Abstract
Endothelial progenitor cells (EPCs) have been reported to possess the capacity to colonize vascular grafts and hold promise for therapeutic neovascularization. However, limited quantities of EPCs have been the major factor impeding effective research on vasculoangiogenesis. In this study, cytokine and culture conditions necessary for the provision of large quantities of endothelial cells (ECs) were investigated. Cord blood was collected from 18 normal full-term deliveries and CD34+ cells were isolated by MACS system (Miltenyi Biotech, Bergish-Gladbach, Germany). To evaluate the effect of cytokines, CD34+ cells were cultured with various cytokine combinations, such as stem cell factor (SCF), flt3-ligand (FL), and thrombopoietin (TPO) with vascular endothelial growth factor (VEGF), interleukin-1β, fibroblast growth factor-basic (FGF-b) as basic cytokines. The quantities of non-adherent and adherent cells were the greatest with SCF, FL and TPO. The addition of TPO to all other cytokines significantly increased the number of non-adherent and adherent cells (p < 0.05, Wilcoxon rank sum test). After four weeks of culture, adherent cells expressed endothelial specific markers such as KDR, CD31 and CD62E. Typical morphology of ECs was observed during culture, such as cord-like structure and cobblestone appearance, suggesting that the adherent cells were consistent with ECs. In this study, the experimental conditions that optimize the production of ECs for therapeutic neovascularization were described. And it was possibly suggested that TPO plays a major role in differentiation from EPCs to ECs.
Though neovascularization began as a concept described by those investigating the pathogenesis of diseases such as diabetic retinopathy and rheumatoid arthritis, it is now widely studied by people investigating tumor metastasis and treatment of ischemic diseases.1-3
Endothelial progenitor cells (EPCs) are known to propagate the neovascularization process by promoting vasculogenesis and angiogenesis. EPCs have properties similar to those of embryonic angioblasts, in that they have the capacity to circulate, proliferate, and differentiate into mature endothelial cells. However, they lack the ability to form luminal characteristics of vasculogenesis and do not possess mature endothelial markers.4 In fact, these cells share similar surface markers with hematopoietic stem cells (HSCs), such as CD34, Flk-1/KDR, Tie-1 and Tie-2 etc.4-6
Studies of EPCs have been limited due to the inability to obtain enough EPCs. HSCs make up less than 1% of bone marrow cells and even a smaller proportion of peripheral blood mononuclear cells, and EPCs comprise less than 1% of HSCs.7,8 Therefore, techniques to isolate and culture the EPCs must be developed before they can be used in the study of neovascularization and in therapeutic protocols.
Asahara et al.5 first described a method to isolate EPCs from human peripheral blood. Numerous studies that characterized EPCs and attempted to optimize culture conditions with various growth factors and cytokines followed.3,9,10 However, the isolation, identification, and characterization of EPCs have been difficult due to the absence of specific endothelial markers or functional assays that differentiate EPCs from HSCs.4,9
The purpose of this study was to isolate EPCs from cord blood and culture them in vitro to observe the effects of various cytokines, such as VEGF, IL-1β, FGF-b, SCF, FL and TPO on the development of EPCs to ECs, and finally, to establish the technical and theoretical background to allow the therapeutic use of EPCs.
Umbilical cord blood was obtained from 18 normal full-term deliveries in Yonsei University Medical Center and was placed into sterile blood collection bags (Green Cross, Seoul, Korea) containing 25 mL CPDA-1. Cord blood collection was approved by the hospital ethics committee and informed consent of the mother was obtained.
Unseparated cord blood was diluted 1 : 4 with Hank's Balanced Salt Solution (HBSS, Gibco-BRL, Grand Island, NY, USA), and mononuclear cells (MNCs) were isolated using Ficoll-Hypaque (density, 1.077; Pharmacia Biotech, Uppsala, Sweden) density centrifugation. MNCs recovered from the interface were washed twice with HBSS and enumerated using hemocytometer. Thereafter, they were resuspended in phosphate-buffered saline (PBS), pH 7.4 (Sigma Chemical Co., St. Louis, MO, USA). Uniform, magnetic beads (Miltenyi Biotech, Bergish-Gladbach, Germany), which were coated with a monoclonal antibody specific for human CD34, were used to separate CD34+ cells. MNCs were resuspended to a concentration of 1 × 108 cells in 300 µL PBS, 5 mM EDTA. These cells were incubated with beads at ratios that 100 µL beads per 108 cells for 15 minutes at 4℃ and processed through a MACS magnetic separation column (Miltenyi Biotech) to obtain purified CD34+ cells. Selected CD34+ cells were detached from the magnetic beads using the plunger and resuspended in Iscove's modified Dulbecco's medium (IMDM).
For identification of HSCs, purified MNCs were incubated with FITC-labeled anti-CD34 antibody (Becton Dickinson, San Jose, CA, USA), PE-labeled anti-CD38 (Becton Dickinson) and anti-CD117 (Pharmingen, San Diego, CA, USA) antibodies. For EPCs, KDR-FITC (Sigma Chemical Co.) and AC133-PE (Miltenyi Biotech), CD31-FITC (Pharmingen) and CD62E-PE (Pharmingen), CD34-FITC and CXCR4-PE (Pharmingen) were used. The percentage of positive cells was analyzed by two-color flow cytometry (FACScan, Becton Dickinson) and compared to IgG isotype control.
CD34+ cells suspended in IMDM at 2 × 105cells/mL were placed for culture into 24- or 96-well microplates, that had been coated with 1 µg/mL fibronectin, by 1 mL or 100 µL, respectively. The media was supplemented with various cytokine combinations, that is, 10 ng/mL of stem cell factor (SCF), 10 ng/mL flt3-ligand (FL), and 5ng/mL thrombopoietin (TPO) with 10 ng/mL vascular endothelial growth factor (VEGF), 10 ng/mL IL-1β, 5 ng/mL fibroblast growth factor-basic (FGF-b) as the basic combination. Also, the culture was performed in the presence or absence of TPO with all other cytokines (VEGF, IL-1β, FGF-b, SCF and FL) for 6 days to evaluate the effect of TPO on EC development. On the 6th day of culture, non-adherent cells were all removed, and the number of non-adherent and adherent cells obtained by culture with the different cytokine combinations was compared. At this time, non-adherent cells were collected after gentle pipeting with PBS and adherent cells were collected by treating PBS containing 5 mM EDTA and harsh pipeting. Thereafter, the remaining adherent cells were cultured in the presence of VEGF, IL-1β and FGF-b, that is, only under the basic combination. Culture media was changed every three days with fresh media supplemented with the basic cytokines. On the 12th day of culture, the number of adherent cells per mm2 was counted using hemocytometer. During the culture period, cells were recovered when they reached 80% confluence, washed and enumerated, then recultured in a 6-well plate or a 75 cm2 flask.
The morphology of adherent cells was observed every week of the culture period using an inverted microscope (Olympus Optical Co., LTD., Tokyo, Japan). After 4 weeks of culture, adherent cells were recovered and analyzed for the expression of EC markers by flow cytometry.
The average number of MNCs isolated from each cord blood by Ficoll gradient centrifugation was 1.34 (±0.43) × 108 and the average number of CD34+ cells isolated by the MACS system was 4.22 (±2.46)×105. Analysis by two-color flow cytometry found that, 0.65% of total MNCs were CD34 positive. The recovery rate of CD34+ cells by the MACS system was 84.04%. CD34+/CD38-cells, considered to be primitive stem cells, made up 0.05% of MNCs and 7.44% of CD34+ cells. AC133+/KDR+ cells, considered to be EPCs, were 0.15% of MNCs and 1.73% of CD34+ cells (Table 1).
The average number of non-adherent cells was expanded to 5.9×104/mL after 6 days when CD34+ cells were cultured only with the basic cytokines (VEGF+IL-1β +FGF-b), that is, this represents a 2.9-fold increase during the culture period. The non-adherent cells were expanded to 5.4-, 3.7- and 4.2-fold when supplemented with SCF, FL and TPO, respectively, in addition to the basic cytokine combination. They were expanded to 7.1-fold when supplemented with SCF+FL, 5.7-fold with FL+TPO and 8.3-fold with SCF+TPO. When supplemented with SCF+FL+TPO, the suspension cells were expanded up to 21.1×104/mL, or 10.7-fold after culture.
Adherent cells were counted at the 12th day of culture. The average number of adherent cells was 5.6/mm2 when cultured with the basic cytokines (n=7). Adherent cell counts were 6.0, 10.7 and 15.9 cells/mm2 when supplemented with SCF, FL and TPO, respectively, in addition to the basic combination. Adherent cell counts were 23.6, 27.1 and 37.9 cells/mm2 when supplemented with SCF+FL, FL+TPO and SCF+TPO, respectively. The number of adherent cells was the greatest (65.4 cells/mm2 when the media with basic cytokines was supplemented with SCF+FL+TPO (Table 2).
To observe the effect of TPO on the proliferation of EPCs, 2×105 CD34+ cells were cultured in a 24-well microplate for 6 days in the presence of TPO plus all the other cytokines. As a result of the addition of TPO, the number of non-adherent cells increased significantly (p=0.0341, Wilcoxon rank sum test) (Fig. 1).
KDR, CD31 and CD62E were found to be expressed on 13.3%, 14.7% and 18.4% of the adherent cells cultured for 4 weeks. However, the frequencies of adherent cells expressing CD34 and AC133 were only 0.4% and 0.0%, compared to 84.0% and 82.3% before culture. The expression of CXCR-4 (CD183) on adherent cells after 4 weeks of culture was also 0.0% (Fig. 2).
Initially, most cells were small and round, but within 24 hours of culture, the cells promptly attached to the wells and became spindle shaped. At about the 3rd day of culture, the cells formed several cell clusters. Cord-like structures resembling the first stages of vasculogenesis appeared as early as 7 days of culture and the characteristic endothelial cobblestone colonies were observed at about 4 weeks of culture (Fig. 3).
EPCs, the cells initiating neovascularization, express similar endothelial-specific markers as mature ECs, including flk-1/KDR (VEGFR-2), Tie-1, Tie-2 and VE-cadherin. Furthermore, EPCs share similar markers with HSC, such as VEGFR-1, CD34, CD31 (platelet endothelial cell adhesion molecule, PECAM), Tie-1, Tie-2 and von Willebrand factor.11-13 However, the isolation and characterization of EPCs from blood have been difficult because of the absence of specific phenotypic markers and functional assays to define them.4
Asahara et al.5 first isolated EPCs from peripheral blood using CD34 and KDR as markers and they found that 10.8 ± 0.9% of peripheral blood were EPCs, coexpressing CD34 and KDR. On the other hand, Peichev et al.4 identified EPCs from cord blood, fetal liver and G-CSF mobilized peripheral blood using AC133 and KDR and found that 1.4 ± 0.5% of cord blood, 1.2 ± 0.3% of fetal liver and 2.0 ± 0.5% of G-CSF mobilized peripheral blood were EPCs, which was much less than that of Asahara et al.5 Peichey et al.4 believed that this difference may be due to the use of a highly specific monoclonal antibody to the extracellular domain of KDR in their study, allowing for the more accurate measurement of EPCs, as compared to the polyclonal antibody directed to the intracellular domain of KDR used by Asahara et al.5 In our study, we isolated CD34+ cells from cord blood and identified EPCs using monoclonal antibodies to the AC133 and KDR, and found that 1.73 ± 0.7% of the cells coexpressing both markers. This result was similar to that of Peichev's.4
AC133, a new hematopoietic stem cell marker, identifies a structurally novel 5-transmembrane antigen that is expressed on a large subset of EPCs and HSCs, but not on the mature endothelium.14-16 Peichev et al.4 demonstrated that CD34+ cells coexpressing AC133 and KDR are functionally non-adherent endothelial cells that have the capacity to migrate and differentiate into mature adherent endothelial cells expressing KDR, but not AC133. Our study also found that cultured adherent cells expressed KDR (13.3%), but not AC133 (0.0%), also suggesting that non-adherent EPCs coexpressing AC133 and KDR had differentiated into mature adherent ECs.
In our study, EPCs were estimated to make up 1.73% of CD34+ cells in cord blood, that is, 7.3 × 103 EPC cells per cord blood collection. Since the number of ECs in each cord blood unit was so low it was impossible to characterize the EPCs prior to culture because at least 1 × 106 cells were needed to analyze their surface markers by flow cytometry.17 Accordingly, we induced the ex vivoexpansion and differentiation of EPCs by culturing them with various combinations of SCF, FL, TPO with VEGF, IL-1β, FGF-b as the basic cytokines. At the same time, we analyzed the optimal cytokine conditions required to induce the ex vivo expansion of EPCs. We found that the number of non-adherent cells was the greatest when the media supplemented with SCF, FL and TPO. Interestingly, however, non-adherent cells coexpressing CD34 and KDR were not detected by flow cytometry after 6 days of culture (data not shown). It was thought that HSCs containing EPCs had already begun to differentiate to their respective hematopoietic cell lineages, and this differentiation was accelerated by the cytokines added during culture. Therefore, to find the optimal cytokine conditions for the differentiation of EPCs to ECs, we made use of the cell adhesiveness that characterizes ECs. The number of adherent cells was also the greatest when the media was supplemented with SCF, FL and TPO.
TPO is known to stimulate VEGF release from hematopoietic progenitors and each cell lineage.18 In our study, the number of non-adherent cells was compared after cultured with or without TPO in the presence of all other cytokines and we found that TPO contributed significantly to the self-renewal and differentiation of EPCs.
Asahara et al.5 compared the expression of leukocyte and EC markers between CD34+ cells isolated from fresh peripheral blood (MBCD34+) and adherent cells produced by the culture of MBCD34+ on fibronectin for 7 days (ATCD34+). In freshly isolated MBCD34+ cells, 15.7% were CD34+, 94.1% were CD45+, 51.3% were CD31+, 27.6% were KDR+, 30.3% were Tie-2+ and 0.6% were CD62E+. In ATCD34+ cells, 32.8% were CD34+, 27.2% were CD45+, 71.5% were CD31+, 35.9% were KDR+, 54.6% were Tie-2+ and 9.0% were CD62E+. Peichev et al.4 compared the expression of several HSC and EC markers by EPC, EC and IL-1β activated EC. They found that KDR, VE-cadherin, CD31 and CD117 were expressed by both EPCs and ECs, but AC133 was expressed only by EPCs. The expression of most markers by IL-β activated ECs was similar to ECs, but CD62E was expressed by IL-β activated ECs but not by EPCs and ECs.
CXCR-4, the natural receptor for the chemokine SDF-1, is expressed on endothelial cells. CXCR-4 knock-out mice are not viable and have multiple defects, including angiogenesis, suggesting that this receptor may play a role in vascular remodeling and, possibly, trafficking of circulating endothelial progenitor cells.4
In our study, adherent cells cultured for 4 weeks expressed EC specific markers such as KDR, CD31 and CD62E though the expression was weak, but did not express CD34, CD117, AC133 and CXCR4. EC markers may have been suppressed secondary to the effect of cytokines used in culture. It is also possible that cell surface antigens were destroyed by the cell scraper used to detach adherent cells for flow cytometric analysis.
The adherent cells not only expressed typical EC antigens, but during the culture period they showed the typical morphologies of endothelial cells, such as the cord-like structure and cobblestone appearance. This provides further evidence that the adherent cells were consistent with ECs.4-6
In conclusion, we describe experimental conditions that optimize the production of ECs and that could be used to obtain large numbers of ECs for therapeutic neovascularization. We also characterized the EPCs in CD34+ cells isolated from cord blood and confirmed that TPO plays a major role in the differentiation from EPCs to ECs.
Figures and Tables
Fig. 1
The numbers of non-adherent cells obtained from cultures preformed with or without TPO in the presence of VEGF, IL-1β, FGF-b, SCF and FL in 24-well microplates (n=9). The line represents the mean values. The addition of TPO significantly increased the numbers of non-adherent cells (p < 0.05, Wilcoxon rank sum test).
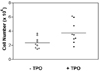
Fig. 2
Flow cytometric analysis of endothelial cells obtained from CD34+ cells after 4 weeks of culture. The dark line identifies the cells labeled with isotype control antibody and the faint line identifies the cells labeled with antibodies specific for the surface markers. Cultured cells were positive for KDR, CD31 and CD62E, but negative for CD34, CD117, AC133 and CXCR4 (cut off, 10%).
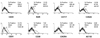
Fig. 3
Morphology of cord blood derived adherent cells at days 7(A), 14(B), 21(C), and 28(D) of in vitro culture. A) and B) Cord-like structures resembling the first stages of vasculogenesis; C) and D) Characteristic endothelial cobblestone colonies (Unstained; magnification, ×100).
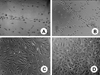
References
1. Denekamp J. The current status of targeting tumor vasculature as a means of cancer therapy: an overview. Int J Radiat Biol. 1991. 60:401–408.
2. Kim KJ, Li B, Winer J. Inhibition of vascular endothelial growth factor-induced angiogenesis suppresses tumor growth in vivo. Nature. 1993. 362:841–844.
3. Isner JM, Asahara T. Angiogenesis and vasculogenesis as therapeutic strategies for postnatal neovascularization. J Clin Invest. 1999. 103:1231–1236.
4. Peichev M, Naiyer AJ, Pereira D, Zhu Z, Lane WJ, Williams M, et al. Expression of VEGFR-2 and AC133 by circulating human CD34+ cells identifies a population of functional endothelial precursors. Blood. 2000. 95:952–958.
5. Asahara T, Murohara T, Sullivan A, Silver M, van der Zee R, Li T, et al. Isolation of putative progenitor endothelial cells for angiogenesis. Science. 1997. 295:964–967.
6. Boyer M, Townsend LE, Vogel LM, Falk J, Reitz-Vick D, Trevor KT, et al. Isolation of endothelial cells and their progenitor cells from human peripheral blood. J Vasc Surg. 2000. 31:181–189.
7. Davey FR, Hutchison RE. Henry JB, Davey FR, editors. Hematopoiesis. Clinical diagnosis and management by laboratory methods. 2001. 20th ed. Philadelphia, PA: Saunders;520–541.
8. Siena S, Bregni M, Brando B, Ravagnani F, Bonadonna G, Gianni AM. Circulation of CD34+ hematopoietic stem cells in the peripheral blood of high-dose cyclophosphamide-treated patients: Enhancement by intravenous recombinant human granulocyte-macrophage colony-stimulating factor. Blood. 1989. 74:1905–1914.
9. Shi Q, Rafii S, Wu MH, Wijelath ES, Yu C, Ishida A, et al. Evidence for circulating bone marrow-derived endothelial cells. Blood. 1998. 92:362–367.
10. Choi K, Kennedy M, Kararov A, Papadimitriou JC, Keller G. A common precursor for hematopoietic and endothelial cells. Development. 1998. 125:725–732.
11. Flamme I, Breier G, Risau W. Vascular endothelial growth factor (VEGF) and VEGF receptor-2 (flk-1) are expressed during vasculogenesis and vascular differentiation in the quail embryo. Dev Biol. 1995. 169:699–712.
12. Vittet D, Prandini MH, Berthier R, Schweitzer A, Martin-Sisteron H, Uzan G, et al. Embryonic stem cells differentiation in vitro to endothelial cells through successive maturation steps. Blood. 1996. 88:3424–3431.
13. Ali J, Liao F, Martens E, Muller WA. Vascular endothelial cadherin (VE-cadherin): cloning and role in endothelial cell-cell adhesion. Microcirculation. 1997. 4:267–277.
14. Miraglia S, Godfrey W, Yin AH, Atkins K, Warnke R, Holden JT, et al. A novel five-transmembrane haematopoietic stem cell antigen: isolation, characterization, and molecular cloning. Blood. 1997. 90:5013–5021.
15. Yin AH, Miraglia S, Zanjani ED, Almeida-Porada G, Ogawa M, Leary AG, et al. AC133, a novel marker for human hematopoietic stem and progenitor cells. Blood. 1997. 90:5002–5012.
16. Gehling UM, Ergun S, Schumacher U, Wagener C, Pantel K, Otte M, et al. In vitro differentiation of endothelial cells from AC133-positive progenitor cells. Blood. 2000. 95:3106–3112.
17. Feldkamp CS, Carey JL. Rose NR, de Macario EC, editors. Standardization of immunoassay methodologies. Manual of clinical laboratory immunology. 1997. 5th ed. Washington D.C.: Am Soc Microbiol;1168–1179.
18. Bobik R, Hong Y, Breier G, Martin JF, Erusallimsky JD. Thrombopoietin stimulates VEGF release from c-Mpl-expressing cell lines and haematopoietic progenitors. FEBS Lett. 1998. 423:10–14.