Abstract
Objective
The purpose of this study is to evaluate the effect of dexamethasone on the damaged blood-ocular barrier caused by triolein emulsion, using contrast-enhanced MR imaging.
Materials and Methods
An emulsion of 0.1-mL triolein in 20 mL of saline was infused into the carotid arteries of 32 cats, 12 cats were placed in the treatment group and 18 cats were placed in the Control group. Thirty minutes after the infusion of triolein emulsion, a set of orbital pre- and post-contrast T1-weighted MR images (T1WIs) were obtained. Infusion of 10 mg/kg dexamethasone into the ipsilateral carotid artery of each of the cats in the treatment group cats and 20 mL saline in each of the cats in the control group was given. A second set of pre- and post-contrast orbital T1WIs were obtained three hours following triolein emulsion infusion. Qualitative analysis was performed for the the anterior chamber (AC), the posterior chamber (PC), and in the vitreous humor of the ipsilateral and contralateral eyes. The signal intensity ratios of the ipsilateral eye over the contralateral eye were quantitatively evaluated in the three ocular chambers on the first and second set of T1WIs, and were then statistically compared.
Results
Qualitatively, the AC, the PC or the vitreous did not show immediate contrast enhancement on the first and the second set of post-contrast T1WIs. However, the AC and the PC showed delayed contrast enhancement for both groups of cats on the second pre-contrast T1WIs. No enhancement or minimally delayed enhancement was seen for the vitreous humor. Quantitatively, the signal intensity ratios in the PC of the treatment group of cats were statistically lower than the ratios of the control group of cats for the second set of T1WIs (p = 0.037). The AC and vitreous showed no statistically significant difference between the feline treatment group and control group (p > 0.05).
Both the blood-brain barrier breakdown and vasogenic edema induced by intra-carotid arterial infusion of a bolus of triolein or triolein emulsion can be seen on contrast-enhanced MR images (1-4). Triolein is a major fat of bone marrow and is therefore a main causative factor of a clinical fat embolism (5).
The blood-ocular barrier (BOB) consists of the blood-retinal barrier (BRB) and the blood-aqueous barrier (BAB). Both the BRB and the BAB inhibit the movement of substances from the plasma to the vitreous or aqueous humor (6, 7). These barriers therefore decrease the effect of chemotherapy (8). To the best of our knowledge, there has been no study attempting to open the BOB by intra-arterial infusion of fat or with a fat emulsion.
The BOB breakdown can be studied on contrast-enhanced MR images. When the BRB is disrupted, contrast media enters the vitreous space and increases the surrounding water proton relaxation rate in direct proportion to the concentration of gadolinium (9). The advantages of MR imaging for studying the integrity of the BOB include repeatability, objectivity, and non-invasiveness. Moreover, quantitative assessment of the BOB breakdown can be performed using contrast-enhanced MR images (9, 10).
Treatment of the clinical fat embolism syndrome is essentially supportive and consists of the use of cardiovascular and respiratory resuscitation and stabilization. Corticosteroids are clinically efficacious inhibitors of edema of the cerebrum, the ciliary body and the retina (10-12). Therefore, BOB breakdown induced by triolein emulsion resulting in increased vascular permeability could be decreased by the use of corticosteroids.
The purpose of this study is to determine the effect of triolein emulsion on the BOB, as determined by the analysis of contrast-enhanced MR images. If triolein emulsion disrupts the BOB, contrast enhancement will be demonstrated in the ocular chambers. The second purpose of the study is to evaluate the effect of dexamethasone on the disrupted BOB. If dexamethasone blocks the disruption of the BOB induced by triolein emulsion, contrast enhancement will decrease on the MR images.
The Animal Research Committee of our institution approved all of our experiments and surgical procedures. The cats were divided into two groups. In the dexamethasone treatment group (n = 12), the cats were injected with 10 mg/kg dexamethasone after infusion of the triolein emulsion. In the control group (n = 18), the cats were injected with 20 ml of normal saline after infusion of triolein emulsion.
The adult cats used in this study each weighed 2.7-3.8 kg. The cats were anesthetized by an intramuscular injection of ketamine HCl (2.5 mg/kg; Korea United Pharm Inc., Seoul, Korea) and xylazine (0.125 mg/kg; Bayer Korea, Seoul, Korea). The cats were allowed to breathe spontaneously ambient air during the procedure. An 18-gauge, intravenous catheter (Angiocath; Becton Dickinson, UT) was placed in the right femoral vein for the purpose of contrast infusion. The right femoral artery was isolated and its distal portion was ligated with 4.0-silk. A second, 18-gauge intravenous catheter was then inserted into the artery. A 3.0F microcatheter (MicroFerret-18 Infusion Catheter, William Cook Europe, Bjaeverskov, Denmark) with a microguidewire, was passed through the intravenous catheter into the lumen of the artery. Either the right or the left internal carotid artery was randomly selected. The tip of the microcatheter was positioned at the level of the fourth cervical vertebra.
A 1-ml syringe containing 0.05 ml of neutral triglyceride triolein (1,2,3-tri [cis-9-octadecenoyl]glycerol, 99% purity, d = 0.91 g/ml, Sigma, St. Louis, MO) and a 25-ml syringe containing 20 ml of saline, were connected to a three-way stopcock. The fat emulsion was made by mixing triolein and saline via the stopcock with a vigorous to-and-fro movement of the syringes for two minutes. The emulsified fat was manually infused into the selected carotid artery at a rate of 4 ml/min for five minutes, followed by infusion of 3 ml of saline.
All studies were performed on a 1.5-T MR unit (Sonata, Siemens, Erlangen, Germany). Each cat was placed in the prone position within a pediatric MR positioner, and a flexible coil was placed above the head of the animal. Orbital images were obtained sequentially 30 minutes after infusion of the triolein emulsion. Using the spin-echo sequence, pre- and post-contrast axial T1-weighted images (T1WIs) were obtained with the following parameters: repetition time (TR) = 320 ms; echo time (TE) = 20 ms; section thickness = 4 mm with a 0.1-mm gap; field of view = 70-75 mm; two excitations; and acquisition matrix = 210 × 256. For contrast studies, 0.2 mmol/kg gadopentate dimeglumine (Magnevist, Schering, Germany) was injected intravenously.
After the first MR imaging, selection of the ipsilateral carotid artery with the microcatheter was performed under fluoroscopic guidance. Dexamethasone (10 mg/kg) was then injected into the carotid artery through the catheter in the feline treatment group approximately one hour following the triolein emulsion infusion. In the feline control group, 10 ml of normal saline at room temperature was infused into the animals.
Using the same sequences as the images of the first MR sequences, pre- and post-contrast, orbital MR images were obtained in both groups of cats three hours after the infusion of triolein emulsion. For contrast studies, 0.2 mmol/kg gadopentate dimeglumine (Magnevist, Schering, Germany) was injected intravenously.
Two experienced neuroradiologists analyzed the MR images. The observers were blinded to the lesion site and group number. The signal intensities (SIs) of the anterior chamber (AC), the posterior chamber (PC), and the vitreous humor were analyzed by consensus in the pre- and post-contrast T1WIs obtained at 30 minutes and three hours after infusion of the triolein emulsion. The SI of the three-hour MR images was compared with the signal intensity of the 30-minute images.
The SIs of the AC, the PC, and the vitreous humor were measured on both sides on the MR scanner workstation (Sonata, Siemens, Erlangen, Germany). A circular region of interest (ROI) was selected at the center of the AC, the PC, and in the vitreous humor (Fig. 1). The dimension of the circular ROI was 0.05-0.09 cm2 in the AC, 0.01-0.02 cm2 in the PC, and 25-35 cm2 in the vitreous humor. The mean SI ratios (SI of the ipsilateral eye/SI of the contralateral eye) of the three chambers were calculated from the first and second pre- and post-contrast T1WIs that were obtained 30 minutes and three hours, respectively, after infusion of the emulsion. To evaluate the therapeutic effect of dexamethasone or saline on the disrupted BOB, the SI ratios of the second MR images were compared for the feline treatment and control groups. Statistical analysis was performed using the analysis of covariance. P < 0.05 was considered to be statistically significant.
First set of MR images obtained 30 minutes after the infusion of triolein emulsion.
All of the three chambers were hypointense on the pre-contrast T1WIs in both groups of cats (Fig. 1 A1 and B1). There was no remarkable difference in the hypointensity in any of the three chambers. The hypointensity showed no difference between the ipsilateral and the contralateral eyes. On post-contrast T1WIs, all three chambers were again hypointense, and there was no contrast enhancement (Fig. 1 A2 and B2) in any of the cats in either group.
The ipsilateral AC showed hyperintensity on the pre-contrast T1WIs in 11 of 12 cats in the treatment group and in all 18 cats in the control group. The contralateral AC also showed hyperintensity in 10 of 12 cats in the treatment group and in all 18 cats in the control group (Fig. 1 A3 and B3). The AC hyperintensity was more prominent in the ipsilateral eye than in the contralateral eye in the control group of cats (Fig. 1 B3, right eye).
The ipsilateral PC revealed hyperintensity on pre-contrast T1WIs in nine of 12 cats in the treatment group (Fig. 1 B3, right eye) and in all 18 cats in the control group, however, the PC of the feline treatment group was less hyperintense than that of the feline control group (Fig. 1 A3, left eye). The contralateral PC showed mild hyperintensity in six of 12 cats in the treatment group and in seven of 18 cats in the control group (Fig. 1 A3, right eye and B3, left eye).
In the majority of cats in both groups, the vitreous humor showed no hyperintensity on the pre-contrast T1WIs of either eye (Fig. 1 A3 and B3). However, in two control group cats, the vitreous humor showed minimal hyperintensity (Fig. 1 B3). On post-contrast T1WIs, no additional contrast enhancement was revealed in any of the three chambers in either eye in either group as compared with the pre-contrast T1WIs obtained three hours after triolein emulsion infusion (Fig. 1 A4 and B4).
The mean SI and SI ratios in the three chambers in both groups are shown in Tables 1 and 2, respectively. The statistical results are also described in Table 2.
Immediate contrast enhancement was not prominent in any acquisition. SIs increased in all three chambers in both eyes of both groups of animals on the second, three-hour T1WIs compared to the first, 30-minute T1WIs, even though the SIs in the vitreous humor increased only minimally. Compared to the other chambers at three hours, the AC revealed the most prominent SI increase in the ipsilateral eyes and in the contralateral eyes in both groups. The SI increase of the PC of the ipsilateral eyes in the treatment group of cats was lower than that in the control group of cats.
The mean SI ratios of the treatment group of cats were higher in the AC compared with those of the control group of cats. However, there was no statistically significant difference in the SI ratios in the AC between the feline treatment group and control group (p > 0.05). In the PC, the SI ratios of the treatment group of cats were significantly lower than the SI ratios of the control group of cats (p = 0.037). The mean SI ratios of the vitreous humor were lower than the mean SI ratios of the AC and the PC in both groups. There was no significant difference in the SI ratios of the vitreous humor between the feline treatment group and control group (p > 0.05).
In the present study, the three chambers - AC, PC, and the vitreous humor - showed different enhancement patterns on the post-contrast T1WIs. Manfre et al. (13) showed that immediate MR imaging after BOB damage did not show contrast enhancement; however, leakage of the contrast medium into the vitreous body or into the aqueous fluid, was demonstrated on delayed scanning obtained 40-50 min following contrast administration. In the present study, delayed contrast enhancement was also observed in the AC of the contralateral eyes in both feline groups (Fig. 1 A3 and B3). This contrast enhancement was due to the normal leakage of contrast medium through the fenestrated capillaries of the ciliary body stroma (14). This result supports our suspicion that proteins bypass the PC and enter the AC directly via the iris root (15). However, the delayed contrast enhancement was mild in the PC of the contralateral eyes.
The vitreous humor showed only mild or less significant enhancement in the normal contralateral eyes in both groups of animals. This finding demonstrates the relatively tight nature of the BRB. The BRB has tight junctions of the non-leaky type. The permeability of the BRB generally resembles cellular permeability, with diffusion being directly related to the predominant roles of lipid solubility and the transport mechanisms (6). In the present study, opening of the BRB was minimal or non-existent, while the BAB revealed significant breakdown.
The effect of triolein emulsion on the normal contralateral eyes seen in this study should be considered as small triolein droplets penetrating capillaries of the brain by continued perfusion pressure from the heart could circulate in the systemic blood stream. Therefore, the circulating triolein emulsion could affect the normal contralateral eyes. However, in previous studies (1-4), triolein did not remarkably affect the contralateral side, probably due to filtration of the triolein droplets by the pulmonary capillaries. As pulmonary arterial pressure is lower than cerebral arterial pressure, the triolein droplets are therefore easily able to penetrate the brain, but not the lung capillaries.
In the ipsilateral eyes on 30-minute, post-contrast T1WIs, no definite contrast enhancement was seen in the three chambers in either group of cats, qualitatively or quantitatively. This finding indicates that extravasation of the contrast media via the BOB, especially by the BAB, into the ocular chambers, is very slow even when the barriers are disrupted. The slow leakage was also evident on the three-hour, post-contrast T1WIs. Compared to the three-hour, pre-contrast T1WIs, no substantial additional contrast enhancement of the ipsilateral eyes was noted in either group of cats on the three-hour, post-contrast T1WIs.
The AC of the ipsilateral eyes in the feline control group showed the highest SI on the three-hour T1WIs. The PC of the same eyes was not as high as the AC. This difference was also noted for the feline treatment group. The SIs of the PC were lower than the SIs of the AC in the ipsilateral eyes of the treatment group animals on the three-hour T1WIs (Table 1). These results suggest that the origin of the aqueous humor of the PC is not the same as that of the AC, thereby supporting the supposition that protein diffusion into the AC does not involve the PC (15).
The present study supports the supposition that protein diffusion into the AC does not involve the PC, as the dexamethasone effect was positive in the PC in this study. The SI ratios were highest in the PC of the ipsilateral eyes in the control group of cats and were significantly lower in the PCs of the treatment group of cats (Table 2). There was no statistical difference in the ACs of the treatment and the control groups. These results suggest that the physiology of the secretion of the aqueous humor into the AC and the PC may be different. Recent fluorophotometric and tracerlocalization studies also indicate that plasma-derived protein in the aqueous humor of the normal rabbit (16) and monkey (17) eyes enters the AC directly from the ciliary body stroma through the iris root.
Patients with long-bone fractures are at risk for the developing fat embolism syndrome. Although an estimated 50% of patients with overt fat embolism syndrome have retinal abnormalities, the overall incidence of fundus lesions in patients with long-bone fractures has been reported as 4% (18). Subclinical fat embolism syndrome has been identified by ophthalmoscopy in patients with long-bone fractures (18). However, to the best of our knowledge, MR imaging findings of the clinical or experimental ocular fat embolism syndrome have not yet been reported.
Treatment of clinical fat embolism syndrome is essentially supportive, consisting of cardiovascular and respiratory resuscitation and stabilization. No specific drug therapy is currently recommended for fat embolism syndrome. Small, prospective, randomized, controlled studies of steroid prophylaxis in patients with long-bone fractures have indicated both a decrease in the development of fat embolism syndrome and a decreased incidence of hypoxemia in the steroid-treated groups of patients as compared with the results in control groups of patients (19). The action mechanism of corticosteroids seems to be a decrease in the rise of plasma-free, fatty-acid levels and an inhibition of the inflammatory cascade that occurs in the early stages of fat embolism syndrome development. However, the dose regimens in fat embolism syndrome have not been standardized and vary from six doses of 1.5 mg/kg methylprednisolone every eight hours (a total dose of 9 mg/kg) to two doses of 30 mg/kg (a total dose 60 mg/kg) (20, 21). In the present study, as only a single, intra-arterial infusion of 10 mg/kg dexamethasone was administered to the animals, the dosage was maintained at nearly the lowest level possible for the steroid treatment of clinical fat embolism syndrome. In the present study, dexamethasone significantly reduced contrast enhancement of the PC. Postulated mechanisms of steroid action hypothetically include membrane stabilization, limitation of the rise in plasma-free, fatty-acid levels, and inhibition of complement-mediated leukocyte aggregation (22).
Corticosteroid treatment prevents not only a BBB but also a BOB breakdown (23). In the present study, contrast enhancement of the PC was significantly lower in the steroid treatment group of cats than in the control group of animals (Table 2) (Figs. 1, 2). This finding indicated that the steroid prevents BAB breakdown. However, in the vitreous humor, the steroid effect was not evident (p > 0.05). This was probably because the vitreous humor showed minimal contrast enhancement in the embolized eyes of a few cats, and therefore in this study, there was no significant difference in the SI in the steroid treatment and control groups.
The small volume of the PC compared with that of the AC or the vitreous humor was a limitation in the present study as it was not a simple procedure to position the ROI in the PC. To accurately measure the SI in the PC, MR images were magnified in the workstation. The area of the ROI for quantitative analysis in the present study in the PC was 0.01~0.02 cm2. This area was adequate for SI measurement in the PC in the present study.
Clinical application of these results is not intended in the present study. However, infusion of triolein emulsion into the ophthalmic artery can be considered for hypovascular tumors of the eyeball in order to improve the therapeutic effect. In addition, steroid treatment can reduce potential orbital complications for the clinical fat embolism syndrome. Further studies will be needed to approach any clinical applications of the present study.
In conclusion, in our study, contrast-enhanced MR images revealed increased vascular permeability of the BAB in cats after intra-arterial infusion of triolein emulsion. Dexamethasone seems to decrease the breakdown of the BAB, especially in the PC.
Figures and Tables
![]() | Fig. 1Orbit MR images (TR/TE, 320/20) of cat in treatment group (A), and of cat in control group (B). Pre-contrast (A1 and B1) and post-contrast (A2 and B2) MR images obtained 30 minutes following triolein emulsion infusion. Pre-contrast (A3 and B3) and post-contrast (A4 and B4) MR images obtained three hours following triolein emulsion infusion. For quantitative analysis, signal intensity was measured with circular region of interest depicted in anterior and posterior chambers and in vitreous humor (A1). Post-contrast MR images obtained 30 minutes following triolein emulsion infusion show no contrast enhancement in anterior chamber, posterior chamber or in vitreous humor (A2 and B2). Pre-contrast MR images obtained three hours following triolein emulsion infusion reveal high signal intensity in anterior chambers of lesion and in contralateral eyes in cats in both groups (A3 and B3), thus representing delayed contrast enhancement. However, hyperintensity is most prominent in ipsilateral eyes of control group cat (B3). Posterior chamber of ipsilateral eyeballs of control group cat show strong, delayed contrast enhancement (long arrow in B3). However, in treatment group cat, posterior chamber of ipsilateral eyeball reveals mild contrast enhancement (long arrow in A3). Minimal, delayed contrast enhancement is seen in posterior vitreous humor of ipsilateral eyes of control group cat (short arrows in B3 and B4). |
Table 1
Mean Signal Intensity in Three Chambers of Ipsilateral and Contralateral Eyes in Both Treatment Groups of Cats at 30 Minutes and Three Hours following Triolein Emulsion Infusion
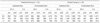
Acknowledgment
We are grateful to Bonnie Hami, Department of Radiology, University Hospital of Cleveland and Haaga Radiology Research, for her assistance in the preparation of this manuscript.
References
1. Kim HJ, Lee CH, Kim HG, Lee SD, Son SM, Kim YW, et al. Reversible MR changes in the cat brain after cerebral fat embolism induced by triolein emulsion. AJNR Am J Neuroradiol. 2004. 25:958–963.
2. Kim HJ, Lee CH, Lee SH, Moon TY. Magnetic resonance imaging and histologic findings of experimental cerebral fat embolism. Invest Radiol. 2003. 38:625–634.
3. Kim HJ, Lee CH, Lee SH, Cho BM, Kim HK, Park BR, et al. Early development of vasogenic edema in experimental cerebral fat embolism in cats: correlation with MRI and electron microscopic findings. Invest Radiol. 2001. 36:460–469.
4. Kim HJ, Lee JH, Lee CH, Lee SH, Moon TY, Cho BM, et al. Experimental cerebral fat embolism: embolic effects of triolein and oleic acid depicted by MR imaging and electron microscopy. AJNR Am J Neuroradiol. 2002. 23:1516–1523.
5. Boyd HM, Peltier LF, Scott JR, Wheeler DH. Fat embolism. II. The chemical composition of fat obtained from human long bones and subcutaneous tissue. Surgery. 1956. 40:661–664.
6. Cunha-Vaz J. The blood-ocular barriers. Surv Ophthalmol. 1979. 23:279–296.
7. Pederson JE, Green K. Aqueous humor dynamics: experimental studies. Exp Eye Res. 1973. 15:277–297.
8. Verbraeken H, Verstraete A, Van de Velde E, Verschraegen G. Penetration of gentamicin and ofloxacin in human vitreous after systemic administration. Graefes Arch Clin Exp Ophthalmol. 1996. 234:Suppl 1. S59–S65.
9. Berkowitz BA, Tofts PS, Sen HA, Ando N, de Juan E Jr. Accurate and precise measurement of blood-retinal barrier breakdown using dynamic Gd-DTPA MRI. Invest Ophthalmol Vis Sci. 1992. 33:3500–3506.
10. Kolodny NH, Goode ST, Ryan W, Freddo TF. Evaluation of therapeutic effectiveness using MR imaging in a rabbit model of anterior uveitis. Exp Eye Res. 2002. 74:483–491.
11. Severinghaus JW. Hypothetical roles of angiogenesis, osmotic swelling, and ischemia in high-altitude cerebral edema. J Appl Physiol. 1995. 79:375–379.
12. Martidis A, Duker JS, Greenberg PB, Rogers AH, Puliafito CA, Reichel E, et al. Intravitreal triamcinolone for refractory diabetic macular edema. Ophthalmology. 2002. 109:920–927.
13. Manfre L, Midiri M, Giuffre G, Mangiameli A, Cardella G, Ponte F, et al. Blood-ocular barrier damage: use of contrast-enhanced MRI. Eur Radiol. 1997. 7:110–114.
14. Freddo TF, Patz S, Arshanskiy Y. Pilocarpine's effects on the blood-aqueous barrier of the human eye as assessed by high-resolution, contrast magnetic resonance imaging. Exp Eye Res. 2006. 82:458–464.
15. Kolodny NH, Freddo TF, Lawrence BA, Suarez C, Bartels SP. Contrast-enhanced magnetic resonance imaging confirmation of an anterior protein pathway in normal rabbit eyes. Invest Ophthalmol Vis Sci. 1996. 37:1602–1607.
16. Freddo TF, Bartels SP, Barsotti MF, Kamm RD. The source of proteins in the aqueous humor of the normal rabbit. Invest Ophthalmol Vis Sci. 1990. 31:125–137.
17. Barsotti MF, Bartels SP, Freddo TF, Kamm RD. The source of protein in the aqueous humor of the normal monkey eye. Invest Ophthalmol Vis Sci. 1992. 33:581–595.
18. Chuang EL, Miller FS 3rd, Kalina RE. Retinal lesions following long bone fractures. Ophthalmology. 1985. 92:370–374.
19. Jenkins K, Chung F, Wennberg R, Etchells EE, Davey R. Fat embolism syndrome and elective knee arthroplasty. Can J Anaesth. 2002. 49:19–24.
20. Kallenbach J, Lewis M, Zaltzman M, Feldman C, Orford A, Zwi S. 'Low-dose' corticosteroid prophylaxis against fat embolism. J Trauma. 1987. 27:1173–1176.
21. Lindeque BG, Schoeman HS, Dommisse GF, Boeyens MC, Vlok AL. Fat embolism and the fat embolism syndrome. A double-blind therapeutic study. J Bone Joint Surg Br. 1987. 69:128–131.
22. Hammerschmidt DE, Weaver LJ, Hudson LD, Craddock PR, Jacob HS. Association of complement activation and elevated plasma-C5a with adult respiratory distress syndrome. Pathophysiological relevance and possible prognostic value. Lancet. 1980. 1:947–949.
23. Edelman JL, Lutz D, Castro MR. Corticosteroids inhibit VEGF-induced vascular leakage in a rabbit model of blood-retinal and blood-aqueous barrier breakdown. Exp Eye Res. 2005. 80:249–258.