. The 72-hour examination as a predictor of recovery in motor complete quadriplegia. Arch Phys Med Rehabil. 1991. 72:546–548.
15. Flanders AE, Schaefer DM, Doan HT, Mishkin MM, Gonzalez CF, Northrup BE. Acute cervical spine trauma: correlation of MR imaging findings with degree of neurologic deficit. Radiology. 1990. 177:25–33.
16. Kalfas I, Wilberger J, Goldberg A, Prostko ER. Magnetic resonance imaging in acute spinal cord trauma. Neurosurgery. 1988. 23:295–299.
17. Kulkarni MV, McArdle CB, Kopanicky D, Miner M, Cotler HB, Lee KF, et al. Acute spinal cord injury: MR imaging at 1.5 T. Radiology. 1987. 164:837–843.
18. Perovitch M, Wang H, Perl S. The evolution of neuroimaging of spinal cord injury patients over the last decade. Paraplegia. 1992. 30:39–42.
19. Schaefer DM, Flanders A, Northrup BE, Doan HT, Osterholm JL. Magnetic resonance imaging of acute cervical spine trauma. Correlation with severity of neurologic injury. Spine. 1989. 14:1090–1095.
20. Silberstein M, Hennessy O. Implications of focal spinal cord lesions following trauma: evaluation with magnetic resonance imaging. Paraplegia. 1993. 31:160–167.
21. Taneichi H, Abumi K, Kaneda K, Terae S. Monitoring the evolution of intramedullary lesions in cervical spinal cord injury. Qualitative and quantitative analysis with sequential MR imaging. Paraplegia. 1994. 32:9–18.
22. Bondurant FJ, Cotler HB, Kulkarni MV, McArdle CB, Harris JH Jr. Acute spinal cord injury. A study using physical examination and magnetic resonance imaging. Spine. 1990. 15:161–168.
23. Hayashi K, Yone K, Ito H, Yanase M, Sakou T. MRI findings in patients with a cervical spinal cord injury who do not show radiographic evidence of a fracture or dislocation. Paraplegia. 1995. 33:212–215.
24. Ishida Y, Tominaga T. Predictors of neurologic recovery in acute central cervical cord injury with only upper extremity impairment. Spine. 2002. 27:1652–1658.
25. Kulkarni MV, Bondurant FJ, Rose SL, Narayana PA. 1.5 tesla magnetic resonance imaging of acute spinal trauma. Radiographics. 1988. 8:1059–1082.
26. Marciello MA, Flanders AE, Herbison GJ, Schaefer DM, Friedman DP, Lane JI. Magnetic resonance imaging related to neurologic outcome in cervical spinal cord injury. Arch Phys Med Rehabil. 1993. 74:940–946.
27. Mascalchi M, Dal Pozzo G, Dini C, Zampa V, D'Andrea M, Mizzau M, et al. Acute spinal trauma: prognostic value of MRI appearances at 0.5 T. Clin Radiol. 1993. 48:100–108.
28. Ramon S, Dominguez R, Ramirez L, Paraira M, Olona M, Castello T, et al. Clinical and magnetic resonance imaging correlation in acute spinal cord injury. Spinal Cord. 1997. 35:664–673.
29. Schaefer DM, Flanders AE, Osterholm JL, Northrup BE. Prognostic significance of magnetic resonance imaging in the acute phase of cervical spine injury. J Neurosurg. 1992. 76:218–223.
30. Selden NR, Quint DJ, Patel N, d'Arcy HS, Papadopoulos SM. Emergency magnetic resonance imaging of cervical spinal cord injuries: clinical correlation and prognosis. Neurosurgery. 1999. 44:785–792.
31. Sett P, Crockard HA. The value of magnetic resonance imaging (MRI) in the follow-up management of spinal injury. Paraplegia. 1991. 29:396–410.
32. Tator CH. Biology of neurological recovery and functional restoration after spinal cord injury. Neurosurgery. 1998. 42:696–707.
33. Lucas JT, Ducker TB. Motor classification of spinal cord injuries with mobility, morbidity and recovery indices. Am Surg. 1979. 45:151–158.
34. Ohta K, Fujimura Y, Nakamura M, Watanabe M, Yato Y. Experimental study on MRI evaluation of the course of cervical spinal cord injury. Spinal Cord. 1999. 37:580–584.
35. Tator CH. Update on the pathophysiology and pathology of acute spinal cord injury. Brain Pathol. 1995. 5:407–413.
36. Wasenko JJ, Hochhauser L, Holsapple JW, Poe LB, Albanese SA, Cacayorin ED. MR of post traumatic spinal cord lesions. Unexpected improvement of hemorrhagic lesions. Clin Imaging. 1997. 21:246–251.
37. Weirich SD, Cotler HB, Narayana PA, Hazle JD, Jackson EF, Coupe KJ, et al. Histopathologic correlation of magnetic resonance imaging signal patterns in a spinal cord injury model. Spine. 1990. 15:630–638.