Abstract
Objective
To devise a new method to measure the amount of soft tissue in pulmonary ground-glass opacity nodules, and to compare the use of this method with a previous volumetric measurement method by use of a phantom study.
Materials and Methods
Phantom nodules were prepared with material from fixed normal swine lung. Forty nodules, each with a diameter of 10 mm, were made with a variable mean attenuation. The reference-standard amount of soft tissue in the nodules was obtained by dividing the weight by the specific gravity. The imaging data on the phantom nodules were acquired with the use of a 16-channel multidetector CT scanner. The CT-measured amount of soft tissue of the nodules was calculated as follows: soft tissue amount = volume × (1 + mean attenuation value / 1,000). The relative percentage error (RPE) between the CT-measured amount of the soft tissue and the reference-standard amount of the soft tissue was also measured. The RPEs determined with use of the new method were compared with the RPEs determined with the current volumetric measurement method by the use of the paired t test.
Results
The CT-measured amount of soft tissue showed a strong correlation with the reference-standard amount of soft tissue (R2 = 0.996, p < 0.01). The mean RPE of the CT-measured amount of soft tissue in the nodules was -7.79 ± 1.88%. The mean RPE of the CT-measured volume was 114.78 ± 51.02%,which was significantly greater than the RPE of the CT-measured amount of soft tissue (p < 0.01).
Since the introduction of low-dose CT for mass screening to diagnose lung cancer, an increasing number of nodules with ground-glass opacity (GGO) have been detected (1, 2).
Software that allows one to measure the dimensions of non-spherical lesions rapidly on each CT section and to compute automatically and easily the cross-sectional area and the volume is now widely available (3-6). Accurate volumetry is essential for the accurate calculation of the volume doubling time of nodules, which is one of the most important clues for differentiating between a malignant and benign nodule. There are few algorithms, however, for the determination of a volumetric measurement or the assessment of a temporal change in the amount of soft tissue of nodules with GGO (7-10). The volume of GGO nodules is more difficult to measure due to the lower contrast of the nodules with the lung parenchyma as compared to solid nodules (11).
If the mean attenuation value of a nodule changes from -700 HU to -300 HU without any change in its diameter, the amount of soft tissue in that nodule will double or triple. However, the volume of the nodule will not change or will change minimally, as determined with the use of current volumetric measurement methods. Current methods of measuring the nodule volume with the use of bi-dimensional and three-dimensional CT do not reflect the mean attenuation value of nodules with GGO. Therefore, a new method of measuring the amount of soft tissue in GGO nodules is required that will reflect the mean attenuation value of the nodules.
The purpose of this study was to demonstrate the problems with the use of the current volumetric measurement methods and to devise a new method for measuring the amount of soft tissue in pulmonary GGO nodules by use of a phantom study.
The nodules with GGO that were used in this study were made from material from swine lung tissue. After a pig was sacrificed, the thorax was opened and the main pulmonary artery was ligated. The lungs were then removed from the thorax. Lung fixation and inflation were performed using the methods of Markarian and Dailey (12). The lungs were inflated with a fixative composed of the following compounds: 50% polyethylene glycol 400, 25% of 95% ethyl alcohol, 10% of 37% formaldehyde and 15% water by volume. This fluid was instilled into the lungs through the trachea so that the lung would be inflated incompletely. The trachea was then clamped to prevent loss of the fixative, and the specimen was kept in a container of the same fixative for three days. The lungs were dried in room air for more than four weeks.
The GGO nodules were then sculptured out of this lung tissue. Forty nodules were prepared, each with a diameter of approximately, or greater than, 10 mm (Fig. 1A). These nodules were compressible to some degree in the 10 mm diameter Styrofoam wells. It was assumed that the amount of soft tissue would not change from compression. The mean attenuation value of the nodules was approximately from-350 HU to -750 HU.
Styrofoam matrix material (with a mean attenuation value of-986 HU) was used as a phantom of the surrounding pulmonary parenchyma. Forty wells, each with a diameter of 10 mm, were drilled into a 5 cm-thick Styrofoam plate (Fig. 1B).
To obtain the reference-standard amount of soft tissue (STARS), the nodules were weighed on a scale with a precision of 0.1 mg. The weight measurement was repeated three times and was averaged to calculate the values used in the analysis. The STARS of a nodule was then calculated by dividing the weight by the specific gravity of the fixed pulmonary parenchymal tissue in the same condition as the phantom nodules. After 20-30 g of the fixed lung tissue was weighed on a precision scale, the lung tissue was compressed and squeezed vigorously with a stick in a 100 mL cylinder to remove the entrapped air in the tissue, and was soaked overnight in water. The volume was measured three times using displacement of the water. Then, the mean specific gravity was determined by dividing the weight by the volume.
The imaging data on the phantom nodules were acquired with the use of a 16-channel multidetector CT scanner (Mx 8000; Philips Medical System, Cleveland, OH). The imaging parameters were as follows: spiral mode, 16 × 0.75 mm collimation, 120 kVp, 200 mA, 1 mm thickness, and 0.75 sec rotation time. The images were reconstructed at 1 mm intervals using a soft tissue algorithm (reconstruction kernel standard C) and an 18 cm field of view (Fig. 2).
Initially, the volume of the nodules was measured with commercially available 3-D software (Rapidia; Infinitt, Seoul, Korea). This program allows a user to segment the nodule and to measure the volume of the nodules at a user-defined attenuation threshold. Regions of interest (ROI) were manually placed on each nodule, and the nodules were segmented automatically on the gray-level threshold.
The CT-measured volume of the nodules (VCT) was defined as the segmented nodule volume with a variety of thresholds. The mean attenuation value of the segmented nodules was then calculated. It was assumed that the mean CT attenuation value of a given nodule volume correlated with the proportion of the gas and tissue within the nodule. Considering that the attenuation value of air is -1,000 HU and the attenuation value of water is 0 HU, it is possible to compute the amount of gas and tissue present in the ROI using the following equation: Volumetissue = Volumegas + tissue [1 + HU / 1,000 ]. According to previously described analyses (13-15), this equation was applied for the measurement of the air volume in the lung with acute lung injury. It was assumed that this method could also be used to measure the amount of soft tissue in pulmonary GGO nodules. The amount of soft tissue in each nodule of interest was measured using the following equation: the amount of soft tissue measured by CT (STACT) = CT-measured volume (VCT) × (1 + mean attenuation value / 1,000). The unit of the STARS, the STACT and the VCT is mm3.
The volume of the nodules was measured using the fixed threshold method. Three lower thresholds (-900 HU, -800 HU and -700 HU) and an upper threshold (200 HU) were used. The accuracy of the lower threshold value was compared, and the most accurate value was selected as the lower threshold.
To evaluate the measurement accuracy, the relative percent error (RPE) was used (16, 17). The RPE is the percent deviation of a measured value from the true value. The RPE of VCT or STACT is defined as 100 × (VCT or STACT - STARS) / STARS, in which VCT is the CT-measured volume of each nodule and STACT and STARS are the CT-measured amount of soft tissue and the reference-standard amount of soft tissue, respectively, in each nodule. All of the measurement data sets were tested for normality using the Kolmogorov-Smirnov test.
To assess the difference between the RPEs with the use of the two measurement methods and among different lower thresholds, the paired t test and the Friedman test (a non-parametric repeated measure one-way analysis of variance) were performed. The Pearson correlation coefficient (R) between the STACT of each lower threshold level and the STARS was calculated to compare the measurement accuracy of the three lower threshold levels. Linear regression analysis was used to assess the association between the STACT and the STARS of the nodules and between the VCT and the STARS at the -900 HU lower threshold level as the measurement was most accurate at that threshold level. Regression between the RPE of the STACT and the mean attenuation value was not analyzed as the STACT already included the mean attenuation value, so that a separate regression would have been meaningless.
A p value of less than 0.05 was considered statistically significant and was subject to further analysis with multiple posttest comparisons. Statistical analyses were performed using SPSS 12.0 for Windows (SPSS, Chicago, IL).
The specific gravity of the fixed lung tissue was 0.9942. The STARS in the nodules ranged from 307.38 mm3 to 754.68 mm3.
The RPEs of STACT of the nodules ranged from -12.2% to -3.32%. The STACT was smaller than the STARS in all of the nodules. The mean RPE of the STACT was -7.79 ± 1.88% at the lower threshold level of -900 HU, -13.4 ± 5.04% at -800 HU and -22.1 ± 11.46% at -700 HU (Table 1). The RPE of the STACT at the lower threshold level of -900 HU was significantly smaller than that at -800 HU and at -700 HU (p < 0.01). The mean RPE of the VCT was 114.8±51.02%. The STACT was much more accurate than the VCT (p < 0.01) (Table 1).
The Pearson correlation coefficients determined between the STACT and the STARS were 0.998 for the -900 HU threshold level, 0.996 for -800 HU and 0.990 for -700 HU (Table 1). Linear regression of the STARS on the STACT of the nodules showed the strongest correlation at the -900 HU threshold level (R2 = 0.996, p < 0.01) (Fig. 3A). The resulting linear equation was as follows: STARS = 1.036 × STACT + 21.199. Regression of the STARS on the VCT of the nodules at the -900 HU lower threshold level showed a poor correlation (R2 = 0.294, p < 0.01) (Fig. 3B). Regression of the RPE of the VCT at the -900 HU threshold level on the mean attenuation value showed a strong negative correlation (R2 = 0.941, p < 0.01) (Fig. 3C).
Volumetric assessment of small pulmonary nodules to estimate tumor growth is gaining increased attention in radiological practice, particularly in conjunction with ongoing lung cancer screening trials (18). Recent advances in imaging technology enable precise 3-D volumetric measurement of tumors, and the use of advanced technology allows more accurate assessments of tumor burden than the use of traditional unidimensional or bidimensional measurement methods (3, 16, 19, 20).
There is, however, no suitable volumetric measurement method for GGO nodules. Ko et al. (11) reported that a higher precision in volume measurement is associated with the use of a partial volume method and a predetermined pure-nodule-attenuation high-frequency algorithm. The investigators used simulated GGO nodules with approximately -360 HU, which were composed of a plastic created by blending epoxy resins and urethanes. The absolute errors of the GGO nodules were observed as higher than the absolute errors of the solid-attenuation nodules. The investigators suggested that any difference between methods of measuring GGO nodule volume was related to the reduced contrast between the GGO nodules and the lung parenchyma. However, the investigators did not consider the effect of the mean attenuation of the nodules on the measured volume.
The amount of soft tissue in a attenuation of -300 HU is different from the amount in a nodule with a mean attenuation of -700 HU. If two nodules have the same diameter, the measured volume of the GGO nodule with a mean attenuation of -300 HU would be the same as that of the nodule with a mean attenuation of -700 HU using current volumetric measurement methods. The amount of tissue in a GGO nodule should increase based on the mean attenuation value of the nodules. Therefore, the mean attenuation value of a nodule should be considered when measuring the volume or amount of tissue in nodules.
In this study, the RPE of the STACT of the nodules ranged from -12.21% to -3.32%. There was a tendency towards improved accuracy with an increase in the mean attenuation value of the nodules. However, the discrepancy among the nodules with different mean attenuation values was not significant. The volume of a GGO nodule, measured with commercially available 3-D software using the threshold method was much greater than the STARS. The RPEs of the VCT of the nodules ranged from 42% to 258%. The RPE of the VCT showed a very strong negative correlation with the mean attenuation value. This study showed that for a lower mean attenuation value of a nodule, a greater RPE of the VCT will be found. This finding is explained by the smaller amount of tissue in a nodule with a lower mean attenuation value. The STACT represents the amount of soft tissue in GGO nodules much more accurately than the VCT.
In this study, the linear regression equation of the STARS on the STACT was as follows: STARS = 1.036 STACT + 21.199 = 1.036 × VCT × (1 + mean attenuation value / 1,000) + 21.199. The slope was near 1 and the R2 (R2 = 0.996, p < 0.01) was very high. These results show that the STACT has a very strong linear correlation with the STARS. The intercept 21.199 indicates that the STACT was somewhat smaller than the STARS. Based on this result, the STARS could be calculated from the STACT, which could be obtained from the VCT and the mean attenuation value of a nodule.
To make artificial GGO nodules, fixed swine lungs were used. Artificial nodules made by blending epoxy resins or urethanes could not be utilized to represent true GGO nodules.
For the lower threshold in this phantom study, -900 HU was used, as the RPE was smaller in proportion to a decrease in the lower threshold. The RPE of the STACT with a -900 HU threshold was significantly smaller than the RPE of an STACT with -800 HU and -700 HU thresholds (p < 0.01). This finding indicates that the volume measured at the -900 HU threshold was the most accurate. When -800 HU or -700 HU was used as the lower threshold, some portions of the nodules were not included in the volume measurement, especially for the nodules with lower mean attenuation values, and the measured mean attenuation value increased.
Application of -900 HU as the lower threshold was possible in the phantom study. In this study, the margin of the nodule could be defined clearly. In addition, the measurement of the volume was not disturbed by a surrounding parenchymal phantom. However, -900 HU could not be used as a lower threshold level in the volumetry of real GGO nodules in patient studies.
The VCT and the STACT measured by two researchers were the same, regardless of the mean attenuation value or diameter of the nodules. The VCT and the STACT measured at two different times were also the same. There was no inter- and intra-observer discrepancy. CT-measurement of the amount of soft tissue in a nodule is an objective method.
This study has several limitations. For one, a chest phantom was not used in the study. Instead, Styrofoam was used as the surrounding lung parenchyma. The results of prior studies on nodule densitometry in phantom models have demonstrated that the mean attenuation value of a nodule is lower in a tissue phantom or a chest phantom. It is uncertain how different the mean attenuation value of GGO nodules within a tissue phantom or a chest phantom is from mean attenuation value of GGO nodules within Styrofoam. The effect of the diameter of the GGO nodules on the accuracy of measurement was not evaluated, which could be another limitation of this study. A further study is needed to evaluate the accuracy of volumetric measurement for smaller GGO nodules with diameters of less than 10 mm.
In summary, the amount of soft tissue in GGO nodules measured by CT is well correlated with the reference standard amount of soft tissue in GGO nodules, and is much more accurate than as determined with the use of the previous volumetric measurement method.
References
1. Diederich S, Wormanns D, Semik M, Thomas M, Lenzen H, Roos N, et al. Screening for early lung cancer with low-dose spiral CT: prevalence in 817 asymptomatic smokers. Radiology. 2002; 222:773–781. PMID: 11867800.


2. Henschke CI, Yankelevitz DF, Mirtcheva R, McGuinness G, McCauley D, Miettinen OS. CT screening for lung cancer: frequency and significance of part-solid and nonsolid nodules. AJR Am J Roentgenol. 2002; 178:1053–1057. PMID: 11959700.
3. Yankelevitz DF, Reeves AP, Kostis WJ, Zhao B, Henschke CI. Small pulmonary nodules: volumetrically determined growth rates based on CT evaluation. Radiology. 2000; 217:251–256. PMID: 11012453.


4. Wormanns D, Kohl G, Klotz E, Marheine A, Beyer F, Heindel W, et al. Volumetric measurements of pulmonary nodules at multi-row detector CT: in vivo reproducibility. Eur Radiol. 2004; 14:86–92. PMID: 14615902.


5. Revel M-P, Lefort C, Bissery A, Bienvenu M, Aycard L, Chatellier G, et al. Pulmonary nodules: preliminary experience with three-dimensional evaluation. Radiology. 2004; 231:459–466. PMID: 15128991.


6. Goo JM, Lee JW, Lee HJ, Kim S, Kim JH, Im JG. Automatic lung nodule detection at low-dose CT: preliminary experience. Korean J Radiol. 2003; 4:211–216. PMID: 14726637.
7. Aoki T, Nakata H, Watanabe H, Nakamura K, Kasai T, Hashimoto H, et al. Evolution of peripheral lung adenocarcinomas: CT findings correlated with histology and tumor doubling time. AJR Am J Roentgenol. 2000; 174:763–768. PMID: 10701622.
8. Takashima S, Maruyama Y, Hasegawa M, Yamanda T, Honda T, Kadoya M, et al. CT findings and progression of small peripheral lung neoplasms having a replacement growth pattern. AJR Am J Roentgenol. 2003; 180:817–826. PMID: 12591704.


9. Kim EA, Johkoh T, Lee KS, Han J, Fujimoto K, Sadohara J, et al. Quantification of ground-glass opacity on high-resolution CT of small peripheral adenocarcinoma of the lung: pathologic and prognostic implications. AJR Am J Roentgenol. 2001; 177:1417–1422. PMID: 11717098.
10. Lee HJ, Goo JM, Lee CH, Yoo CG, Kim YT, Im JG. Nodular ground-glass opacities on thin-section CT: size change during follow-up and pathological results. Korean J Radiol. 2007; 8:22–31. PMID: 17277560.


11. Ko JP, Rusinek H, Jacobs EL, Babb JS, Betke M, McGuinness G, et al. Small pulmonary nodules; volume measurement at chest CT-phantom study. Radiology. 2003; 228:864–870. PMID: 12954901.


12. Markarian B, Dailey ET. Heizman ER, editor. Preparation of inflated lung specimens. The Lung : radiologic-pathologic correlations. 1984. 2nd ed. St Louis, MO: Mosby-Year Book;p. 4–12.
13. Vieira SR, Puybasset L, Lu Q, Richecoeur J, Cluzel P, Coriat P, et al. A scanographic assessment of pulmonary morphology in acute lung injury. Significance of the lower inflection point detected on the lung pressure-volume curve. Am J Respir Crit Care Med. 1999; 159:1612–1623. PMID: 10228135.
14. Lee HJ, Im JG, Goo JM, Kim YI, Lee MW, Ryu HG, et al. Acute lung injury: effects of prone positioning on cephalocaudal distribution of lung inflation CT assessment in dogs. Radiology. 2005; 234:151–161. PMID: 15550370.
15. Malbouisson LM, Muller JC, Constantin JM, Lu Q, Puybasset L, Rouby JJ. CT Scan ARDS Study Group. Computed tomography assessment of positive end-expiratory pressure-induced alveolar recruitment in patients with acute respiratory distress syndrome. Am J Respir Crit Care Med. 2001; 163:1444–1450. PMID: 11371416.
16. Marten K, Funke M, Engelke C. Flat panel detector-based volumetric CT: prototype evaluation with volumetry of small artificial nodules in a pulmonary phantom. J Thorac Imaging. 2004; 19:156–163. PMID: 15273611.
17. Do KH, Chung MJ, Goo JM, Lee KW, Im JG. Evaluation of computer aided volumetry for simulated small pulmonary nodules on computed tomography. J Korean Radiol Soc. 2004; 50:101–108.


18. Henschke CI, Naidich DP, Yankelevitz DF, McGuinness G, McCauley DI, Smith JP, et al. Early lung cancer action project: initial findings on repeat screening. Cancer. 2001; 92:153–159. PMID: 11443621.
19. Jennings SG, Winer-Muram HT, Traver RD, Farber MO. Lung tumor growth: assessment with CT comparison of diameter and cross-sectional area with volume measurement. Radiology. 2004; 231:866–871. PMID: 15163822.
20. Kostis WJ, Yankelevitz DF, Reeves AP, Fluture SC, Henschke CI. Small pulmonary nodules; reproducibility of three-dimensional volumetric measurement and estimation of time to follow-up CT. Radiology. 2004; 231:446–452. PMID: 15128989.


Fig. 1
Phantom nodules made of fixed swine lung (A). Forty nodules were placed in wells with diameter of approximately 10 mm of 5 cm-thick Styrofoam plate (B).
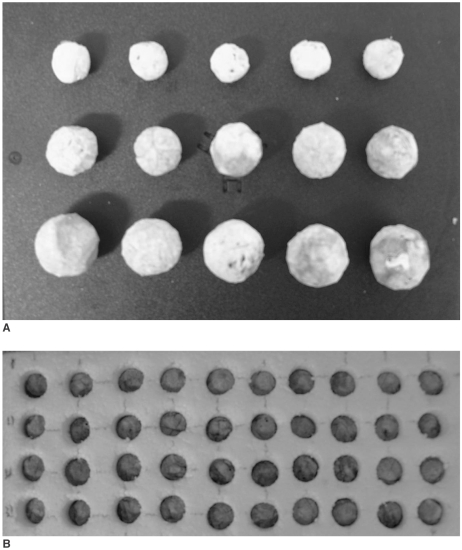
Fig. 2
CT image of 40 ground-glass opacity nodules. Mean attenuation values of nodules range from -754 HU to -352 HU.
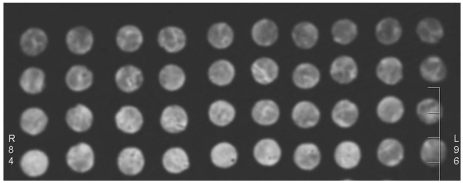
Fig. 3
Scatterplots of linear regression.
A. Regression of STARS on STACT of nodules at -900 HU lower threshold level. Scatterplot shows very strong correlation between STACT and STARS. Resulting linear equation was as follows: STARS = 1.036 × STACT + 21.199 (R2 = 0.996, p < 0.01).
B. Regression of STARS on VCT of nodules at -900 HU lower threshold level. Scatterplot shows poor correlation between VCT and STARS (R2 = 0.294, p < 0.01).
C. Regression of relative percent error of VCT on attenuation values of nodules at 900 HU lower threshold level. Scatterplot shows negative strong correlation between mean attenuation value of nodules and VCT (R2 = 0.941, p < 0.01).
RPE = relative percentage error; STACT = CT-measured amount of soft tissue in nodules; STARS = reference-standard amount of soft tissue in nodules; VCT = CT-measured volume of nodules; MHU = mean attenuation value of nodules
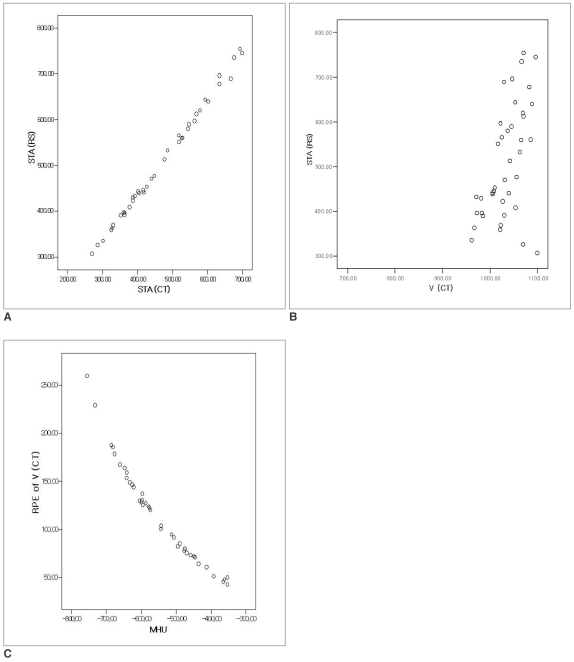